Clinical Vignette
Background
As described in the previous chapter, terrorists could use nuclear material in one of a half dozen scenarios: detonation of a nuclear weapon, detonation of an IND, sabotage of a nuclear power facility, or more probably, RPSs or RDDs. This chapter summarizes the known health effects from exposure to nuclear materials.
Properties
Although neither the detonation of a thermonuclear device, an atomic bomb, nor an attack on a nuclear reactor would go unnoticed, the use of RPSs and RDDs may not readily be detected as radioactivity and cannot be detected by our senses. Exposure from an RPS or from an RDD would only be known once symptoms of radiation-related illnesses began or by detection using sensing devices such as a Geiger counter.
Minimizing the health effects of radiation exposure is consequence of three factors: time, distance, and barrier. There is a dose-dependent relationship for the time of exposure and the amount of radiation absorbed that directly impacts health effects. Similarly, exposure decreases exponentially and proportionally to the distance from a radiation source. Physical barriers, even if not leaded, provide an effective shield. The denser the shielding, of course, the more protection afforded.
Sources
Radiation exposure comes from a wide range of natural and manmade sources. Most naturally occurring atoms (e.g., thorium, uranium, radium) that exist in the earth exist at thermodynamically low-energy levels and are quite stable. The few that are unstable, (e.g., uranium) attempt to become stable by releasing energy from their nuclei in the form of radiation. There are internal body deposits of radiation in living cells, including potassium-40, carbon-14, radium, and others. External cosmic rays originate in outer space and exposure to them increase twofold in mountainous regions, and even more at jet aircraft altitudes. Human exposure to natural radiation is greater in that area of the earth’s crust rich in radioactive elements. X-rays from medical diagnostics and treatment are the largest source of artificial ionizing radiation exposure. Additional exposures occur from cigarettes, radioactive material in building materials or bedrock, radon gas, and phosphate fertilizers. Production, regulatory, and construction workers in the nuclear industry are exposed, although stringent monitoring requirements are in place. Medical, emergency response, and first-responder personnel may inadvertently be exposed to radiation before they are aware that a nuclear terror event has transpired.
Pathophysiology
Radiation injuries result from three basic methods of exposure: irradiation, external contamination, and internal contamination. The location and severity of injury are influenced by these routes of exposure. Irradiation is radiation exposure from an external source without physical contact with the body. Irradiation from x-rays, gamma rays, neutrons, or alpha or beta particles may be non-penetrating (affecting only the skin) or penetrating (affecting areas deeper than the skin). The depth of penetration is a function of the energy contained within the electromagnetic wave or radiation particle. Decontamination is unnecessary with irradiation so HCWs may focus their attention on acute medical issues. External contamination occurs when radionuclides come into contact with external body surfaces. Adequate decontamination is needed with external contamination to avoid exposure to care providers. These particles are transferable to hands, clothing, and other items, and can inadvertently add to the patient’s body burden if allowed to be inhaled, ingested, or entered into open wounds. Finally, internal contamination results from the inhalation, ingestion, injection, or intrusion through wounds of radionuclides. Once inside the body, they emit damaging radiation to local tissues and organs.
Three properties govern how tissues respond to radiation. First, radiation sensitivity varies directly with the rate of cell proliferation and with the number of potential future cell divisions. Simply put, rapidly growing cells (e.g., gastrointestinal epithelium) or cell lines that are generative (e.g., spermatogonia or hematopoeitic stem cells) are more sensitive to radiation than are other cells. Radiosensitivity varies inversely with the degree of morphologic and functional differentiation; that is, more mature and differentiated cells are generally less susceptible to radiation injury than are immature or dedifferentiated cells.
Ionizing radiation (Fig. 30–1) breaks the covalent bonds of nuclear material and alters genomic sequences, irreversibly destroys the structure and function of formed proteins in tissues, and generates free radicals from water that may damage tissue. Linear energy transfer (LET), which determines the risk of injury, occurs maximally at a distance equal to the width of a DNA molecule, an inspiring fact of nature that explains in part how nature’s genetic abundance, evolution, and ionizing radiation are intertwined. Damage to any key cellular compounds with important structural or metabolic functions can result in cell damage or death. The extent of the damage determines whether the consequences occur immediately or with delay. DNA may experience hundreds of damaged sites after irradiation exposure. Types of damage that can occur vary from point mutations, partial and complete gene deletions, misrepair, to translocations. Ionizing radiation may cause sufficient DNA damage as to fracture the double helix itself. Indirect damage occurs via water molecules that have been ionized into free radical form or electrophilic hydroxyl ions.
Certain cell types and sites are classically affected by radiation exposure. The most radiosensitive cells are those that are most actively dividing or dedifferentiated, or stem cells, the latter due to the fact that they undergo repeated cell division. Relative biologic effectiveness (RBE) is a term used to describe the quality of injury caused by LET in cells. Those cells that have the most need of frequent sublethal repair turn out to be most radiosensitive. These include stem cells and gastrointestinal tract epithelium. Radiation may disrupt normal cell growth and replication, impair cell motility, and damage membranes. These effects may occur below the threshold for cellular apoptosis in cell death.
The high rate of cell turnover and large surface area makes the gastrointestinal tract among the most radiosensitive organs—with changes occurring within hours of exposure. Ingested radionuclides are absorbed or pass through, depending on physicochemical properties, such as solubility. Whatever the biological path of radioisotopes, they emit radiation into surrounding structures, tissues, cells, and cell organelles. The gut immune system components can also be affected, particularly intramucosal lymphocytes.
Stem cells within the hematopoetic system are also highly radiation sensitive, including the multipotent stems cells in the erythropoietic, thrombopoietic, and myelopoietic cell lines. There is a group of stem cells that, because of their lower frequency of dividing, tend to show greater survival with exposures of less than 6 Gy, and thereby play an essential role in restoration of cell lines. Lymphocytes are said to be the most vulnerable to radiation injury of all the blood elements.
Nervous tissue, being so well differentiated, can be damaged if exposed to high enough levels of radiation. Axonal demyelination, microvascular injury and hemorrhage, and apoptosis may all occur, resulting clinically in sensorimotor and autonomic dysregulation.
Pulmonary injury may occur if radionuclide particles are inhaled. Their effects depend on deposition site, which in turn is a function of particle size. Particles less than 5 microns reach the alveoli, whereas larger ones deposit higher up the respiratory tree where they cause local tissue, or if cleared by mucociliary escalator, find their way to the gastrointestinal tract.
Signs and Symptoms
ARS refers to the multiorgan system injury resulting from exposure to ionizing radiation. It requires a high dose of penetrating radiation (greater than 1 Gy) to all or much of the body (referred to as “whole body exposure”) over the course of several minutes to develop. ARS involves four organ systems: hematopoietic, gastrointestinal, pulmonary, and central nervous system (CNS). The severity of ARS depends on a combination of factors relating to exposure, such as duration and dose of radiation, how deep the radiation penetrates, and total amount of tissue exposed to radiation. Host factors, such as age and co-morbidities and are also pertinent.
The clinical presentation of ARS is independent of the type and the source of ionizing radiation. The Atomic Age is rife with examples where individuals developed ARS from therapeutic, industrial, accidental, or deliberate exposure to radiation.
ARS occurs as four clinically distinct stages: prodromal, latent, illness, and resolution. The severity of signs and symptoms worsens, and the time of each stage lessens as radiation exposure increases.
- 1. Prodrome. This stage occurs within minutes to hours after exposure, depending on the severity of the exposure. It is characterized by several days of nausea, vomiting (possibly bloody), diarrhea, malaise, and anorexia. Higher exposures (>5 Gy) may prolong and broaden the symptoms seen in the prodromal stage, including dypsnea, fever, fatigue, erythema, conjunctivitis, and irritability. The ARS prodrome typically resolves in several days.
- 2. Latency. This is a 2- to 6-week period during which the individual may feel relatively well or even completely asymptomatic. However, subclinical damage to stem cells, epithelial cells, and hematopoetic cell lines are underway, accompanied by functional immunodeficiencies and risk of opportunistic infections. The duration of the latent phase is inversely related to the severity of the exposure and may not occur at all with severe exposure.
- 3. Illness. A wide range of signs and symptoms can arise, including those seen during the prodromal phase, as well as bleeding, bruising, and fevers. Secondary clinical effects—immunodeficiency, sepsis, and thrombocytopenia—may develop. The sloughing of injured gastrointestinal epithelial cells and luminal stem cells causes malabsorption, hemorrhage, ulceration, and hypersecretion of fluid into the lumen. Dramatic fluid losses, hypotension, paralytic ileus, and microbial invasion may result in shock and infection. With severe radiation exposures (greater than 30 Gy), the CNS suffers diffuse microvascular damage, causing vomiting, mental status changes, fever, seizures, increased intracranical pressure, coma, and death. It follows that mortality is high and occurs quickly following the onset of CNS symptoms, typically within seventy-two hours.
- 4. Resolution. This stage results either in death or in clinical resolution of the ARS. Recovery may take months, but residual risk for malignancy remains.
Generally, the prognosis in ARS is proportional to the severity and duration of signs and symptoms. Using the aspects of concept of “biodosimetry,” signs and symptoms can help give an indication of exposure levels (Table 30–1). Whole body radiation below 2 Gy will resolve with little more than transient nausea and minimal or no extreme permanent injury, excepting the potential for carcinogenic transformation. Exposure to between 2 and 8 Gy will produce the ARS prodrome, but survival is still likely. In part this is due to the heterogeneity of exposure (e.g., due to partial shielding), so that some stem cells and other radiosensitive cells escape exposure and are then capable of aiding recovery once the acute, often infectious, risks pass. Whole body radiation doses higher than 8 Gy are almost uniformly fatal, with severe prodromal symptoms, CNS involvement, and rapid death.
Mild | Moderate | Severe | Lethal | |
---|---|---|---|---|
Signs and Symptoms | (1–2 Gy) | (2–4 Gy) | (4–6 Gy) | (>8 Gy) |
Vomiting | ||||
Onset | ≥2 hr | 1–2 hr | <1 hr | <10 min |
Incidence (%) | 10–50 | 70–90 | 100 | 100 |
Diarrhea | None | None | Mild | Heavy |
Onset | — | — | 3–8 hr | <1 hr |
Incidence (%) | — | — | <10 | ∼100 |
Temperature | Normal | <38.5°C | Fever | High fever |
Onset | — | 1–3 hr | ≥38.5°C | <1 hr |
Incidence (%) | — | 10–80 | 1–2 hr | 100 |
80–100 | ||||
Headache | Slight | Mild | Moderate | Severe |
Onset | — | — | 4–24 hr | 1–2 hr |
Incidence (%) | — | — | 50 | 80–90 |
Level of consciousness | Normal | Normal | Normal | Unconscious for sec-min |
Onset | — | — | — | Sec-min |
Incidence (%) | — | — | — | 100 at ≥50 Gy |
The absolute lymphocyte count is a useful predictor of mortality. A >50% decline in absolute lymphocyte count over twenty-four hours indicates substantial radiation exposure and risk of injury or death.
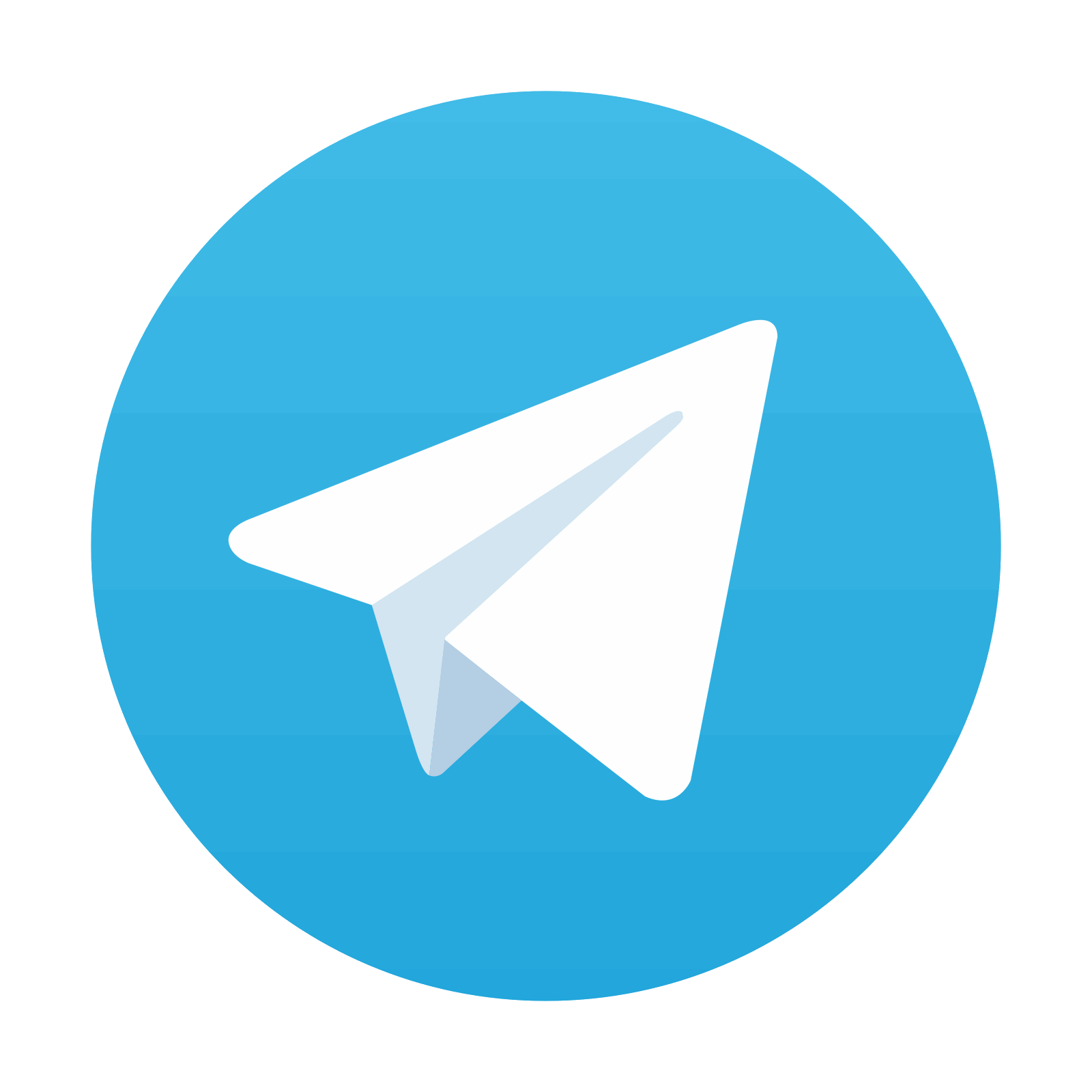
Stay updated, free articles. Join our Telegram channel

Full access? Get Clinical Tree
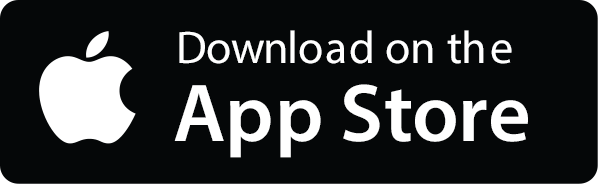
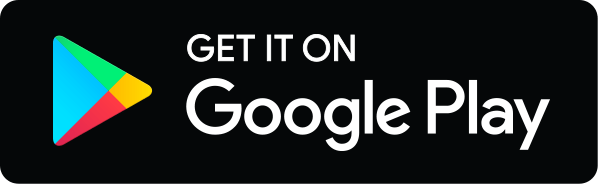