Abstract
Many of the drugs used by anaesthetists have effects on the medullary respiratory centre, the peripheral chemoreceptors and the airways. Their effects are most easily classified by drug class.
What effects do anaesthetic drugs have on the respiratory system?
Many of the drugs used by anaesthetists have effects on the medullary respiratory centre, the peripheral chemoreceptors and the airways. Their effects are most easily classified by drug class:
Volatile anaesthetic agents. The inhalational agents have dose-dependent effects on the respiratory system:
– An increase in respiratory rate (RR), but with reduced VT.
– Blunted ventilatory response to hypercapnoea, the extent of which varies between volatile agents: enflurane > desflurane > isoflurane > sevoflurane > halothane. The exceptions are N2O (which has no effect) and ether (which may increase V̇E).
Intravenous anaesthetic agents.
– Initial respiratory stimulation following induction of general anaesthesia.
– Subsequent respiratory depression. Respiratory stimulation is followed by abrupt respiratory centre depression. VT falls and apnoea may occur, especially with the use of propofol.
– Specific to certain drugs:
▪ Propofol depresses laryngeal reflexes. This is used to the anaesthetist’s advantage: depression of laryngeal reflexes allows the insertion of a laryngeal mask airway (LMA).
▪ Propofol is thought to abolish the peripheral chemoreceptor response to hypoxaemia.
▪ Ketamine differs from the other intravenous agents – in addition to its cardiovascular stability, ketamine preserves airway reflexes and spontaneous ventilation.
Other drugs commonly used in anaesthesia with effects on the respiratory system are:
– Depression of the respiratory centre, resulting in a reduced RR and blunting of the ventilatory response to hypercapnoea.
– Antitussive action, depressing the cough reflex.
– Histamine release (with morphine), which may precipitate bronchospasm in susceptible patients.
– Rarely, chest wall rigidity (wooden chest syndrome) following the rapid intravenous injection of strong opioids, which can interfere with ventilation.
Benzodiazepines cause depression of the respiratory centre, resulting in a decrease in RR and blunting of the ventilatory response to hypercapnoea.
Depolarising and non-depolarising muscle relaxants. The most obvious effect of the muscle relaxants on the respiratory system is respiratory muscle paralysis necessitating mechanical ventilation. Adequate reversal of neuromuscular blockade is required to prevent post-operative respiratory failure. Other respiratory effects specific to particular agents are:
– Atracurium may cause histamine release, resulting in bronchospasm.
– Suxamethonium and mivacurium may cause prolonged respiratory muscle weakness in patients with reduced levels of plasma cholinesterase (suxamethonium apnoea).
Non-steroidal anti-inflammatory drugs may precipitate bronchospasm in susceptible asthmatics.
Local anaesthetics used in neuraxial blockade. During spinal anaesthesia, intrathecal local anaesthetic may rise high enough to cause weakness of the intercostal muscles. This occurs most commonly in obstetric anaesthesia, where sensory levels of T2 to T4 are typical. The diaphragm is unaffected (as it is supplied by nerve roots C3–5), unless the level of block is exceptionally high.
How are the lungs affected by general anaesthesia?
There are many physiological changes during the induction and maintenance of anaesthesia:
– Laryngospasm and bronchospasm. Manipulation of the airway (by laryngoscopy, intubation or insertion of an LMA) can precipitate laryngospasm or bronchospasm. Bronchospasm is more common in patients who already have reactive airways (asthmatics, smokers), whilst laryngospasm is more common in children, particularly with inhalational inductions (as propofol is a useful depressant of laryngeal reflexes).
– Humidification. Endotracheal tubes (ETTs) and LMAs both bypass the upper airway. The typical functions of the upper airway (humidification, filtration and warming of inspired gases) are usually performed instead by a heat and moisture exchanger. Inadequate warming and humidification may result in dry, tenacious secretions, leading to mucous plugging.
– Loss of physiological positive end-expiratory pressure (PEEP). ETTs also bypass the larynx: the 3–5 cmH2O of physiological PEEP is lost, predisposing the patient to atelectasis.
– Increased work of breathing. The extent to which airway devices increase the resistance to gas flow depends on their internal radius, as determined by the Hagen–Poiseuille equation. The larger radius of the LMA makes it suitable for spontaneous ventilation. The narrower radius of the ETT increases the work of breathing. This is of particular importance in small children (very small ETT radius) and when weaning intensive care patients.
– Increased dead space. All airway devices increase mechanical dead space: V̇E must increase (sometimes significantly) if V̇A is to be maintained.
Lung volumes. At induction of general anaesthesia, functional residual capacity (FRC; the essential store of O2 during apnoea) is inevitably reduced due to:
– The supine position;
– The relaxation of the chest wall muscles associated with general anaesthesia;
– Co-morbidities, such as acute abdomen, obesity;
– Patient population, such as paediatric and obstetric patients.
To maximise FRC at induction of anaesthesia and therefore to maximise the volume of O2 in the lungs, it may help if the patient is in a semi-recumbent position. Despite adequate pre-oxygenation, some patients are particularly prone to hypoxaemia on induction of anaesthesia, such as the obese. Intraoperative positioning (lithotomy and Trendelenburg) further reduce FRC by increasing the pressure of the abdominal contents on the diaphragm.
Low lung volumes also cause a reduction in airway radius (which increases resistance to gas flow, leading to an increased work of breathing) and an increase in pulmonary vascular resistance.
Atelectasis has three causes: absorption of gases behind closed airways, alveolar compression and loss of pulmonary surfactant. The first two are common during general anaesthesia:
– Absorption atelectasis. When breathing room air, very little N2 diffuses across the alveolar–capillary barrier: N2 remains in the alveoli, ‘splinting’ them open. During anaesthesia, the combination of diffusible gases (O2 and N2O) and small-airway closure (due to CC encroaching on the reduced FRC) means that all the alveolar contents may diffuse into the blood, leading to atelectasis.
– Compression atelectasis. Under general anaesthesia, a combination of reduced diaphragmatic tone and compression from the abdominal contents causes basal atelectasis. This is exacerbated by the loss of physiological PEEP and increased abdominal pressure (e.g. due to pneumoperitoneum).
V̇/Q̇ mismatch as a result of:
– Low lung volume, causing closure of small airways (due to CC encroaching on FRC).
– Increased tendency for atelectasis.
– Positive pressure ventilation, which alters intrathoracic pressure, reducing right ventricular preload and changing pulmonary capillary dynamics; West zone 1 occurs in the lung apex (see Chapter 16).
– Impairment of hypoxic pulmonary vasoconstriction by volatile anaesthetic agents.
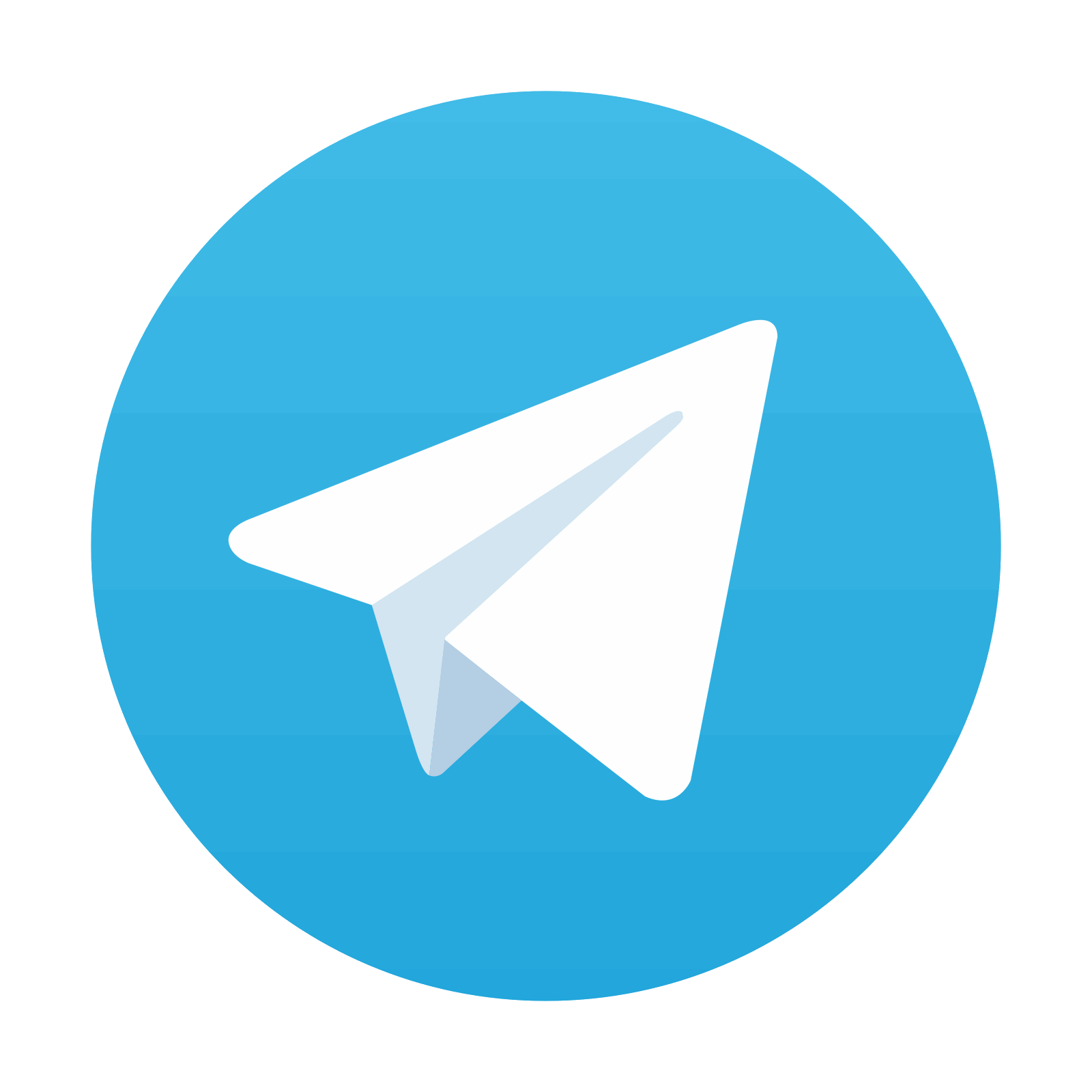
Stay updated, free articles. Join our Telegram channel

Full access? Get Clinical Tree
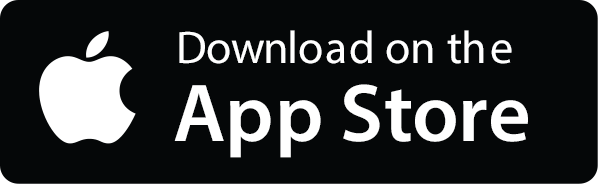
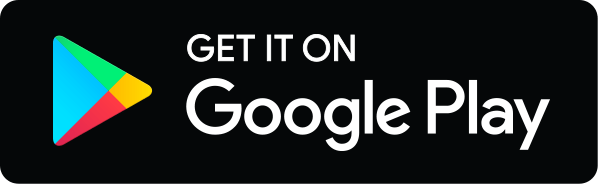