Summary
Pediatric anesthesiology is a distinct subspecialty of anesthesia. It focuses on the perioperative care of preterm and term neonates, infants, children, and teenagers. The pediatric population is vastly different from adults, as the clinician must recognize variations in anatomy, physiology, pharmacodynamics, and pharmacokinetics. For example, infants and children under 3 years old have a greater rate of morbidity and mortality associated with anesthesia than adults [1, 2]. The American Society of Anesthesiologists Closed Claims Project reports almost half of malpractice litigation involving pediatric injuries have been related to adverse respiratory events associated with improper oxygenation and/or ventilation. It is important for anesthesiologists caring for children to have a detailed knowledge of the distinctions between pediatric and adult patients. This chapter will discuss anatomic, physiologic, and pharmacologic distinctions, as well as infection prevention and anesthetic considerations, in the pediatric population.
Introduction
Pediatric anesthesiology is a distinct subspecialty of anesthesia. It focuses on the perioperative care of preterm and term neonates, infants, children, and teenagers. The pediatric population is vastly different from adults, as the clinician must recognize variations in anatomy, physiology, pharmacodynamics, and pharmacokinetics. For example, infants and children under 3 years old have a greater rate of morbidity and mortality associated with anesthesia than adults [Reference Tay, Tan and Ng1, Reference Kaye, Fox and Padnos2]. The American Society of Anesthesiologists Closed Claims Project reports almost half of malpractice litigation involving pediatric injuries have been related to adverse respiratory events associated with improper oxygenation and/or ventilation. It is important for anesthesiologists caring for children to have a detailed knowledge of the distinctions between pediatric and adult patients. This chapter will discuss anatomic, physiologic, and pharmacologic distinctions, as well as infection prevention and anesthetic considerations, in the pediatric population.
Anatomy
Head and Neck
When compared to adults, neonates and infants have a comparatively big head and tongue, but a relatively small body, necessitating a particular neutral shoulder roll for anesthesia induction [Reference Höhne, Haack, Machotta and Kaisers3, Reference Dalal, Murray, Messner, Feng, McAllister and Molter4]. The “sniffing position” is less advantageous for bag–mask ventilation or glottic visualization because of an infant’s relatively large head. Neonates have a more cephalad and anterior larynx, situated at the C3–4 vertebrae, rather than at the C6 vertebral level in adults [Reference Kaye, Fox and Padnos2, Reference Höhne, Haack, Machotta and Kaisers3]. The epiglottis is narrow, omega-shaped, and angled away from the axis of the trachea, thus obscuring the vocal cords [Reference Kaye, Fox and Padnos2]. Whereas an adult’s larynx is cylindrical in nature, a child’s larynx is funnel-shaped [Reference Butterworth, Mackey and Wasnick5]. Additionally, the cricoid cartilage is the narrowest portion of the airway in children aged 5 years or younger; the glottis is the narrowest section of the airway in older children and adults [Reference Butterworth, Mackey and Wasnick5]. The cricoid cartilage reaches adult proportions at the age of 10–12 years. Neonates are obligate nasal breathers until the age of 3–5 months. Prior to this age, the delicate nasal passages can be obstructed or compromised by secretions, nasal congestion, stenosis, canal atresia, endotracheal tubing, or even the tongue [Reference Trabalon and Schaal6].
Physiology
Respiratory
Oxygen consumption in a full-term neonate is twice that of an adult. In preterm neonates, oxygen consumption is three times an adult’s oxygen consumption. In comparison to adult patients, infants have the most resistance to airflow in small airways and the bronchi. The relatively smaller diameter of the neonatal airway and greater compliance of the trachea, bronchi, and chest wall provide less support to maintain negative intrathoracic pressure. Combined with low lung compliance (stiff lungs), increased chest wall compliance results in diminished functional residual capacity (FRC). Neonates and infants are more susceptible to hypoxia and respiratory failure because of a lower proportion of type 1 muscle fibers in the diaphragm and other respiratory muscles [Reference Kaye, Fox and Padnos2, Reference Butterworth, Mackey and Wasnick5]. Type 1 fibers allow prolonged, repetitive movement and the percentage of these muscle fibers increases with age. Therefore, preterm neonates have significantly fewer type 1 fibers, compared to even a 2-year-old child.
Loss of tone in pharyngeal and laryngeal structures is responsible for airway obstruction during sedation and anesthesia. This occurs in a dose-dependent fashion. The greater the depth of sedation, the greater the loss of airway muscle tone. Continuous positive airway pressure (CPAP), chin lift, and jaw thrust, as well as lateral positioning, improve airway patency and ventilation.
Cardiovascular
Newborns have a different cardiovascular physiology than older children and adults. Throughout growth, the cardiovascular system undergoes constant shifts in preload, afterload, contractility, loading, and flow patterns [Reference Vrancken, van Heijst and de Boode7]. There are characteristic differences in cytoarchitecture, metabolism, and function between the immature neonatal myocardium and the adult myocardium. For example, the neonatal myocardium has poorly formed T tubules and fewer mitochondria and sarcoplasmic reticulum, and depends on extracellular calcium for contractility. Carbohydrates and lactate serve as its primary energy source. However, the neonatal myocardium is parasympathetic-dominant, and has limited cardiac output increase with increased preload, as well as decreased compliance. Therefore, contractility cannot be enhanced to improve stroke volume with increased fluid volume. As a result, blood pressure is increased by increasing the heart rate [Reference Miller and Pardo8]. Anesthetic overdose can quickly result in bradycardia, which decreases cardiac activity. The neonatal heart is more vulnerable to unpredictable anesthetics and narcotics than the adult heart [Reference Kaye, Fox and Padnos2]; however, the neonatal myocardium can tolerate ischemia much better than the adult myocardium. Of note, cardiovascular events, such as bradycardia and asystole, frequently occur following inadequate oxygenation and ventilation. Thus, the origin of cardiovascular events seems to be secondary to respiratory complications.
Renal
Renal function is immature at birth and approaches adult values by the age of 2 years [Reference Bueva and Guignard9, Reference Rodieux, Wilbaux, van den Anker and Pfister10]. At birth, the newborn is in a condition of hypofiltration, with 20% of an adult’s glomerular filtration rate [Reference Musso, Ghezzi and Ferraris11, Reference Sulemanji and Vakili12]. Renal blood flow doubles in the first 2 weeks after birth and will reach adult values by the age of 2 years. As a result, the glomerular filtration rate parallels renal blood flow, and also doubles in the first 2 weeks following birth and increases until it reaches adult values, occurring at the age of between 1 and 2 years [Reference Kaye, Fox and Padnos2, Reference Sulemanji and Vakili12].
Fluid and volume management is an important task by the anesthesiologist because the neonatal kidney cannot conserve or excrete water in the same manner compared to older children and adults. Neonates are less efficient at excreting potassium and tend to have a higher normal serum value of potassium than adults. Neonates are also limited in their ability to respond to an acid load and tend to maintain a slightly acidic pH (7.37) and decreased plasma bicarbonate concentration, compared to older children and adults.
Kidney function impairment throughout adolescence has an impact on opioid clearance, intake, delivery, and metabolism [Reference Rodieux, Wilbaux, van den Anker and Pfister10].
Hepatic
A major function of the liver is to transform lipid-soluble drugs into water-soluble metabolites that are excreted by the kidney. At birth, the liver is structurally and physiologically immature. Hepatic blood supply is decreased in neonates and gradually improves as heart activity increases [Reference Rodieux, Wilbaux, van den Anker and Pfister10]. Drug-metabolizing enzymes, delivery mechanisms, and protein-binding systems are also impaired [Reference Kaye, Fox and Padnos2, Reference Rodieux, Wilbaux, van den Anker and Pfister10]. At birth, cytochrome P450 enzyme levels are 30% of adult levels [Reference Doherty and Salik13]. Cytochrome P450 enzymes are responsible for drug biotransformation. In neonates, phase I behavior is decreased but eventually increases to adult levels by late puberty [Reference Hines14]. Due to physiologic immaturity of neonatal hepatic metabolism, medications administered to neonates remain metabolically active for extended amounts of time, raising their susceptibility to general anesthetics, barbiturates, and opioids [Reference Kaye, Fox and Padnos2]. Furthermore, impaired liver function raises the likelihood of hypoglycemia, hyperbilirubinemia, and coagulopathy [Reference Doherty and Salik13].
Hematologic
Fetal hemoglobin (HbF) accounts for 70–90% of hemoglobin molecules in newborns, but is replaced by adult hemoglobin (HbA) at the age of 3 months [Reference Doherty and Salik13]. Thus, physiologic anemia is observed in preterm infants at 3–6 postnatal weeks and in term infants aged 8–12 weeks. HbF has a greater affinity for oxygen, which shifts the oxygen–hemoglobin dissociation curve to the left, thus shielding red blood cells from sickling [Reference Doherty and Salik13]. Due to an inactive liver during the first few months of development, there is a deficiency of vitamin K-dependent clotting factors II, VII, IX, and X. As a result, preterm and term neonates have prolonged prothrombin time (PT) and activated partial thromboplastin time (aPTT).
Central Nervous System
Children are more vulnerable to head and spinal trauma than adults due to poor neck muscles and ligaments and a comparatively big head in comparison to body height [Reference Figaji15]. Additionally, the neonate’s spinal cord and dural sac stretch to the L3 and S4 vertebral levels, respectively. Since weak blood vessels and an abnormal cerebral autoregulation system predispose neonates born before 32 weeks’ gestation to intraventricular hemorrhage, up to 20% of children born before 32 weeks’ gestation suffer from interventricular hemorrhage [Reference Szpecht, Szymankiewicz, Nowak and Gadzinowski16]. Neonatal blood–brain barriers are immature, which increases resistance to lipid-soluble medications, and immature myelination reduces the influence of local anesthetics on the central nervous system [Reference Doherty and Salik13].
Glucose Regulation
The perinatal period is marked by improvements in endocrine activity, glucose homeostasis, and gluconeogenic enzyme levels [Reference Beardsall and Dunger17]. Hypoglycemia is a serious, but common, complication in neonatal growth [Reference Zijlmans, van Kempen, Serlie and Sauerwein18, Reference Bester and Pretorius19]. Preterm infants have depleted liver glycogen reserves, premature gluconeogenic enzymes, and immature hormonal responses [Reference Bester and Pretorius19]. Children who are preterm and/or small-for-gestational age are more likely to experience hypoglycemia when fasting. This is particularly true for children under the age of 3 [Reference Zijlmans, van Kempen, Serlie and Sauerwein18, Reference Bester and Pretorius19]. Prolonged hypoglycemia has been linked to long-lasting neurologic complications [Reference Beardsall and Dunger17–Reference Cowett and Loughead20]. However, glucose supplementation is not likely for healthy infants undergoing surgical procedures. This is likely due to hormonal stress decreasing glucose uptake and anesthetic agents decreasing metabolic demands. Children who are critically ill do require blood glucose monitoring and possibly intraoperative glucose supplementation.
Thermoregulation
Increased heat flow in preterm and term infants and its surroundings is facilitated by a large surface area-to-body volume ratio, narrow-diameter and prominent curvatures of body parts, low subcutaneous fat, and a thin and easily permeable epidermis [Reference Tourneux, Libert and Ghyselen21, Reference Soll22]. Related to their underdeveloped shivering processes, these patients depend heavily on nonshivering thermogenesis, a phase in which brown adipose tissue found in the spine, scapulae, kidneys, and adrenal glands is metabolically active [Reference Kaye, Fox and Padnos2, Reference Soll22, Reference Kumar, Shearer, Kumar and Darmstadt23]. It is important to maintain normal body temperature in children undergoing anesthetic agents. Lack of shivering in infants leads to their inability to recognize hypothermia [Reference Kumar, Shearer, Kumar and Darmstadt23]. Covering the child’s head can significantly reduce temperature loss. Forced air warming units have been shown to help maintain normothermia. Other measures, such as warming the operating room and adding humidity to the ventilator circuit, assist in maintaining normal physiologic temperatures in neonates and children.
Pharmacokinetics
Absorption
Volatile anesthetics enter the body by inhalation through lung alveoli where they enter the capillary beds. Owing to their increased alveolar airflow and comparatively poor functional residual potential, neonates and young children absorb volatile anesthetics more rapidly [Reference Stoelting, Hines and Marschall24, Reference Olsson25]. Drugs administered enterally in pediatric patients are usually absorbed more slowly than drugs administered in adults. This is because of narrower gastrointestinal tract, sluggish gastric emptying, immature secretion, and fewer protein transporters in children, as well as higher gastric pH in early childhood [Reference Edginton, Fotaki, Dressman and Reppas26, Reference Lu and Rosenbaum27].
Distribution
Factors affecting drug distribution include rate of blood flow, molecular size and solubility of the medication, and drug binding to plasma proteins and tissues. Pediatric patients usually have lower levels of plasma protein, lower body fat level, and a higher body water content, all of which affect the intensity of opioid delivery [Reference Lu and Rosenbaum27]. Reduced plasma protein levels result in an increase of free opioid molecules, while reduced body fat content results in an increase in drug level in the bloodstream due to lower volume for drug delivery [Reference Kaye, Fox and Padnos28]. On the other hand, higher amounts of overall body water increase the amount of drug delivery. The immature neonatal blood–brain barrier often affects opioid distribution, leading to heightened susceptibility to anesthetic agents in neonates [Reference Pardo and Miller29].
Metabolism
While the liver is the primary site of anesthetic agent metabolism, other sites of drug metabolism include the kidney, bowel, lung, and skin [Reference Lu and Rosenbaum27]. The cytochrome P450 pathway is largely responsible for completing drug metabolism in the liver. These enzymes are found at the cellular level within the endoplasmic reticulum and mitochondrial membranes. Drugs may remain in their prodrug form, from which the biologically active form is synthesized, but most drugs are inactivated during metabolism. Neonates have slightly lower hepatic enzyme levels and blood supply, which results in medications being metabolically active over a more prolonged period than in adult patients.
Excretion
Drug metabolites are excreted from the body via water, saliva, or exhaled breath. The liver excretes metabolites into bile, which then travels through the gastrointestinal tract to the intestines before excretion in the feces. Certain metabolites are reabsorbed from the gastrointestinal tract and eventually excreted in the urine via the kidneys. Children’s slower gastrointestinal activity lengthens the time required for metabolites to be reabsorbed from the intestines. The kidneys are not fully functioning before the 20th week of life, with decreased glomerular filtration rates, thus resulting in metabolites being excreted more slowly [Reference Pardo and Miller29].
Infection Prevention
Hospital-acquired infections are a significant source of morbidity and mortality in the healthcare setting. Infections cost billions of dollars per year and lead to extended hospital stays and higher mortality [Reference Brooks and Golianu30–Reference Macfarlane32]. Infection prevention and transmission should be a major goal in the organizational mission. Safety programs for both patients and healthcare workers should be implemented, with specifically designed accident prevention policies and procedures. Anesthesiologists are critical to the prevention of perioperative infections. Handwashing and decontamination are well-known means of infection prevention. Prophylactic antibiotics administered on time and temperature regulation in the operating room also decrease infection rates and are within the anesthesiologist’s control [Reference Bratzler, Dellinger and Olsen33–Reference Morse, Blackburn, Hannam, Voss and Anderson35].
Implementing best-practice bundles in pediatric intensive care units can decrease catheter-associated bloodstream infection rates, with maintenance bundles playing a critical role in infection reduction [Reference Miller, Griswold and Harris36]. These bundles provide measures to maintain the highest possible seal, sterile and clean dressings, and skincare before catheter insertion. Central line insertion bundles are considered standard of care.
Another consideration for anesthesiologists is the risk of contamination of peripherally inserted central lines after airway modulation. The oral cavity contains over 500 bacteria species, including Staphylococcus and Streptococcus strains [Reference Samaranayake and Matsubara37]. The anesthesiologist’s mask breathing, oral airway positioning, and endotracheal tube placement all provide the potential for bacterial infection and dissemination to other locations; these are also more likely to occur concurrently with, or before obtaining, vascular access in pediatric anesthesia, in contrast to adult anesthesia where vascular access is usually obtained before induction with anesthetic agents [Reference Martin, Kallile, Kanmanthreddy and Zerr38].
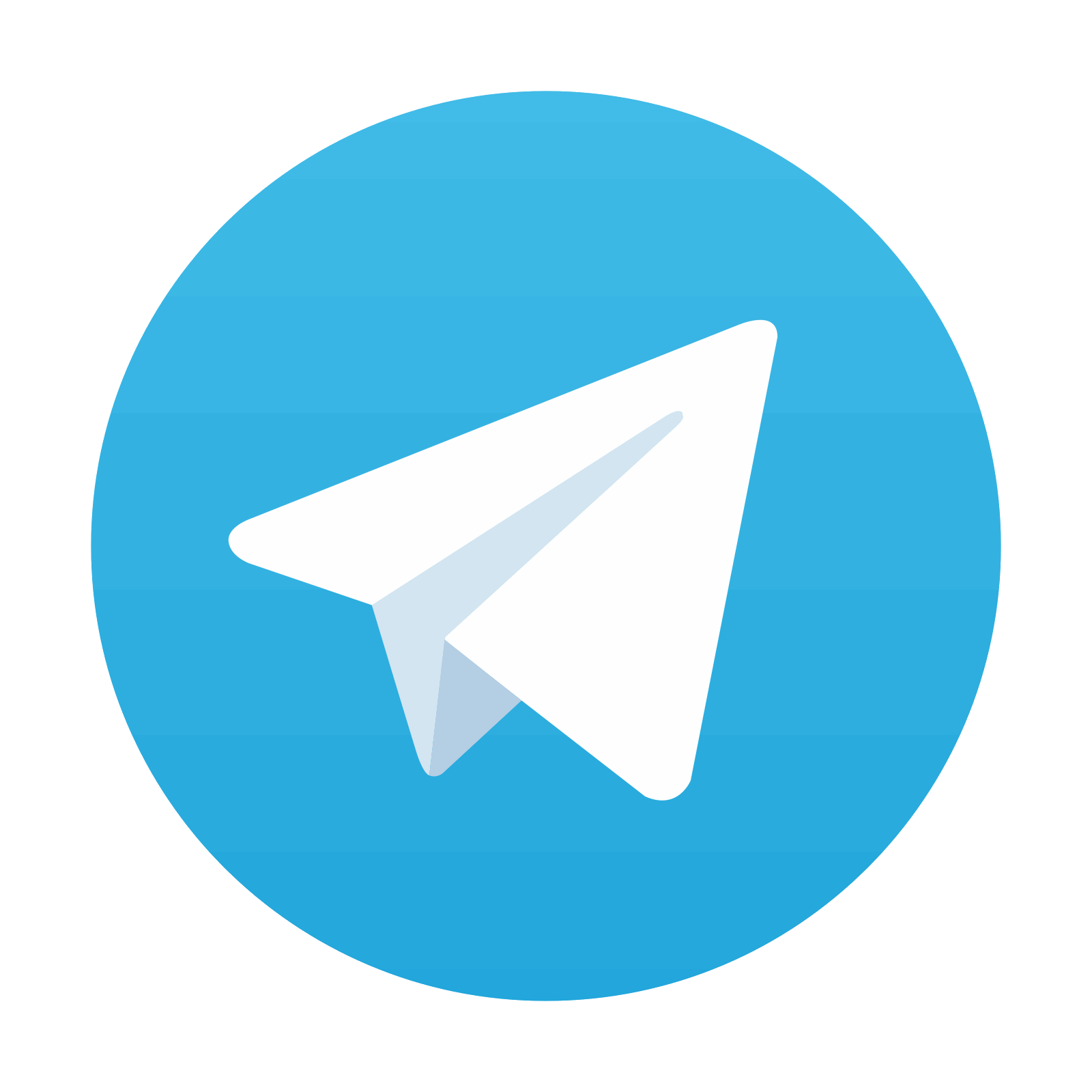
Stay updated, free articles. Join our Telegram channel

Full access? Get Clinical Tree
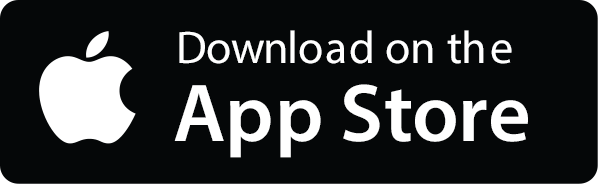
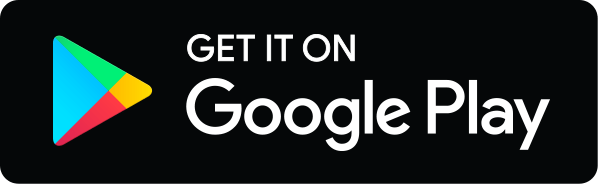
