Fluid and Electrolyte Disorders
Kevin Blackney
Jonathan Charnin
I. INTRODUCTION
A. Multiple systems are used to regulate fluids and electrolytes during “normal” states of health. In the perioperative period, homeostatic regulation may become unbalanced by periods of fasting, blood loss, medication effects, and blood pressure changes. Perioperatively, clini-cians must use all the available data to assist their patients in achieving and maintaining fluid and electrolyte balance. Failure to do so may result in delayed discharge from the postanesthesia care unit (PACU), unanticipated transfer from the PACU to the intensive care unit (ICU), and increased morbidity or mortality. Factors to be considered when replacing fluids or electrolytes include:
1. The type of surgical procedure performed and estimated blood loss
2. The volume and type of fluid administered intraoperatively
3. Baseline medical conditions (i.e., cardiac, pulmonary, and renal dis-ease)
B. Many strategies for fluid management have been proposed. Recently, two strategies have risen to prominence, sometimes referred to as “liberal” and “restrictive.”
1. Liberal fluid administration can cause the following complications:
a. Interstitial and pulmonary edema
b. Poor wound healing
c. Delayed gastric emptying/prolonged resumption of bowel function
d. Cardiovascular overload
2. Restrictive fluid administration can potentially lead to:
a. Hypotension and shock
b. Increased postoperative nausea/vomiting
3. The best approach to perioperative fluid management is still under debate.
C. Water in the human body resides in two fluid compartments: intracellular fluid (ICF) and extracellular fluid (ECF). The ICF constitutes approximately 67% of total body water (TBW), whereas the ECF consists of the remaining 33% TBW. The ECF is further broken down into the interstitial compartment (75% of the ECF) that surrounds cells and the plasma compartment, which comprises the remaining 25%. Excess fluid in the interstitial compartment leads to edema formation. The electrolyte composition in the ICF and ECF will differ, but the osmo-larity will be the same.
1. The “third space” refers to fluids, known as transcellular fluids, that are normally low in volume and high in turnover. Examples include pleural, peritoneal, and cerebrospinal fluid.
D. Movement of water between the compartments is governed by os-motic forces.
1. The cell membrane between the intracellular (ICF) and interstitial (ECF) compartments is a charged lipid bilayer that prevents free
movement of cations such as sodium and potassium. Movement of these ions is strictly through charged protein channels. This creates a transmembrane voltage potential for cell function. The lipid bilayer does allow water to freely pass from hyperosmolar to hypo-osmolar areas, thus creating an osmotic pressure gradient.
movement of cations such as sodium and potassium. Movement of these ions is strictly through charged protein channels. This creates a transmembrane voltage potential for cell function. The lipid bilayer does allow water to freely pass from hyperosmolar to hypo-osmolar areas, thus creating an osmotic pressure gradient.
2. In contrast, the capillary endothelium allows for free passage of both water and ions between the interstitial (ECF) and plasma (ECF) compartments. Therefore, the driving force for water movement here is the concentration of plasma proteins, especially albumin, which creates osmotic (i.e., oncotic) pressure, shifting water between these two compartments.
a. Starling’s law is used to describe fluid movement in this manner.
Jv = Kfc[(Pc – Pi) – δ(πc – πi)]
Jv is the transcapillary fluid flux, Kfc is the filtration coefficient across the capillary wall, Pc and Pi are the capillary and interstitial hydrostatic pressures, respectively, δ is the reflection coefficient, and πc and πi are the capillary and interstitial oncotic pressures. δ is the permeability of a substance through a capillary membrane.
b. In inflammatory states, δ is increased, allowing more proteins to leave the plasma compartment and enter the interstitial compartment. This creates an oncotic driving force toward interstitial accumulation and edema formation.
E. The electrolyte differences between the fluid compartments create membrane potentials important for cellular function.
1. The predominant ECF electrolyte creating an osmotic gradient is sodium. Chloride, calcium, and bicarbonate are also in higher concentration in ECF.
2. The predominant ICF cation is potassium. Proteins provide neutrality.
3. Gastrointestinal (GI) tract fluids contain elevated concentrations of potassium, chloride, and hydrogen ions. Thus, postoperative nausea and vomiting or gastric suctioning results in a hypokalemic, hypochloremic metabolic alkalosis.
II. ASSESSMENT OF FLUID STATUS
A. There is no single physiologic or biochemical marker that can act as a perfect indicator of fluid status and resuscitation. Clinicians therefore utilize assessment of vital signs, physical exam findings, and additional physiologic and biochemical testing.
B. Vital signs are likely the first indication of suboptimal fluid resuscitation in the postoperative patient.
1. Assuming the patient is not on a nodal blocking agent and other causes of tachycardia are ruled out, an increase in heart rate (HR) is often the first vital abnormality indicating hypovolemia (Table 23.1).
This is a normal physiologic response to hypovolemia, and treatment should be aimed at repleting fluid losses.
This is a normal physiologic response to hypovolemia, and treatment should be aimed at repleting fluid losses.
TABLE 23.1 Stages of Hypovolemic Shock | |||||||||||||||||||||||||
---|---|---|---|---|---|---|---|---|---|---|---|---|---|---|---|---|---|---|---|---|---|---|---|---|---|
|
2. As in evaluation of HR, assessing the blood pressure must be done after ruling out other causes of hypotension when assessing volume status in the PACU. Other causes include blood pressure-lowering medications, neuraxial anesthesia, and effects of residual anesthetic agents, to name a few.
3. Delta down (DD) and delta pulse pressure (DPP) are both effective dynamic indicators of hypovolemia in mechanically ventilated patients with arterial line monitoring. These correlate best when the patient is resting on a mechanical ventilator with 8 mL/kg tidal volumes, and positive end-expiratory pressure (PEEP) between 0 and 5 cm H2O. DD is defined as the difference in the systolic blood pressure (sBP) after a 5-second respiratory pause from the minimum sBP during a positive pressure breath. A difference of 5 mm Hg or more is considered a positive finding of hypovolemia. DPP is the difference of the maximal and minimal pulse pressure (PPmax and PPmin) during mechanically assisted breathing divided by the mean. A value of 13% or greater is considered a positive test for hypovolemia (Fig. 23.1). In the proper patient (i.e., no significant restrictive or valvular cardiac disease, no arrhythmias, no acute respiratory distress syndrome, normal abdominal compartment pressures, adequate sedation, closed chest), both of these methods correlate well in identifying patients with hypovolemia. The sensitivity and specificity of DPP for predicting hypovolemia has been shown to be as high as 94% and 96%, respectively, and DD has been shown to correlate well with DPP.
4. Urine output (UOP) can be difficult to analyze with reference to volume status in the PACU. Medications, physiologic changes from PPV, as well as the stress response induced by surgery that alters
release of antidiuretic hormone (ADH) and the renin-angiotensin system (RAS), can all affect normal UOP. In the first 48 hours postoperatively, the patient may continue to be oliguric, defined as a UOP < 0.5 mL/kg/hour. Furthermore, the serum concentration of ADH increases 50- to 100-fold during surgery and remains elevated for 3 to 5 days postoperatively. UOP should not be used as the lone indicator of fluid status.
release of antidiuretic hormone (ADH) and the renin-angiotensin system (RAS), can all affect normal UOP. In the first 48 hours postoperatively, the patient may continue to be oliguric, defined as a UOP < 0.5 mL/kg/hour. Furthermore, the serum concentration of ADH increases 50- to 100-fold during surgery and remains elevated for 3 to 5 days postoperatively. UOP should not be used as the lone indicator of fluid status.
5. Orthostasis is defined as an increase in HR of at least 10 beats per minute, or a decrease in SBP of at least 20 mm Hg when standing for 3 minutes after lying flat and supine. Performing orthostatic vital signs in the PACU is often not possible, and the accuracy of orthostatic vital signs in determining hypovolemia is poor.
C. The physical exam, when evaluating volume status, can include lung and heart auscultation, evaluation for jugular venous distension (JVD), hepatojugular reflex (HJR), and the skin. All of these can be difficult in the PACU. With the exception of pulmonary auscultation in fluid overload, none of these should be used as isolated findings.
1. Lung auscultation in fluid overload and pulmonary edema present as crackles. Crackles are nonmusical sounds heard in mid- to late inspiration and are gravity dependent. Studies about the accuracy of identifying pulmonary edema through auscultation of crackles vary, some showing a sensitivity and specificity of only 50% each. Identifying pulmonary edema early is important. A large retrospective study of 8,195 patients found that 7.6% of those undergoing major surgery developed pulmonary edema. Of that cohort, 11.9% died because of excessive fluid administration, especially in the first 36 hours postoperatively.
2. Heart auscultation may yield an S3 heart sound in overload states, but is otherwise of little yield in evaluating volume status.
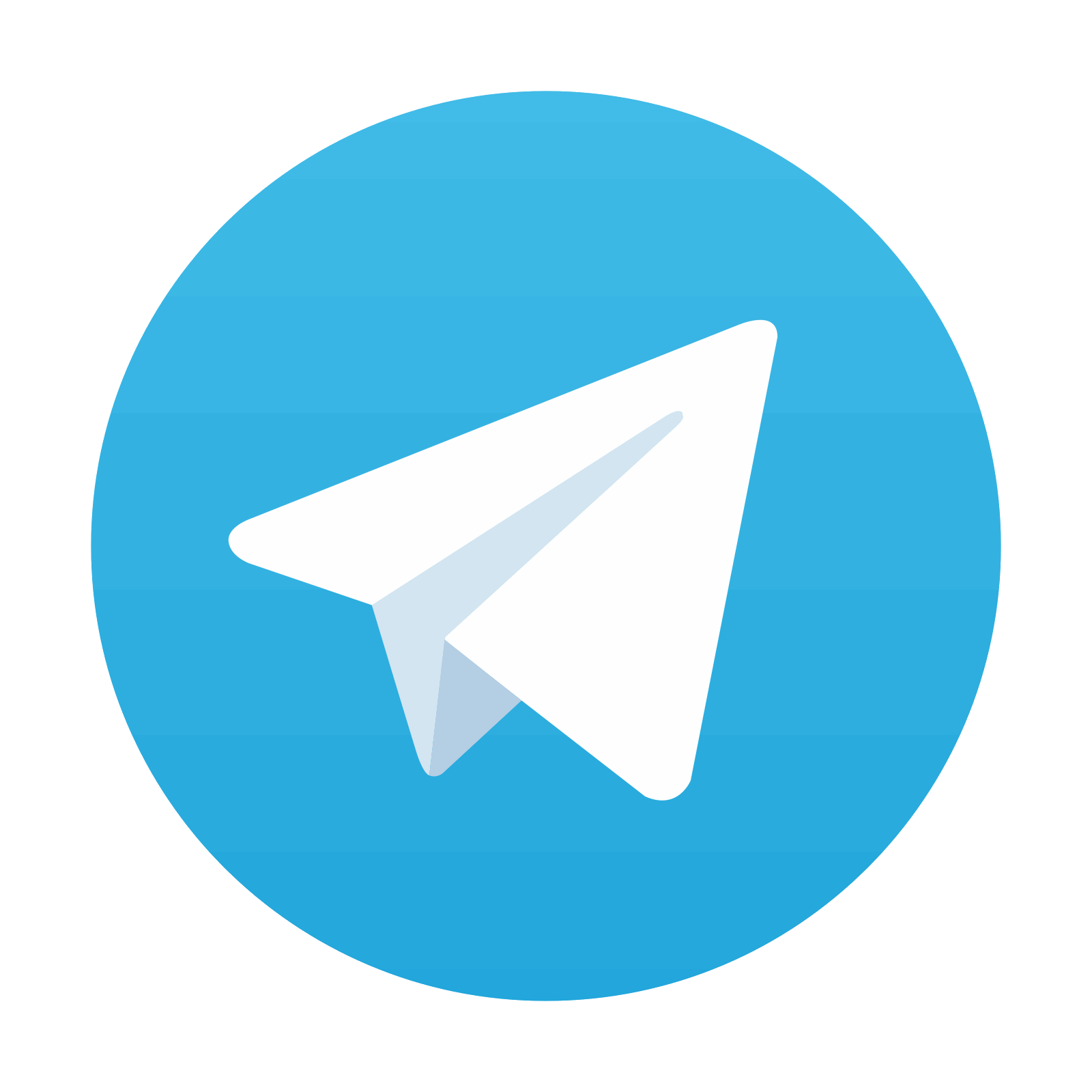
Stay updated, free articles. Join our Telegram channel

Full access? Get Clinical Tree
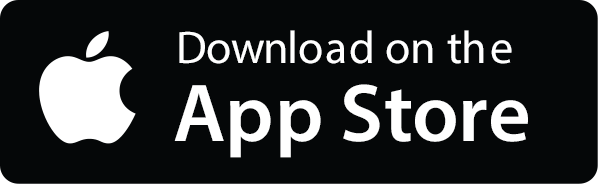
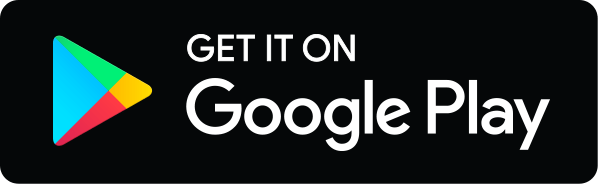