Abstract
BDZs modulate the effects of GABA at GABAA receptors. The specific α-subunit type determines the BDZ pharmacology – anxiolytic or sedative. Two BDZ receptor subtypes have been identified: BZ1, found in the spinal cord and cerebellum – responsible for anxiolysis; and BZ2, found in the spinal cord, hippocampus and cerebral cortex – responsible for sedative and anticonvulsant activity.
Hypnotics and Anxiolytics
Physiology
γ-Aminobutyric acid (GABA) is the main inhibitory neurotransmitter within the central nervous system (CNS) and acts via two different receptor subtypes, GABAA and GABAB:
GABAA – this receptor is a ligand-gated Cl− ion channel. It consists of five subunits (2α, β, δ and γ – each having a number of variants) arranged to form a central ion channel. GABA binds to and activates GABAA receptors and increases the opening frequency of its Cl− channel, augmenting Cl− conductance and thereby hyperpolarising the neuronal membrane. Cl− ion conductance is potentiated by the binding of benzodiazepines (BDZs) to the α subunit of the activated receptor complex. GABAA receptors are essentially (but not exclusively) postsynaptic and are widely distributed throughout the CNS.
GABAB – this receptor is metabotropic (i.e. acts via a G-protein and second messengers), and when stimulated it increases K+ conductance, thereby hyperpolarising the neuronal membrane. GABAB receptors are located both presynaptically on nerve terminals and postsynaptically in many regions of the brain, as well as in the dorsal horn of the spinal cord. Baclofen acts only via GABAB receptors to reduce spasticity.
BDZs modulate the effects of GABA at GABAA receptors. The specific α-subunit type determines the BDZ pharmacology – anxiolytic or sedative. Two BDZ receptor subtypes have been identified: BZ1, found in the spinal cord and cerebellum – responsible for anxiolysis; and BZ2, found in the spinal cord, hippocampus and cerebral cortex – responsible for sedative and anticonvulsant activity.
Benzodiazepines
Uses
Benzodiazepines are used commonly in anaesthesia as premedication and to sedate patients during minor procedures. They are also used more widely as anxiolytics, hypnotics and anticonvulsants.
Structure
At its core BDZs have two ring structures. The first is a benzene ring (see p. 84); the second has seven members (five carbon and two nitrogen) and is called a di-azepine ring. However, for pharmacological activity, BDZs also have a carbonyl group at position 2 on the di-azepine ring, another benzene ring and a halogen on the first benzene ring.
Midazolam
Presentation
Midazolam is presented as a clear solution at p. 3.5. It is unique among the BDZs in that its structure is dependent on the surrounding pH. At p. 3.5 its di-azepine ring structure is open, resulting in an ionised molecule, which is therefore water-soluble. However, when its surrounding pH is greater than 4, its di-azepine ring structure closes so that it is no longer ionised and therefore becomes lipid-soluble (see Figure 18.1). Its pKa is 6.5, so that at physiological p. 89% is present in an unionised form and available to cross lipid membranes.
Figure 18.1 Structures of midazolam.
As it is water-soluble it does not cause pain on injection.
Uses
Midazolam is used intravenously to sedate patients for minor procedures and has powerful amnesic properties. It is also used to sedate ventilated patients in intensive care.
Kinetics
Midazolam may be given orally (bioavailability approximately 40%), intranasally or intramuscularly as premedication. It has a short duration of action due to distribution. It is metabolised by hydroxylation to the active compound 1-α hydroxymidazolam, which is conjugated with glucuronic acid prior to renal excretion. Less than 5% is metabolised to oxazepam. It is highly protein-bound (approximately 95%) and has an elimination half-life of 1–4 hours. At 6–10 ml.kg−1.min−1 its clearance is larger than that of diazepam and lorazepam so that its effects wear off more rapidly following infusion (see Table 18.1).
Alfentanil is metabolised by the same hepatic P450 isoenzyme (3A3/4), and when administered together their effects may be prolonged.
Diazepam
Diazepam has a high lipid solubility, which facilitates its oral absorption and its rapid central effects. It is highly protein-bound (approximately 95%) to albumin and is metabolised in the liver by oxidation to desmethyldiazepam, oxazepam and temazepam, all of which are active. It does not induce hepatic enzymes. The glucuronide derivatives are excreted in the urine. It has the lowest clearance of the BDZs discussed here and its half-life is hugely increased by its use as an infusion (see Table 18.1; see also context-sensitive half-time, p. 78).
Diazepam may cause some cardiorespiratory depression. Liver failure and cimetidine will prolong its actions by reducing its metabolism. When administered with opioids or alcohol, respiratory depression may be more pronounced. In common with other BDZs it reduces the MAC of co-administered anaesthetic agents.
Lorazepam
Lorazepam shares similar pharmacokinetics and actions to other BDZs although its metabolites are inactive. It is used for premedication as an anxiolytic and amnesic. It is first-line therapy for status epilepticus (50–100 µg.kg−1 intravenous, sub lingual or per rectum from 1–12 years, maximum dose 4 mg; > 12 years, 4 mg).
Lorazepam is well absorbed following oral or intramuscular administration, highly plasma protein-bound (approximately 95%) and conjugated with glucuronic acid producing inactive metabolites, which are excreted in the urine (see Figure 18.2).
Figure 18.2 Metabolism of diazepam.*Less than 5% of temazepam is metabolised to oxazepam.
Temazepam
Temazepam is used as a nighttime sedative and as an anxiolytic premedicant. It has no unique features within the BDZ family. It is well absorbed in the gut, is 75% protein-bound and has a volume of distribution of 0.8 l.kg−1. Eighty per cent is excreted unchanged in the urine while glucuronidation occurs in the liver. Only a very small amount is demethylated to oxazepam. It has a half-life of about 8 hours and may result in some hangover effects.
Chlordiazepoxide
Chlordiazepoxide, the first BDZ derivative available for clinical use, is an anxiolytic sedative used to treat symptoms associated with alcohol withdrawl. When used to treat short term anxiety it is used at a dose from 5 mg tds up to 25 mg qds but this should be titrated carefully to the individual needs of the patient. It has a high oral bioavailbility and a complex metabolic pathway into a number of active metabolites. Its elimination half life is 5–30 hours which may increase in the elderly.
Flumazenil
Flumazenil is an imidazobenzodiazepine.
Uses
Flumazenil is used to reverse the effects of BDZs, that is, excessive sedation following minor procedures or in the treatment of BDZ overdose. However, its use is cautioned in mixed drug overdose as it may precipitate fits. It is given by intravenous injection in 100 µg increments and acts within 2 minutes. Its relatively short half-life (about 1 hour) compared with many BDZs means that further doses or an infusion may be needed.
Mechanism of Action
Flumazenil is a competitive BDZ antagonist. However, it has some agonist activity as well and its ability to precipitate seizures in certain patients may be a result of inverse agonist activity.
Effects
These include nausea and vomiting. It may also precipitate anxiety, agitation and seizures especially in epileptic patients.
Kinetics
Flumazenil is 50% plasma protein-bound and undergoes significant hepatic metabolism to inactive compounds that are excreted in the urine.
α-agonistsDexmedetomidine
Presentation and Uses
Dexmedetomidine is the S-enantiomer of medetomidine, the R- form having no pharmacological activity. It is used to produce light to moderate sedation (Richmond Agitation-Sedation scale) for (usually ventilated) patients in ICU, but has also been used for procedural sedation (including paediatric patients) and in balanced general anaesthesia. It is presented as a colourless solution in vials containing 100 μg.ml−1 and is typically diluted to a concentration of 4–8 μg.ml−1 prior to use.
Dose
For sedation in ICU it is used at an initial rate of 0.7 μg.kg−1.hr−1 which is adjusted to effect (range of 0.2–1.4 μg.kg−1.hr−1). When used alone or alongside other drugs in anaesthetic practice a loading dose of 1 μg.kg−1.hr−1 for 10 minutes may be used followed by a maintenance infusion of 0.2–0.6 μg.kg−1.hr−1. Larger infusion rates (5–10 μg.kg−1.hr−1) are described for the uncommon use as a sole agent for general anaesthesia.
Mechanism of Action
Dexmedetomidine is highly selective for the α2-receptor (α2:α1 ratio 1600:1), making it eight times more selective than clonidine. It inhibits the release of noradrenaline (NA) at the locus coeruleus of the pons which is intimately linked with the natural sleep pathways.
Effects
Central Nervous System – it induces sedation and anxiolysis. The sedation is more like physiological sleep than pharmacologically induced sedation or anaesthesia and this has been supported by EEG studies. It has been associated with less delirium compared to some drug protocols. It also produces analgesia by actions in the substantia gelatinosa of the dorsal horn of the spinal cord where it reduces the release of transmitters involved in nociception.
Cardiovascular – its effects here are biphasic, initially producing hypertension (mediated by α2B receptors on vascular smooth muscle), and then proceeding to hypotension and bradycardia as a result of reduced central NA release. This profile is more common during the loading dose phase and has been reported to progress to asystole, albeit rarely, when administered as a sole agent. However, it should be used with extreme caution in those with conduction defects.
Respiratory – it appears to have no respiratory impairment and the airway remains patent and may be especially useful during extubation. In addition, it has decongestant and antisialagogue effects which may be advantageous during sedation for fibreoptic intubation.
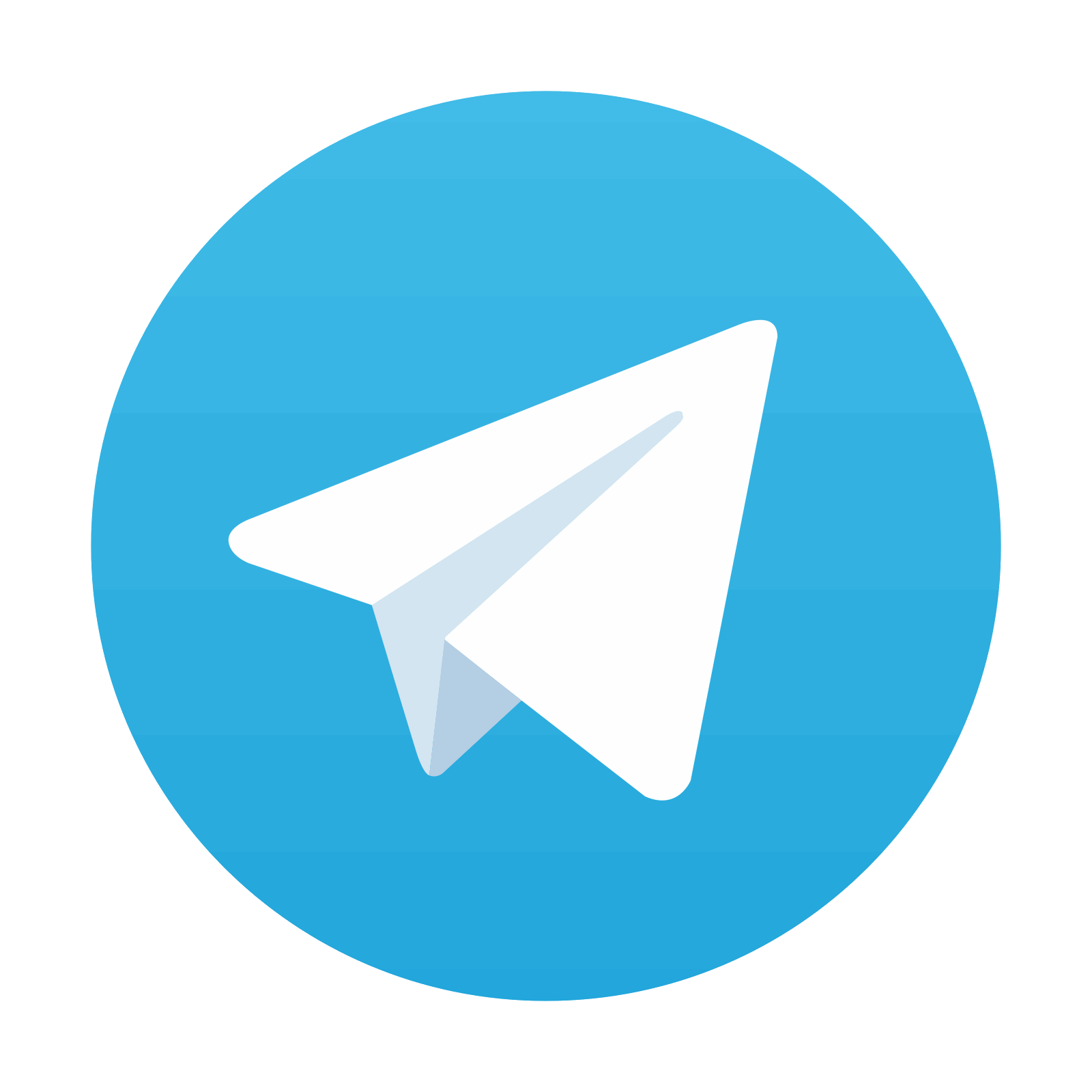
Stay updated, free articles. Join our Telegram channel

Full access? Get Clinical Tree
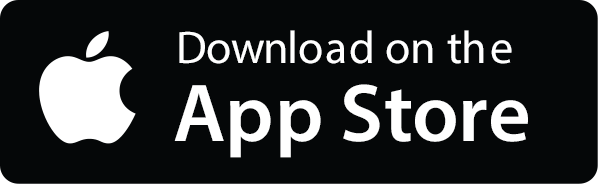
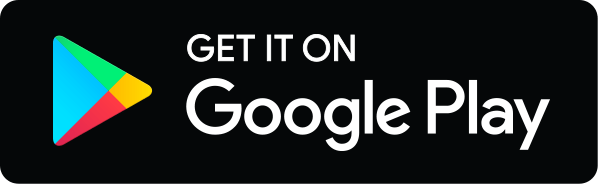
