CARDIOPULMONARY RESUSCITATION
SAGE R. MYERS, MD, MSCE, DANA A. SCHINASI, MD, AND FRANCES M. NADEL, MD, MSCE
GOALS OF EMERGENCY THERAPY
CPR is a series of interventions aimed at restoring and supporting vital function after apparent death. The immediate goal of resuscitation is to reestablish substrate delivery to meet the metabolic needs of the myocardium, brain, and other vital organs. The overall goal is to return the child to society without morbidity related to the underlying disease or the resuscitation process. Given the poor prognosis of children who have arrested, primary prevention efforts are crucial in improving childhood mortality. This chapter will focus on the management of the critically ill child and neonate based on the 2010 American Heart Association (AHA) CPR Resuscitation Guidelines, Pediatric Advanced Life Support (PALS) principles, the literature, and our experience. Of note, the next edition of the AHA Guidelines are expected in the fall of 2015.
INCIDENCE
It is estimated that of the 10,000 children who suffer an out-of-hospital cardiac arrest (OHCA) annually, only 5% to 12% will survive, most with significant neurologic injury. U.S. mortality rates related to age in years for 2010 and 2011 are shown in Table 4.1. Of note, mortality rates of children less than 1 year old approach the rates of people over 54 years old. Table 4.2 describes the leading causes of death for children and young adults. After the first year of life, unintentional injury is the most common cause of death and therefore, preventable.
RELATED CHAPTERS
Resuscitation and Stabilization
• A General Approach to Ill and Injured Children: Chapter 1
• Approach to the Injured Child: Chapter 2
• Interfacility Transport and Stabilization: Chapter 6
Medical Emergencies
• Cardiac Emergencies: Chapter 94
Procedures and Appendices
PATIENT CHARACTERISTICS
Age
Children less than 1 year of age have a much higher incidence and worse rate of survival from OHCA than older children or teens. In large population-based studies of children who were resuscitated by the Emergency Medical Services (EMS) after an OHCA, 39% to 44% are infants. Pediatric CPR education should focus on anatomic and physiologic characteristics of the young child; but the emergency department (ED) staff must be prepared to cope with the full spectrum of age and size. In fact, in the Children’s Hospital of Philadelphia Emergency Department, 4% of the patients requiring resuscitative services were adults.
Etiology
OHCA encountered in the ED results from trauma (20% to 30% of cases), sudden infant death syndrome (SIDS), or other causes. Though the literature varies in defining the cause of arrest, a primary cardiac etiology occurs in about 13% of children, as compared to 72% of adults. Approximately 10% of OHCA patients have ventricular tachycardia (VT) or ventricular fibrillation (VF). Children with congenital anomalies, chronic sequelae of prematurity or birth trauma, and those with chronic relapsing disease are also seen in the ED, as increasing numbers of children have survived the neonatal period, transplantation, complex surgery, and cancer therapy and have been discharged from the hospital.
Pediatric cardiopulmonary arrest follows one of two pathways: (1) Respiratory distress followed by respiratory failure and secondary cardiac arrest; or (2) primary cardiac arrest due to cardiac disease. In our experience, the majority of children suffer an asphyxial arrest (Fig. 4.1).
Demographics
There are few demographic studies to identify socioeconomic, ethnic/racial, familial, or community characteristics of children who require life support interventions. A recent multi-city study of children older than a year showed a predominance of males and children of black race. In addition, the majority of pediatric arrests occurred in private residences; less than half were witnessed arrests. It is difficult to generalize this information as the sample was limited to an urban population. National demographic studies are important however for developing profiles of high-risk patient populations for subsequent development of surveillance or prevention programs, as well as targeted community-based education initiatives. Some states have begun child death review efforts that shed light on prevention strategies. On a national level, the Cardiac Arrest Registry to Enhance Survival (CARES) is an EMS-based registry for OHCA in 29 participating US cities. Data on both pediatric and adult OHCA events and outcomes are collected with the goal to improve resuscitative care. On a local level, hospital-based quality improvement programs can help to track the population of patients drawn from one hospital’s catchment area.
TABLE 4.1
DEATHS AND DEATH RATES, BY AGE, UNITED STATESa
FIGURE 4.1 Pathophysiologic pathways from etiologies to cardiac arrest. (Adapted from PALS, American Heart Association.)
TABLE 4.2
10 LEADING CAUSES OF DEATH BY AGE GROUP, UNITED STATES, 2012
TREATMENT OVERVIEW
Pediatric CPR presents the emergency physician with many challenges. The child may arrive from EMS without the same level of field treatment that adult patients receive routinely.
The overwhelming majority of cardiac/respiratory arrests occur in adults so paramedics have limited exposure, a lower comfort level and less success in advanced life skills for children. The wide spectrum of age, weight, and diagnoses adds additional complexity and equipment needs (see Chapter 139 Prehospital Care).
The chain of survival after an OHCA arrest includes rapid access to EMS, rapid CPR, rapid defibrillation when indicated, and rapid advanced care. Once in the ED, resuscitation is best accomplished with an effective leader who organizes and directs a skilled team. The American College of Surgeons divides trauma resuscitation into the primary and secondary surveys (see Chapter 2 Approach to the Injured Child), and the application of this technique to medical resuscitation can allow for rapid, thorough assessment of the patient in an orderly manner. The primary survey is the assessment of airway, breathing, circulation, and disability (ABCDs). The secondary survey is a head-to-toe examination to determine the etiology of the arrest, while concurrently, diagnostic studies are done, consultants are called, and arrangements for definitive care are completed (see Chapter 1 A General Approach to Ill and Injured Children). The ABCDs are reassessed frequently throughout the resuscitation.
Our experience shows that careful management of airway and breathing is extremely important in children. Because the cause of the arrest is most often related to respiratory failure and the child’s myocardium is relatively resilient to hypoxemia, the rapid correction of hypoxemia may be all that is necessary to restore circulation. Bag-valve-mask (BVM) ventilation is an effective way to rapidly address hypoxemia and, in the prehospital setting, BMV alone (without subsequent intubation) has been shown to improve outcomes for patients with respiratory arrest in urban settings.
For those patients who do not respond to airway and breathing management alone, life support interventions are more complex. In the ED, the lack of an immediate patient response usually predicts a need for multiple drug interventions.
Vascular access is a major challenge in severely ill pediatric patients. Peripheral intravenous access may be difficult to establish quickly. The intraosseous (IO) route is a safe, effective method to obtain access rapidly. In fact, PALS recommends immediate IO access for a patient in cardiac arrest. Central lines are useful for longer-term access but require advanced skills and often take more time to place.
Arrhythmia management is a relatively infrequent problem in pediatric life support. The absence of atherosclerotic vascular disease makes the child’s myocardium less susceptible to arrhythmias; and a minority of arrested children present with a shockable rhythm. As a result, antiarrhythmic medications and defibrillation are infrequently used. The most common cardiac rhythms to be recognized and managed in pediatric arrest are sinus bradycardia, pulseless electrical activity (PEA), and asystole. The exceptions to this are those children with congenital heart disease and those who have sustained direct myocardial trauma (see Chapter 94 Cardiac Emergencies). These children may have unusual and difficult arrhythmias that require subspecialist expertise to achieve a successful outcome.
Finally, there remains much to be learned on how to care for children requiring resuscitation. Researchers in CPR face many challenges. In the past, much of the work was done at single sites retrospectively. The creation of research networks and CPR registries has greatly expanded the possibility for prospective, large, multicentered trials. CPR research has unique ethical and legal considerations. Exception from informed consent is an evolving concept in studies of children with a life-threatening condition that has furthered recent work. Patient populations, characteristics, terminology, and methodology vary among studies, making it difficult to compare results. To address these concerns, experts developed and issued the Utstein Criteria that has brought some uniformity to CPR research terminology.
PROGNOSIS
Survival after CPR in children is variable. Despite advances in medical care of the critically ill child and improved EMS systems, OHCA survival remains poor. Less than 10% of children who suffer an OHCA survive, usually with significant neurologic injury (Table 4.3). Unfortunately, about 60% to 88% of OHCA are unwitnessed events and only about 1⁄3 of children receive bystander CPR, which may contribute to their poor prognosis. However, if a hospitalized child has respiratory arrest that is recognized rapidly and managed skillfully, immediate survival may be as high as 90%. Overall, children resuscitated after an in-hospital arrest (IHA) have a more favorable outcome with about 30% or more of children surviving to hospital discharge (see Table 4.4). Earlier recognition of prearrest phases, advances in medical care and education, and rapid response teams have likely contributed to the improved survival over time seen in IHA.
TABLE 4.3
OUTCOME FOR OUT-OF-HOSPITAL PEDIATRIC CARDIOPULMONARY ARREST
TABLE 4.4
OUTCOME FOR IN-HOSPITAL PEDIATRIC CARDIOPULMONARY ARREST
CLINICAL MANIFESTATIONS
The clinical manifestations of persons requiring immediate life support are most often related to failure of oxygen delivery to the skin, brain, kidneys, and cardiovascular system. One can identify most potential or existing life-threatening conditions by assessing a child’s general appearance and abnormalities in their airway, breathing, and circulation (ABCs) as outlined in Chapter 1 A General Approach to Ill and Injured Children (Fig. 4.2). It is essential to identify patients who are at risk for failure of substrate delivery as early intervention prevents respiratory failure and subsequent cardiovascular collapse.
MANAGEMENT
Management Sequence
Figure 4.3 outlines an efficient tool to rapidly assess and manage critically ill or injured patients.
C-A-B Versus ABC. For basic life support, the 2010 AHA guidelines recommend that the rescuer begin chest compressions first in an effort to remove obstacles associated with airway interventions and decrease delays in resuscitation. Healthcare providers should tailor all resuscitation recommendations to meet the needs of the individual patient; thus interventions occur simultaneously using ABC approach.
AIRWAY
Evaluation
The overwhelming majority of pediatric arrests are due to asphyxia; the recognition and treatment of airway obstruction and respiratory failure is crucial.
Despite good evidence that prehospital intubation in children does not improve outcomes over BVM ventilation in areas without prolonged transit time, many children are still intubated in the field. When a child arrives in the ED with an ETT in place, verify position immediately. For stable patients, measured end-tidal CO2 (ETCO2) (continuous is optimal) and chest radiograph confirms placement. In arrested patients, interpretation of ETCO2 and breath sounds may be unreliable; in addition to these strategies, tube placement is confirmed by direct laryngoscopy. All patients should have continuous pulse oximetry, ETCO2, and cardiorespiratory monitors placed immediately.
FIGURE 4.2 Signs and symptoms of lack of substrate delivery to vital organ systems. CNS, central nervous system.
FIGURE 4.3 Management sequence for pediatric life support. CNS, central nervous system; EKG, electrocardiogram; BP, blood pressure; BUN, blood urea nitrogen; Ca++, calcium.
MANAGEMENT
Airway Positioning
If cervical trauma is suspected, the head and cervical spine must be stabilized (during all airway maneuvers). Airway obstruction is most often related to relaxation of the jaw and neck muscles causing the tongue and mandibular tissues to fall posteriorly against the posterior wall of the hypopharynx. Airway positioning maneuvers, such as the head tilt–chin lift and the jaw thrust, are used first to relieve obstruction (Fig. 4.4). Jaw thrust alone is used if cervical stabilization is needed.
Artificial Airways
If airway positioning fails to relieve obstruction, oropharyngeal (OPA) or nasopharyngeal artificial airways (NPA) may be used.
Oropharyngeal Airways
Estimate OPA size by placing it against the side of the child’s face; with the flange at the corner of the mouth assuring that the tip ends just proximal to the angle of the mandible; place it using a tongue depressor (Fig. 4.5). OPAs are used in unconscious patients only. If not inserted properly or too short, the OPA may push the tongue backward into the posterior pharynx, aggravating airway obstruction. If the OPA is too long, it may touch the larynx and stimulate vomiting or laryngospasm.
Nasopharyngeal Airways
The correct NPA size covers the distance from the nares to the tragus of the ear (Fig. 4.6). The NPA can be used in conscious patients. The NPA may lacerate the vascular adenoidal tissue, thus adenoidal hypertrophy and bleeding diatheses are relative contraindications to the use of these airways.
Endotracheal Tubes
ET tubes are used to overcome upper airway obstruction, isolate the larynx from the pharynx, allow mechanical aspiration of secretions from the tracheal bronchial tree, and facilitate mechanical ventilation or end-expiratory pressure delivery (see Chapter 3 Airway).
BREATHING
Evaluation
Breathing is assessed through observation of chest wall movement and auscultation. Gas exchange is confirmed by auscultation and monitoring of ETCO2 and pulse oximetry.
FIGURE 4.4 A: Upper airway obstruction related to hypotonia. B: Partial relief of airway obstruction by means of head extension (danger of cervical spine injury in cases of trauma). C: Extreme hyperextension causing upper airway obstruction. D: Fully open airway through use of jaw thrust or jaw lift. E: Oropharyngeal airway stenting mandibular block off of posterior pharyngeal wall.
MANAGEMENT
Spontaneous Ventilation
Supplemental oxygen is administered to the spontaneously breathing ill patient. If the patient is not breathing spontaneously, positive pressure ventilation is required. Though the optimal concentration is not known, it is reasonable to provide 100% oxygen during CPR. Hyperoxia is a mediator of postresuscitation injury, thus titration of Fio2 to the minimum concentration to achieve saturation of at least 94% is recommended.
FIGURE 4.5 Oropharyngeal airway: flange (A), bite block (B), stent (C), and gas exchange or suction conduit (D).
FIGURE 4.6 Nasopharyngeal airways in a variety of sizes.
Oxygen Delivery Devices
A variety of oxygen delivery devices are available for use in patients who have patent airways. The oxygen delivered depends on the child’s size and minute ventilation.
Nasal Cannulas
One hundred percent humidified oxygen is delivered to the nares at a flow of 4 to 6 L per minute. Due to entrainment of room air, the final oxygen delivery is low, usually 30% to 40%.
High Flow Nasal Cannulas
High flow nasal cannula (HFNC) allows the delivery of humidified and warmed oxygen/gas at flow rates up to 12 L per minute in infants and 30 L per minute in children. This increased flow allows for noninvasive continuous positive airway pressure (CPAP) and has been used as an alternative to CPAP devices, especially for infants with bronchiolitis.
Oxygen Masks
There are several types of oxygen masks that offer a wide range of inspired oxygen concentrations.
Simple Masks. The simple face mask delivers a moderate Fio2 that varies from 35% to 60%.
Partial Rebreathing Masks. Partial rebreathing masks allow reliable delivery of an Fio2 of 50% to 60%. When the flow in the reservoir bag is greater than the patient’s minute ventilation and the oxygen is adjusted so the bag does not collapse during inhalation, there is negligible CO2 rebreathing allowing for more reliable oxygen delivery.
Nonrebreathing Masks. These masks have nonrebreathing valves incorporated into the face mask and the reservoir bag and reliably provide oxygen concentrations up to 95% with high flow rates 10 to 15 L per minute.
Continuous Positive Airway Pressure Devices
CPAP provides positive pressure to stent open the child’s airways leading to improved ventilation and oxygenation. CPAP can be applied with large nasal prongs, a nasal mask which covers just the nose of the patient, or a full face mask which covers the mouth and nose.
Assisted Ventilation
BLS rescue breathing rates in infants and children with respiratory arrest is 12 to 20 breaths per minute, with higher rates for infants and younger children. Newly born infants may need a rate of 40 to 60 breaths per minute. For children and infants who require CPR, the recommended respiratory rate is 8 to 10 breaths per minute. Ventilations are asynchronous with chest compressions when an advanced airway is in place. Ventilations are coordinated with chest compressions with a rate of 15 compressions to 2 ventilations (100 to 120 compressions per minute) when an advanced airway is not in place. With all ventilation techniques, the force and volume needed to just see the chest rise is recommended. Overventilation is a common error in resuscitation; in the AHA 2013 Consensus Statement, a ventilation rate of less than 12 and a ventilation volume that causes no more than minimal chest wall rise during CPR is emphasized.
Because of risk of infection transmission, mouth-to-mouth resuscitation is no longer recommended. Instead, rescue breathing should be done with a pocket mask. Placement of the mask over the mouth alone, over the mouth and nose, or over a tracheostomy site depends on the patient and the equipment available.
Expired Air Techniques
Bag-valve-mask ventilation is an essential skill for all emergency medicine clinicians and provides a rapid means to provide oxygenation and ventilation for children with respiratory and/or circulatory failure.
Masks
The properly fit mask covers the tip of the chin, the mouth, and the nose allowing for a tight seal against the skin. Masks with a pneumatic cuff design allow for the easiest and most efficient fit that avoids air leaks. When enough personnel are available, a two-person technique may improve oxygen delivery and ventilation. One person uses both hands to correctly position the mask on the face creating a good seal, while the other squeezes the bag paying attention to ensure appropriate chest rise and ventilation.
Hand-squeezed, Self-inflating Resuscitators
Hand-squeezed, self-inflating resuscitators are easy to use and do not require a gas source. The elasticity of a self-inflating bag allows it to refill with oxygen or room air as the gas intake valve opens. During compression the gas intake valve closes, and a second valve opens to allow flow into the patient. A valve between the mask and the bag allows for patient exhalation into the atmosphere. Most self-inflating bags are equipped with a pressure-limiting pop-off valve that is preset at 35 cm H2O to prevent barotrauma; if not, an inline manometer should be used (Fig. 4.7). To deliver oxygen concentrations 60% to 90%, use a flow rate of 10 to 15 L per minute. Use a minimum of a 500-mL bag for infants and children.
Anesthesia Bags
Anesthesia breathing circuits (sometimes called a Mapleson circuit) consist of a source of fresh gas flow attached to a reservoir bag with an adjustable pressure-limiting (APL) valve (Fig. 4.8). These devices are favored by many for several reasons. When there is no significant leak at the mask-to-patient interface, CPAP can be provided to the patient airway and the pressure adjusted with the APL valve. Positive pressure ventilation can also be provided by squeezing the bag. The respiratory effort can be observed through changes in the filling and emptying of the reservoir bag. Additionally, if the fresh gas flow is provided through an oxygen blender, any desired concentration of oxygen may be provided. However, there are several disadvantages with this type of bag. First, a high-pressure source of fresh gas flow is necessary for the system to function at all. Second, significant experience in maintaining a complete seal at the mask-to-patient interface is needed in order to maintain positive airway pressure and positive pressure ventilation can occur. The operator must adjust the fresh gas flow rate and the APL opening with the patient ventilation to balance rate of gas escape from circuit to prevent over or under filling. If the bag is removed from a leak-tight patient application, it promptly deflates and one must wait for the reservoir to refill. Alternatively, overfilling the bag may result in dangerously high-pressure transmission to the lung and stomach. Third, exhaled gases return to the circuit and bag which can lead to hypercarbia unless the fresh gas flow is great enough (generally twice the patient’s minute ventilation) to “washout” the circuit. These disadvantages have prompted many to recommend the primary use of the self-inflating resuscitator bag as the primary mode of ventilation.
FIGURE 4.7 Self-inflating hand-powered resuscitator: compressible unit (A), oxygen source (B), oxygen reservoir (C), one-way valve assembly (D), and mask with transparent body (E).
FIGURE 4.8 Anesthesia bag.
Mechanical Ventilators
In children who require prolonged or relatively high minute ventilation, inspiratory pressures, or positive end-expiratory pressures (PEEPs), the use of a mechanical ventilator may provide better management compared to manual ventilation. Care must be taken to assure that the appropriate tidal volumes and pressures are set for patient size and treatment goals. We recommend the use of mechanical ventilator only if the providers are skilled in the use of the device or in consultation with a pediatric expert and appropriate support from Respiratory Therapy.
CIRCULATION
The 2010 AHA guidelines emphasize immediate, high-quality chest compressions. Adult cardiac arrest survival has improved with immediate chest compressions and rapid defibrillation. Pediatric primary asphyxial arrest requires a different strategy including rapid recognition of prearrest signs and well-executed airway management and immediate chest compressions at the onset of circulatory arrest.
Evaluation
The 2010 AHA guidelines minimize the importance of the pulse check by healthcare providers as it is neither quickly nor reliably assessed. A clinician should take no more than 10 seconds to determine the presence of a brachial, femoral, or carotid pulse. Evaluate the effectiveness of circulation by observing skin and mucous membrane color, and checking capillary refill (Fig. 4.9). Continuous electrocardiogram (EKG) monitoring and frequent blood pressure measurements are required. Most modern defibrillators have “quick-look” paddles to allow for rapid evaluation of cardiac rhythm. Defibrillator adhesive pads provide both continuous EKG monitoring and defibrillation and can remain in place while external cardiac compression (ECC) is being performed.
Management
Management may be divided into five phases: (i) Cardiac compression, (ii) establishment of an intravascular access, (iii) use of primary drugs, (iv) use of secondary drugs, and (v) defibrillation.
EXTERNAL CARDIAC COMPRESSION
Well-executed ECC is the primary treatment for cardiopulmonary arrest. The AHA 2010 Guidelines and 2013 Consensus Statement recommend the following: push hard (1⁄3 chest diameter), push fast (at least 100 per minute), allow full chest recoil, minimize interruptions (<10 seconds) in chest compressions, avoid hyperventilation (8 to 10 beats per minute [bpm]), and change rescuers at least every 2 minutes. With the patient on a firm surface, compress the lower half of the sternum, avoiding the xiphoid process. The compression depth is about one-third to one-half the AP diameter of the chest or 2 in in children and 1½ in in infants. For infants (<1 year), the Thaler or two thumb-encircling hands technique is the preferred method for healthcare providers (Fig. 4.10). The recommended rate of compressions for all ages except neonates is at 100 to 120 compressions per minute. The duration of the compression and the relaxation phases should be equal. Blood flow and cardiac output are impaired by incomplete chest wall release that occurs when the rescuer leans over the patient or does not pause at the end of the compression phase. Prior to intubation, compressions and ventilations should be coordinated in a 15:2 ratio (Table 4.5). Once the airway has been secured, coordination of compressions and rescue breathing is no longer necessary. Interruptions in chest compressions result in diminished cardiac output and are associated with poorer outcomes.
FIGURE 4.9 Delayed capillary refill.
Previously, ED rescuers had little more than the presence of the femoral artery pulse with compressions as a means of quickly assessing the adequacy of ECC. Newer techniques such as ETCO2 monitors and accelerometer sensors allow for the rapid and continuous monitoring of ECC quality. Exhaled CO2 rises as pulmonary blood flow and cardiac output increases; an ETCO2 of less than 10 mm Hg may indicate inadequate chest compression technique. Patients who have return of spontaneous circulation (ROSC) often first demonstrate an abrupt increase in ETCO2 to 35 mm Hg or greater. An accelerometer/force sensor monitor provides real-time feedback of CPR quality. A sensor puck is placed on the child’s chest and provides visual and verbal feedback about ECC quality. Though previously only available for children >8 years old, new pediatric electrodes with CPR rate and depth sensor capabilities are now available for infants and children less than 8 years old.
FIGURE 4.10 External cardiac compression using the Thaler method of thumbs encircling the chest. A: Infant receiving chest compressions with thumb 1 fingerbreadth below the nipple line and hands encircling chest. B: Hand position for chest encirclement technique for external chest compressions in neonates. Thumbs are side by side over the lower third of the sternum. In the small newborn, thumbs may need to be superimposed (inset).
In the newly arrested child, vigorous, high-quality chest compressions generate approximately one-third of the normal cardiac output. This generates a coronary artery perfusion pressure (CPP) of approximately 10 to 20 mm Hg. CPP determines the blood flow to the myocardium and is equivalent to the difference between the aortic pressure and the right atrial pressure. A minimum CPP of 5 to 10 mm Hg is needed to produce any myocardial blood flow. Increased CPP to levels that allow for myocardial perfusion during CPR is associated with ROSC. Pauses in CPR result in lower CPPs, supporting recent AHA efforts to minimize interruptions in compressions during resuscitation.
The mechanism by which blood moves during CPR continues to be the subject of investigation. Techniques that augment this forward flow could potentially improve the rates of successful ROSC and neurologic survival. “Direct compression” and “thoracic pump” describe the current mechanisms that explain blood flow during CPR (see Fig. 4.11). In the “direct compression” model, the heart is squeezed between the sternum and the posterior vertebrae. During compression (systole), blood moves through the AV valves and the aorta. During relaxation (diastole), blood fills the myocardium in preparation for the next systole. In the “thoracic pump” model, the heart is viewed as a conduit. During compression, venous valves at the thoracic inlet close preventing retrograde flow, the venous side of the circulation is compressed, and blood moves forward through the AV valves and the aorta. During relaxation, negative intrathoracic pressures suck blood into the pulmonary bed and heart in preparation for the next systole. In practice, both methods likely contribute to blood flow. Because of the compliance and elasticity of the chest wall and the intrathoracic structures, direct compression may play a larger role in the pediatric patient.
TABLE 4.5
CHEST COMPRESSION PARAMETERS
FIGURE 4.11 Blood flow during external cardiac compression. RV, right ventricle; LV, left ventricle.
Based on the previous data, investigators have explored different techniques to increase blood flow through the aorta and the coronary arteries. These have included high-frequency compression rates (more than 100 compressions per minute), interposed abdominal compression CPR (IAC-CPR), active compression–decompression CPR (ACD-CPR) using a suction device applied to the chest, vest CPR, open chest massage, simultaneous ventilation–compression CPR, and use of automated feedback devices that monitor the quality of CPR. To date, compression rates and automated performance feedback have been the most promising. The other techniques are not supported by pediatric data and their use is not indicated.
Compression rates affect the cardiac output. During CPR, CPP rises during consecutive chest compressions and falls during pauses. Compression rates of more than 100 have been shown to improve cardiac output, CPP, and 24-hour survival when compared with rates less than 80. Although the optimal compression rate remains unknown, compression rate of at least 100 per minute is now recommended for all ages beyond the neonatal period. There is some evidence in adults that compression-only CPR (no ventilation) produces similar survival rates. Because of the etiology of pediatric arrest, in addition to a higher metabolic rate and lower functional residual capacity, this practice is not recommended for children with an asphyxial arrest.
The optimal duty cycle (compression:relaxation) also remains unknown. To date, a duty cycle of 50% is believed to provide the highest flow rates. Leaning or incomplete decompression of the chest during the relaxation phase occurs commonly and may affect the cardiac output generated by CPR by decreasing venous return.
Open cardiac massage provides better blood flow to vital organs in animals and in adults when compared with CPR. However, because of the compliance of the thoracic structures in children, this technique may not offer any benefit. There is inadequate data to recommend its use in the resuscitation of children who have suffered a medical cause of their arrest.
In the newly arrested patients, CPR should be provided immediately to establish a minimum of circulation to the brain and heart, as this is associated with improvements in both survival and outcome. Though healthcare providers may assume mastery of this seemingly basic skill, many studies have demonstrated that the quality of CPR administered in both OHCA and IHA meets the AHA standards <50% of the time. Providers fail to adhere to recommended chest compression rate and depth; interruptions, overventilation, and leaning are common. In a case series of out-of-hospital arrests, paramedics provided chest compressions only half of the available time. In adults, ROSC is associated with a higher chest compression rate and an increased depth of compression and decreased pre- and postcompression pauses related to defibrillation. Survivors are more likely to have received rates between 85 and 100 and the average depth of compression 2.4 mm deeper than nonsurvivors.
Though a rescuer may not feel fatigued, chest compression quality deteriorates within the first 2 minutes of chest compressions, leading to the current recommendation of switching providers every 2 minutes. However, frequent changes in providers cause an interruption in compressions and new compressors may be more likely to lean during the first compressions. Some providers may maintain high-quality compressions beyond the recommended 2-minute limit. Using a real-time CPR feedback system may provide objective information regarding when to change compressors. In Bobrow’s study of adults with an OHCA, chest compression rate, depth, ventilation rate, and preshock pause were all improved when audiovisual feedback was used in combination with rescuer retraining. Most importantly, feedback and training improved survival by 30%.
INTRAVENOUS ACCESS
The site used for vascular access depends on the patient’s condition and the provider’s experience. The most common sites used in the ill pediatric patient include peripheral venous access, IO access, and central venous access via the femoral vein.
In the arrested patient, IO access is indicated and can be accomplished quickly (30 to 60 seconds) and provides a route for all drugs and fluids needed during resuscitation. The onset of action of drugs administered via this route is comparable to that of drugs administered into the central circulation. Adenosine, which is rapidly metabolized once administered, may not work when given via the IO. Manual pressure, a push–pull fluid delivery system or use of a pressure bag is necessary when giving fluids to restore the vascular volume in order to overcome the resistance of the marrow venous plexus. The preferred site in children is the medial surface of the tibia 1 to 3 cm below the tibial tuberosity Alternative sites include the anterior surface of the distal femur, proximal to the medial malleolus, and the anterior iliac spine (Fig. 4.12). There are several types of rigid, styletted needles commercially available for this procedure in infants and children. There are also semiautomated IO devices available for use in children. The bone injection gun (BIG) is a spring-loaded device that can be used in adolescents/adults and can effectively penetrate the thicker bony cortex. The EZ-IO (Vidacare, San Antonio, TX) a battery-powered handheld drill, is available for use in both children and adults. Further research is needed to evaluate efficacy of the semiautomated IO placement devices as compared to manual IO needles. Contraindications to IO placement include recently fractured bone, osteogenesis imperfecta, and osteopetrosis. Complications are rare and have been reported in less than 1% of patients. These have included extravasation, epiphyseal injury, fracture, compartment syndrome, fat embolism, and thrombosis (see Chapter 141 Procedures).
FIGURE 4.12 Intraosseous needle placed in distal femur.
Peripheral venous access provides an adequate route for prearrest resuscitations. Veins of the hands, forearm, and ankle are most commonly used. Prolonged unsuccessful attempts at IV access in critically ill or injured children delay fluid or medication administration as well as interfere with other life-saving procedures; use of the IO should not be delayed. Recent advances in the use of bedside ultrasound by the emergency clinician have increased the placement of ultrasound-guided peripheral IVs, especially in patients with difficult IV access. An IV escalation plan should be developed and in place (see Chapter 142 Ultrasound).
The femoral vein is the easiest central vein to access in the critically ill child and has less complications. Central venous access provides a more secure route, allows the capability of monitoring central venous pressure, and allows for blood sampling. In adults, this route has been shown to provide more rapid onset of action and higher peak drug levels that theoretically could affect outcome. This has not been shown to be the case in the pediatric patient. In a child with uncompensated shock or arrest, IO access is obtained first for rapid initial resuscitation and central venous access may follow (see Chapter 141 Procedures).
DRUGS OF RESUSCITATION
Estimating Body Weight
Drug doses, fluid therapy, and equipment size is weight and size based. The 50th percentile weight from a standardized growth curve can be used to estimate weight based by known/estimated age. The Broselow tape allows a simple, accurate method of estimating the weight and drug doses based on the measured height (Fig. 4.13). In the absence of compelling research, AHA currently recommends using the actual or tape-based weight for obese children rather than an ideal weight. Drug doses should never exceed the adult maximum dose. Many handheld computer programs are available to calculate pediatric resuscitation drug doses (see Table 4.6 for doses and comments on common resuscitation medications).
Alternative Routes of Medication Administration
Intravenous administration, including IO, is the preferred route for medications. However, the pulmonary bed provides a surface for absorption of lipid-soluble drugs and can be used if vascular access is unavailable. Currently, lidocaine, epinephrine, atropine, and naloxone (LEAN) can be used via this route. The optimal drug dosage remains unknown as absorption likely varies widely due to its dependence on pulmonary blood flow. Animal studies have shown that standard doses of epinephrine (0.01 mg per kg) achieve serum levels that are approximately 10% of those achieved by the intravascular route. Thus, the recommended dose of epinephrine to be given via the ET tube is 0.1 mg per kg, 10 times the recommended IV dose. Larger doses of the LEAN drugs are also recommended ranging from 2 to 10 times the IV dose. All drugs are diluted in 5 mL of saline and given with five manual ventilations to distribute it across the alveolar surface. Other routes of medication administration for patients for which vascular access has not yet been obtained include intramuscular (epinephrine for anaphylaxis), subcutaneous (terbutaline for status asthmaticus), and transmucosal, including rectal mucosa, buccal mucosa, and nasal mucosa (benzodiazepines for status epilepticus).
FIGURE 4.13 Broselow tape for determining drug dosage schedule based on patient length. A: Placement of tape. B: Equipment size and drug dosage schedule printed on tape.
FLUID RESUSCITATION
Isotonic crystalloids (normal saline or lactated Ringer solution) in 20 mL per kg aliquots remain the mainstay of acute volume resuscitation. Dextrose solutions should not be used in the initial phases of fluid resuscitation. For children, it is important to recognize signs of shock (poor end organ perfusion) and treat with isotonic crystalloid boluses even if the blood pressure is normal.
Hypertonic saline causes an osmotic shift of fluid from the intracellular and interstitial spaces to the extracellular compartment, providing rapid volume expansion with less interstitial edema. In addition, less volume is required, which can be given over a shorter period of time. Hypertonic solutions are also believed to reduce ICP by establishing an osmotic gradient across the blood–brain barrier that draws water from the brain into the vascular space. Conversely, potential ill effects include continued hemorrhage from injured blood vessels, and increased ICP due to leakage of sodium through a disrupted blood–brain barrier. Currently, data does not support the use of hypertonic saline over isotonic crystalloid for the resuscitation of patients.
Serum albumin concentration has been shown to be inversely related to mortality risk. Thus, its use in the resuscitation of ill patients has been explored. It is 30 times more expensive than crystalloid solutions and has limited availability. Systematic reviews have failed to show benefit from its administration. Albumin is believed to have some anticoagulant properties and may leak across the capillary wall promoting edema.
DEFIBRILLATION AND CARDIOVERSION
The true prevalence of VT and VF in children with cardiopulmonary arrest in published reports is approximately 10% in both IHA and OHCA pediatric arrest (Table 4.7). An additional 10% to 15% will develop VT/VF as a subsequent rhythm. Although the need for defibrillation is relatively uncommon, it must always be considered in older children, children with a history of congenital heart disease or dysrhythmias, and children who experience a witnessed sudden collapse arrest. Interestingly, a recent secondary analysis of the CARES registry found that though younger children (1 to 8 years) with OHCA had a relatively low incidence of an initial shockable rhythm (11%) as expected, older children (9 to 18 years old) had an incidence of an initial shockable rhythm similar to that of adults (32%).
Defibrillation is the asynchronous delivery of a shock to the myocardium in an attempt to produce simultaneous depolarization of a critical mass of myocardial cells to allow spontaneous repolarization and the resumption of a perfusing cardiac rhythm. Most defibrillators deliver biphasic wave forms allowing for successful defibrillation at a lower energy of 150 J. Standard adult paddles, 8 to 13 cm in diameter and pediatric paddles 4.5 cm in diameter, are available with most defibrillators. The correct paddle size is that which makes complete uniform contact with the chest wall. The large paddle can usually be used for infants older than 1 year of age and/or weighing more than 10 kg. Larger paddle surfaces result in decreased intrathoracic impedance and optimize the energy reaching the myocardium. Electrode paste decreases impedance and prevents skin injury. Paddles are applied anteriorly at the right, upper chest below the clavicle and to the left of the nipple in the anterior axillary line directly over the heart; they are applied with pressure and should never touch each other. Most defibrillators also have disposable hands-free adhesive pads that allow both rhythm recognition and shock delivery. These can be applied in the same location on the arrested child immediately at the beginning of the resuscitation and aid in preventing delays in rhythm checks and treatment. The initial dose for defibrillation is 2 J per kg, increased to 4 J per kg if the first attempt is unsuccessful.
Automated external defibrillators (AEDs) automatically interpret the cardiac rhythm and, if pulseless VT/VF is present, advise the operator to deliver a charge. They are small, easy to use, and have batteries that last for 5 years. For patients with pulseless VT/VF, early rapid defibrillation is the treatment of choice. AEDs have been proven to be highly sensitive and specific when used on adults, and there is good evidence that its use in the out-of-hospital setting has resulted in a dramatic improvement in survival of adults with VF.
TABLE 4.6
DRUGS OF RESUSCITATION
TABLE 4.7
PRESENTING RHYTHM IN PEDIATRIC PATIENTS WITH OUT-OF-HOSPITAL CARDIOPULMONARY ARREST AND IN-HOSPITAL CARDIOPULMONARY ARREST (%)
Pediatric-based EKG rhythm analysis algorithms for AEDs are 99% sensitive and specific for determining shockable and nonshockable pediatric rhythms.
Available AEDs deliver a standard adult charge between 150 and 200 J, they can be used for children >1 year of age. An attenuating pediatric electrode system is available which decreases the charge delivered to 50 J and is preferred if available for children 1 to 8 year olds. For infants <1 year, a manual defibrillator is preferred, but an AED equipped to deliver pediatric charge doses may be used. If neither of these is available, an AED may be used.
Synchronous cardioversion is delivered with the patient’s R wave, reducing the risk of VF by avoiding delivery of the charge during the T wave. This is indicated for treatment of perfusing rhythms, when a pulse is present, such as stable VT or SVT. The initial charge for synchronized cardioversion is 0.5 to 1 J per kg. This dose can be doubled to 2 J per kg if the tachydysrhythmia persists. Sedation and analgesia should be considered during this procedure.
SPECIFIC RESUSCITATION SCENARIOS
In the 2010 AHA resuscitation guidelines, an international expert panel updated guidelines for pediatric prearrest/arrest scenarios. Figures 4.14–4.16 summarize these guidelines along with the following commentary. It is important to remember that no algorithm can cover every clinical situation; the treating team of healthcare professionals must consider the many etiologies of arrest and modify therapies accordingly for the individual patient. Early recognition and immediate treatment of respiratory and circulatory failure and frequent reassessment are the foundation of successful resuscitation efforts for all scenarios encountered in the ED.
FIGURE 4.14 PALS Bradycardia Algorithm. ABCs, A, airway, B, breathing, C, circulation; AV, atrioventricular; CR, cardiorespiratory; EKG, electrocardiogram; ETI, endotracheal intubation; Hgb, hemoglobin; HR, heart rate; IV/IO, intravenous/intraosseous. (Source: American Heart Association, Inc.)
Bradycardia with a Pulse and Poor Perfusion
Bradycardia most commonly results from impending or existing respiratory failure (Fig. 4.14). As always, early recognition and intervention to support the respiratory system and other vital functions reduces morbidity and may be life-saving. Other etiologies of bradycardia include heart block, heart transplant, increased ICP, hypoglycemia, hypercalcemia, drug effect, increased parasympathetic tone, and hypothermia. The first step in the treatment of children with bradycardia is airway management. Remember that because increase in heart rate is the primary mechanism by which children increase their stroke volume, bradycardia readily leads to hypotension. Chest compressions should begin when perfusion is inadequate. Assess the rhythm on the cardiac monitor. If the bradycardia is believed to be due to increased vagal tone or heart block, atropine is an appropriate intervention. For most situations, epinephrine is the drug of choice. In children with heart block, cardiac pacing may be necessary.
Pulseless Arrest
Asystole and PEA are the most common arrest scenarios encountered in children (Fig. 4.15). In the pulseless child, the rhythm must be identified so the correct intervention can be instituted. In a witnessed, sudden collapse arrest, primary cardiac etiologies should be considered. For asystole and PEA, standard dose epinephrine is the drug of choice. Always consider treatment of reversible causes such as hypovolemia, hypothermia, electrolyte abnormalities, poisonings, tension pneumothorax, and cardiac tamponade.
Treatment for pulseless VT/VF is immediate CPR and defibrillation. In the 2005 guidelines, the recommendation for single defibrillation replaced the recommendation for three successive shocks. Biphasic defibrillators have a higher first shock success rate and the administration of three successive shocks leads to delays in CPR that is associated with decreased survival. Cardiac compressions are interrupted only for rhythm checks and shock delivery. If defibrillation is unsuccessful, standard dose epinephrine is the drug of choice. The use of vasopressin may be considered. Amiodarone is the preferred drug for refractory pulseless VT/VF Magnesium is indicated if the rhythm is torsades de pointes VT. The pattern of interventions is CPR→ rhythm check→ resume CPR while charging defibrillator → deliver single shock→ resume CPR→ brief rhythm check → resume CPR as drug is prepared and administered. Interruptions in CPR should be held to very brief periods to check the rhythm and to administer shocks. The resuscitation team should anticipate the need for the next interventions.
FIGURE 4.15 PALS Pulseless Arrest Algorithm. ABCs, A, airway, B, breathing, C, circulation; CPR, cardiopulmonary resuscitation; ETCO2, end tidal CO2; ETI, endotracheal intubation; IO, intraosseous; IV, intravenous; PEA, pulseless electrical activity; PTX, pneumothorax; ROSC, return of spontaneous circulation; VF, ventricular fibrillation; VT, ventricular tachycardia. (Source: American Heart Association, Inc.)
Tachydysrhythmias with a Pulse and Poor Perfusion
The differential diagnosis of tachycardias includes sinus tachycardia (ST), SVT, and VT. Narrow complex morphology and beat-to-beat variability are usually present in children with ST (Fig. 14.16). Rates rarely exceed 220 bpm in infants and 180 bpm in children. Common causes of ST include hypoxemia, hypovolemia, hyperthermia, metabolic abnormalities, and pain/anxiety. Therapy is directed at treating the underlying cause.
SVT can be distinguished from ST by its lack of beat-to-beat variability and rate (most often >220 bpm in infants, and >180 bpm in children). In children, aberrant conduction yielding a wide complex rhythm SVT occurs less than 10% of the time. SVT is most commonly caused by accessory reentry pathways. Patients with stable SVT have adequate oxygenation and perfusion; those with unstable SVT have inadequate perfusion and thus require rapid intervention. Chemical or electrical conversion can be used for the treatment of unstable SVT. Because of the efficacy and safety of adenosine in the treatment of SVTs, it is the drug of choice when vascular or IO access is available. In infants, verapamil can cause myocardial depression or arrest and should be used only in consultation with a cardiologist. It is optimal to obtain a 12-lead EKG prior to and during treatment to aid in the diagnosis. If the patient fails to convert to sinus rhythm after two doses of adenosine, synchronized cardioversion (0.5 J per kg) is recommended. Use of sedation/analgesia should be considered. Vagal maneuvers were reintroduced in the 2000 AHA guidelines for stable SVT. In infants and young children, ice may be applied to the face; in older children, carotid massage and Valsalva maneuvers, such as knee to chest or forceful blowing on an obstructed straw, may be attempted. Ocular pressure should be avoided. Use of other therapies such as procainamide, digoxin, and beta-blockers may be considered after pediatric cardiology consultation.
FIGURE 4.16 PALS Tachycardia with Pulses and Poor Perfusion Algorithm. ABCs, A, airway, B, breathing, C, circulation; CBC, complete blood count; CR, cardiorespiratory; EKG, electrocardiogram; ETCO2, end tidal CO2; ETI, endotracheal intubation; Hgb, hemoglobin; HR, heart rate; IV/IO, intravenous/intraosseous; RR, respiratory rate; SVT, supraventricular tachycardia; VT, ventricular tachycardia; WOB, work of breathing. (Source: American Heart Association, Inc.)
VT is characterized by wide complex (QRS >0.08 second) and typically has a rate ranging from 120 to 200 bpm. Etiologies of VT include prolonged QT syndrome, structural heart disease, myocarditis, cardiomyopathy, and poisonings. In children presenting with stable VT, close monitoring and immediate consultation with a pediatric cardiologist to determine etiology and definitive treatment is the best management. For children with unstable VT, begin with synchronized cardioversion. For hemodynamically stable patients, consider chemical conversion using amiodarone or procainamide in conjunction with consultation with an expert.
EXTRACORPOREAL CARDIOPULMONARY RESUSCITATION
There is now some information regarding the use of extracorporeal CPR (E-CPR) through the use of extracorporeal membrane oxygenation (ECMO) or cardiopulmonary bypass following CPR to treat refractory cardiac arrest in children presenting to an ED setting. Currently, it is an option available in large, tertiary care Children’s Hospitals. The majority of the literature to date comes from the treatment of inpatients with primary cardiac disease. Use of E-CPR for these patients following a witnessed arrest seems to increase the chance for favorable outcome. The 2010 Guidelines state “There is insufficient evidence to recommend the routine use of ECPR for patients in cardiac arrest. However in settings where ECPR is readily available, it may be considered when the time without blood flow is brief and the condition leading to the cardiac arrest is reversible or amenable to heart transplantation or revascularization.” Use of E-CPR may be considered for children who have had a short downtime and have received high-quality CPR and a cardiac etiology is suspected when the resources and personnel are available.
FIGURE 4.17 Newborn Resuscitation Algorithm. CR, cardiorespiratory; CPAP, continuous positive airway pressure; ETI, endotracheal intubation; HR, heart rate. (Source: American Heart Association, Inc.)
NEWLY BORN INFANT RESUSCITATION
Guidelines for CPR in the newly born child and for newborns in the neonatal intensive care unit (NICU) differ from guidelines for CPR of the young infant presenting to the ED (Fig. 4.17). To avoid confusion, the AHA recommends that the PALS CPR algorithm be used for all nonnewly born pediatric patients presenting in arrest to the ED, PICU, and other non-NICU settings given that there is no scientific evidence to support the use of either algorithm over the other in these patients.
Though rare, the ED team must be prepared for the resuscitation of the newly born. Fortunately, 90% of neonates transition from intrauterine to extrauterine life without resuscitative needs beyond simple warming and stimulation. However, the remaining 10% require some assistance, and 1% will require extensive resuscitative efforts. Resuscitative needs vary greatly by birth weight. Approximately 6% of term newborns will require resuscitation at birth, compared with nearly 80% of infants weighing less than 1,500 g. The key to a successful newborn resuscitation for the ED team includes preparedness of staff and equipment and anticipation of high-risk births.
Emergency Department Preparedness
Early notification allows time to assemble important team members. Education of staff, necessary equipment, and specific policies and procedures are critical for preparedness. Multiple trained personnel must be available for high-risk deliveries. In addition to a standard obstetric tray, every ED should have a newborn resuscitation kit that is readily accessible, maintained, and rapidly restocked after use. Necessary equipment and medications are listed in Table 4.8. A medication dosing chart by weight and a radiant warmer are invaluable. Because neonatal resuscitations in the ED are uncommon, simulations and mock codes allow staff to remain familiar with neonatal resuscitation skills and supplies. Most births that occur outside the delivery room have high-risk components such as trauma-induced labor and unexpected or teenage pregnancy. Important history includes prematurity, multiple gestation, meconium-stained amniotic fluid, and maternal drug use. The team can then anticipate the need for assisted ventilation, simultaneous resuscitations, tracheal suctioning, or pharmacologic interventions. Table 4.9 lists other risk factors associated with the need for neonatal resuscitation.
TABLE 4.8
NEONATAL RESUSCITATION EQUIPMENT AND DRUGS
TABLE 4.9
NEONATAL HIGH-RISK PROFILE
Pathophysiology
Physiology of Intrauterine Development
Full alveolar development and sufficient surfactant production is not complete until 34 weeks’ gestation. Prior to 23 to 24 weeks’ gestation, there is a lack of surfactant and terminal airways have not developed, therefore resuscitation prior to 23 to 24 weeks is generally futile.
Changes at Birth
The fetus has two large right-to-left shunts: One from the right atrium to the left atrium through the foramen ovale, and the second from the pulmonary artery to the aorta across the ductus arteriosus. At birth, two major changes occur that eliminate these shunts: the umbilical cord is clamped, and then respirations are initiated. Expansion of the lungs increases the neonate’s Pao2 and pH, which causes pulmonary vasodilation and a fall in pulmonary vascular resistance. The initial breaths taken by the infant must inflate the lungs and effect a change in vascular pressures so that the lung water is absorbed into the pulmonary arterial system and cleared from the lung. This inflation pressure is a powerful mechanism for the release of pulmonary surfactant, which increases compliance of the lung and establishes functional residual capacity.
Asphyxia
Neonatal asphyxia can result from multiple factors. The initial response to asphyxia is hyperpnea and sinus tachycardia. If there is no significant increase in Pao2, after 2 to 3 minutes, respirations will stop (primary apnea). During primary apnea, stimulation such as drying or slapping of the feet will restart breathing. If the apnea is prolonged (more than 1.5 minutes), the infant becomes bradycardic and may attempt gasping, nonrhythmic respiratory efforts while the heart rate continues to fall. Soon thereafter, the child ceases to gasp (secondary apnea) and stimulation will not cause the infant to resume breathing. Ventilatory support must be initiated for the newborn to survive. Brain and other organ damage progresses rapidly beyond this point.
Management
Initiation and Termination of Resuscitation
Noninitiation of resuscitation is appropriate in conditions associated with high mortality or poor outcome such as a gestational age less than 23 weeks or birth weight of less than 400 g or anencephaly. For conditions associated with a high rate of survival and acceptable morbidity such as gestational age ≥25 weeks and most congenital anomalies, resuscitation should be initiated. Though the often-precipitous nature of ED births may make it difficult, it is important to include parents in the discussion regarding the initiation and continuation of resuscitative efforts. Resuscitative efforts should be performed on any term infant; if there is any question of viability of premature infants, it is probably best to initiate resuscitative efforts.
After 10 minutes of a well-executed resuscitation, if the heart rate remains undetectable, it is reasonable to stop resuscitative efforts. Consultation with a neonatal expert may be helpful in making such a decision.
Initial Management Priorities
The initial assessment and management occur quickly and often simultaneously. For the term, vigorous baby, routine care and reunification with the mother are all that is usually necessary. The initial steps of neonatal resuscitation include positioning and clearing the airway, drying and warming with prevention of heat loss, stimulating, and repositioning. These steps occur within 30 seconds and are followed immediately by an evaluation of respirations, heart rate, and color. When possible, all resuscitation equipment should be ready for use, the radiant warmer on, and a team with preassigned roles assembled. Figure 4.17 is a flow diagram of neonatal resuscitation.
Thermoregulation
Ambient temperature in the ED is lower than ideal for a newborn. All infants, especially very low–birth-weight (VLBW) and premature babies, are at great risk of hypothermia because of their greater body-surface-area-to-weight ratio, minimal fat stores, and thinner epidermis and dermis. Hypothermia increases metabolic needs and produces hypoxia, hypercarbia, metabolic acidosis, and hypoglycemia and delays normal transition.
As the patient is dried and placed under a radiant warmer, the temperature should be monitored via the axillary route using electronic thermometers with a disposable tip. Normal axillary temperatures fall between 36.5°C and 37.4°C. Rectal temperatures are reserved for infants whose core temperature may be in question. Alternative methods of warming infants, particularly while awaiting a radiant warmer in the case of an unexpected delivery, include warm blankets and towels. Placing the dried infant naked against the mother’s body and covering both mother and infant with blankets may also warm the stable infant. For VLBW infants, additional methods of warming include wrapping the baby in food grade plastic wrap, using exothermic mattresses or a radiant heater and increasing the room’s ambient temperature. Monitor the temperature carefully as these methods may lead to hyperthermia. Although preventing heat loss is vital, hyperthermia should be avoided because it is associated with perinatal respiratory depression and hypoxic-ischemic injury may be worsened.
Suctioning
Many newborns have excessive secretions, including amniotic fluid, cervical mucus, and meconium that can generally be removed by placing the infant on his or her side and gently suctioning the mouth and then the nose with a bulb syringe. Mechanical suction with an 8F or 10F suction catheter may also be used. To avoid soft tissue injury, negative pressure from mechanical suctioning should not exceed 100 mm Hg. Suctioning of the oropharynx in a newborn is likely to cause vagally mediated bradycardia and/or apnea. Excessive suctioning may also contribute to atelectasis. Therefore, suctioning is reserved for the newly born with obvious obstruction to breathing or those requiring positive pressure ventilation (PPV).
Stimulation
Most newborns will begin effective breathing during stimulation from routine drying and suctioning. Other methods of safe stimulation include flicking the heels and rubbing the back of the newborn infant. More vigorous methods of stimulation are unnecessary and may be associated with harmful consequences. If, after a brief period of stimulation, suctioning, and drying (no more than 30 seconds) effective respirations have not been established, PPV is initiated (Fig. 4.17).
Airway Positioning
If airway obstruction persists after routine suctioning, reposition the airway. Correct positioning, with the neck slightly extended in the “sniffing position,” aligns the posterior pharynx, larynx, and trachea and facilitates air entry. This maneuver may also be accomplished by placing a towel or blanket beneath the upper back of the supine infant. Avoid flexion or hyperextension of the newborn’s neck, which is likely to exacerbate airway obstruction.
Administration of Oxygen. Recent studies have shown better survival for newborns resuscitated with air compared to 100% oxygen. Sustained high levels of oxygen may be toxic. The AHA recommends newborns SpO2 should correlate with a range of normal values as described in Table 4.10. To achieve the targeted SpO2, clinicians should use air or blended oxygen when available, titrating the amount of oxygen based on the pulse oximetry. If the baby remains bradycardic after 90 seconds of air or blended oxygen, 100% oxygen should be started. Deliver warm, humidified oxygen when possible to maintain temperature.
Airway and Breathing
One large observational study noted that initial management steps and ventilation were effective in establishing normal vital signs in more than 99% of newly born infants. Therefore, particular attention must be paid to providing skilled ventilation interventions for compromised newborns in the ED.
Bag-valve-mask Ventilation
If initial management interventions are unsuccessful and the newborn is still not breathing or is gasping, or the heart rate is less than 100 bpm, PPV must be initiated. For the infant’s first breath, a relatively higher inflation pressure, between 25 and 40 cm H2O, delivered slowly over several seconds is commonly used. Subsequent ventilations typically require less pressure (15 to 20 cm H2O for normal lungs and 20 to 40 cm H2O for diseased or immature lungs), and are best judged by good chest wall rise and breath sounds and heart rate response. An assisted ventilatory rate of 40 to 60 breaths per minute will provide effective ventilation and oxygenation.
TABLE 4.10
TARGETED PREDUCTAL SPO2 AFTER BIRTH
PPV is similar to infants and children with a few caveats. First, relatively small volumes of air (approximately 4 to 6 mL per kg) are delivered to the newly born. A 450-mL self-inflating bag rather than the larger bags should be used to avoid complications from barotrauma. Many self-inflating bags have a pressure-limiting pop-off valve set at 30 to 45 cm H2O, occlusion of this valve may be necessary if initial high inflation pressures are needed for the first breath.
If bag-valve-mask ventilation is required for longer than several minutes, an orogastric tube should be placed to decompress the stomach. If respirations are restored and the heart rate is >100 bpm, PPV may be slowly discontinued. If respirations remain inadequate or the HR <100 bpm, assisted ventilation must be continued and endotracheal (ET) intubation must be considered.
Endotracheal Intubation
In the event that there is a prolonged need for PPV or mask ventilation has not been effective in restoring vital functions, ET intubation is indicated. Chapter 3 Airway reviews advanced airway management in depth and this generally applies to the newly born with a few differences. Sizes of airway equipment can be determined by birth weight (Table 4.11). ET tube size can be estimated by gestational age: ETT size in mm = Gestational age in weeks divided by 10. Thus, a 35-week premature infant would require a 3.5-mm ET tube. Proper ET tube insertion depth is estimated as follows: Total cm at gum line = 6 + Weight of the infant in kg.
End Expiratory Pressure
For centers skilled in the respiratory management of neonates, CPAP may be an alternative to ET intubation in a spontaneously breathing preterm newborn with respiratory distress.
Laryngeal Mask Airways
LMAs can be successfully used for ventilating full-term or near-term newborns, particularly in cases of ineffective bag-valve-mask ventilation or failed ET intubation. Although LMAs may be used as a secondary device to ventilate newborns by healthcare practitioners skilled in their use, data supporting use of LMAs in preterm infants are insufficient to routinely recommend their use in this scenario.
TABLE 4.11
SELECTION OF AIRWAY EQUIPMENT BY WEIGHT
TABLE 4.12
MEDICATIONS FOR NEONATAL RESUSCITATION
Circulation
Chest Compressions. Chest compressions are needed in less than 0.1% of all births. Bradycardia and asystole are virtually always a result of respiratory failure, hypoxemia, and tissue acidosis and are successfully treated with airway management. Chest compressions are indicated if the heart rate remains less than 60 bpm despite 30 seconds of PPV.
The chest compression:ventilation ratio in the newly born is 3:1 aiming for 90 chest compressions and 30 ventilations in 1 minute (rate of 120 events per minute) (Table 4.5). The Thayer technique is the preferred means of ECC (Fig. 4.10).
Vascular Access. The umbilical vein is considered the preferred site for vascular access during neonatal resuscitation; it is easily located and cannulated, and medication delivery requires insertion only to the point at which blood can be aspirated (usually 2 to 4 cm) (see Chapter 141 Procedures). Vascular access may also be obtained by placing peripheral catheters in the extremities or scalp. IO lines may also be used. A 20- or 22-gauge spinal needle may replace the 16- or 18-gauge larger IO needles; however, the procedure for line placement in the proximal tibia is the same as for older children. Recall that premature infants have a small IO space. Finally, the ET tube may be used for administration of epinephrine when vascular access has not yet been established.
Medications and Volume Expanders for Acute Resuscitation
Epinephrine
Standard dose epinephrine is indicated when the newborn’s heart rate remains less than 60 bpm, despite 30 seconds of effective ventilation with 100% oxygen and another 30 seconds of coordinated chest compressions and ventilation (see Table 4.12 for dosing). It may be administered via an umbilical venous catheter, a peripheral IV, an IO line, or the ET tube and repeated every 3 to 5 minutes. Intravenous epinephrine should be administered as rapidly as possible and followed by a 1-mL normal saline flush. High-dose epinephrine is not recommended and may cause harm.
Volume Expanders
Crystalloid (lactated Ringer or normal saline) is administered intravenously over 5 to 10 minutes in 10 mL per kg aliquots to avoid an increased risk of intracranial hemorrhage. Administration of O-negative red blood cells (cross-matched with mother’s blood if time allows) may be needed for large volume blood loss or poor response to crystalloid infusion. Albumin-containing solutions are not recommended because of cost, limited availability, risk of infection, and potential increased mortality.
Other Medications
ED stabilization should focus on basic resuscitation interventions outlined above. Other medications such as buffers, narcotic antagonist, vasopressors, or surfactant therapy are rarely given in ED phase of care.
Sodium Bicarbonate
Bicarbonate therapy may contribute to respiratory acidosis and a worsening intracellular acidosis, which may actually impair myocardial and cerebral function. In prolonged resuscitations, after establishment of adequate ventilation, bicarbonate may be given to treat documented metabolic acidosis or hyperkalemia using arterial blood gases and serum chemistries to guide administration. Use 0.5 mEq per mL (4.2%, half-strength) solution to decrease effects of hypertonicity. Little research data exist to support the choice of other buffers, such as tris(hydroxymethyl)aminomethane (THAM), for documented metabolic acidosis; practitioners may use this to reduce the occurrence of hypernatremia.
Naloxone Hydrochloride
Naloxone is a narcotic antagonist that reverses narcotic-induced respiratory depression. Naloxone is not recommended for the initial resuscitation attempts in the newly born. The focus of the resuscitation remains the same, providing adequate respiratory support to correct abnormalities in ventilation and circulation. Naloxone given to the newborn of a mother suspected of narcotic addiction may precipitate acute narcotic withdrawal and seizures.
Atropine
Atropine is not recommended for acute neonatal resuscitation as vagal stimulation does not cause bradycardia in neonates. Furthermore, many investigators believe that the vagally mediated bradycardia response to hypoxia is a valuable reflex to guide resuscitative efforts and should not be pharmacologically abolished by atropine.
Glucose
Hypoglycemia in the neonate is associated with increased risk for brain injury and adverse outcomes with hypoxic-ischemic events. Therefore, the administration of IV glucose infusion should be considered as early as is practical after initial resuscitative measures. The goal of glucose infusion should be to maintain euglycemia.
Artificial Pulmonary Surfactant
Newborns who require intubation due to the respiratory distress associated with insufficient surfactant production may benefit from replacement therapy with an artificial surfactant product. Prematurity of less than 35 weeks is the most common indication for surfactant use. Replacement surfactant is delivered to the pulmonary bed via an ET tube. Use of surfactant in the ED should be done in consultation with a neonatologist.
Antibiotics
Chorioamnionitis is usually due to Group B streptococcal or Escheria coli infections. Ampicillin and gentamycin are reasonable empiric antibiotics in a newly born infant with suspected chorioamnionitis (see Chapters 87 Fever in Infants and 102 Infectious Disease Emergencies).
Special Situations
Induced Hypothermia and Hypoxic-ischemic Encephalopathy in Newly Born Infants
Data from large, multicenter, randomized controlled trials have demonstrated that systemic or selective head cooling hypothermia initiated within 6 hours of life, in infants ≥36 weeks reduced death or neurologic disability in infants with moderate or severe hypoxic-ischemic encephalopathy. In a 2012 meta-analysis of 7 studies, induced hypothermia resulted in a 24% decrease in the relative risk of death or major neurodevelopmental disability. The American Academy of Pediatrics Committee on Fetus and Newborn and the AHA guidelines recommend that infants ≥36 weeks’ gestation who have moderate to severe hypoxic encephalopathy be offered therapeutic hypothermia. Currently, hypothermia therapy is not recommended for infants less than 36 weeks’ gestation but clinical trials are ongoing for this age group.
Induced hypothermia should be done at a facility able to provide comprehensive neonatal care, following studied protocols, initiated within 6 hours of birth and continued for 72 hours. For infants born at centers unable to provide such care, early identification of potential candidates and consultation with experts is critical. Table 4.13 defines criteria for moderate to severe encephalopathy. Ideally, medical centers skilled in hypothermia management and community hospitals should develop educational programs and procedures for transfer.
Meconium
Management of meconium-stained amniotic fluid has changed substantially over the last 2 decades. Meconium staining of the amniotic fluid complicates 12% of all pregnancies; the risk of meconium-related complications increases to nearly 30% in infants born after 42 weeks’ gestation. Approximately 2% to 5% of infants born with meconium in the amniotic fluid will experience some degree of aspiration syndrome, ranging from mild tachypnea to very severe pneumonitis with persistent pulmonary hypertension (Fig. 4.18).
TABLE 4.13
INDUCED HYPOTHERMIA FOR NEWBORNS
When meconium staining is detected during an ED delivery, the infant should be immediately transferred to the resuscitation team without intrapartum suctioning of the infant’s mouth and nose (Fig. 4.19). This recommendation is a change from previous guidelines after a multicenter randomized trial showed no decrease in the risk of meconium aspiration syndrome when suctioning was performed after delivery of the infant’s head but before delivery of the body.
Current guidelines for further management of newborns with meconium in the amniotic fluid are based on the status of the newborn rather than the consistency of the meconium. If meconium is present and the infant has depressed respirations, muscle tone, or a heart rate <100 bpm—direct suctioning of the trachea soon after delivery is indicated before many respirations have occurred. After delivery, place the infant in a warm environment, and before other resuscitative efforts, intubate the trachea to facilitate suctioning of the lower airway. Because the ET tube itself is the largest diameter item placed in the trachea, it is the most effective means of suctioning meconium. A meconium aspirator (Fig. 4.20) directly attached between the ET tube and mechanical suction provides a means of meconium removal. Negative pressure is applied by occluding the opening on the side of the aspirator with a finger. Mechanical suctioning should not exceed 100 mm Hg. Repeat intubation and suctioning with another ET tube may be required until the aspirated material is clear. After initial tracheal suctioning, it may be necessary to begin PPV if the heart rate or respirations are severely depressed, despite persistent meconium in the airway. Wait until completion of tracheal suctioning and resuscitation to place an orogastric tube to empty meconium from the newborn’s stomach.
FIGURE 4.18 Meconium aspiration radiograph.
FIGURE 4.19 Management of infant born with meconium-stained amniotic fluid. PPV, positive-pressure ventilation.
When meconium is present and the baby is vigorous (normal respiratory effort and muscle tone, and HR >100 bpm), a bulb syringe or large-bore (12F or 14F) suction catheter is used to clear secretions and any meconium from the mouth and nose. Tracheal suctioning should not be done in vigorous infants, regardless of the consistency of the meconium.
Prematurity
Early involvement of neonatologists and neonatal centers adept in the management of low–birth-weight infants is crucial to improve outcome. Hospitals without neonatal units need easily available guidelines and established relationships for accessing neonatal consultation and transport. Premature infants are at greater risk for bacterial infections, heat loss, inadequate ventilation, and intraventricular hemorrhage. They are more likely to develop respiratory distress and require assisted ventilation. Hyperoxia may lead to complications such as retinopathy of prematurity so beginning the resuscitation with less than 100% inspired oxygen is a reasonable option. If no improvement in heart rate or color occurs by 90 seconds, the fraction of inspired oxygen should be increased. Once the infant is stabilized after initial resuscitative care, the fraction of inspired oxygen can be decreased while monitoring pulse oximetry.
The germinal matrix of the preterm infant’s brain is vulnerable to bleeding. Factors contributing to subsequent intracranial hemorrhage include excessive pressure or osmolality delivered to an already maximally dilated vascular bed. Subsequently, in premature infants, hyperosmolar solutions such as 25% dextrose or 8.4% sodium bicarbonate should be avoided. Volume expanders, dextrose, and half-strength sodium bicarbonate solutions, when indicated, should be administered slowly to minimize injury to these vascular beds.
FIGURE 4.20 Meconium aspirator.
Pneumothorax
Pneumothorax is a potentially lethal problem in the neonate because it can rapidly progress to a tension pneumothorax. It is often the result of PPV, PEEP, or resuscitation. Pneumothorax is also more common in premature infants with surfactant deficiency or meconium aspiration. If significant respiratory distress is present and a pneumothorax is suspected, rapid decompression may be achieved with a large syringe, 20-gauge needle or catheter over needle using a three-way stopcock. The needle is advanced at the fourth intercostal space in the anterior axillary line or the second interspace in the midclavicular line. Subsequently, a chest tube (8F) may be placed using a standard technique (see Chapter 141 Procedures).
Diaphragmatic Hernia
Diaphragmatic hernia is a true neonatal emergency; the diagnosis is confirmed by a chest radiograph showing bowel gas within the thorax (Fig. 4.21). Infants with diaphragmatic hernias require ET intubation to avoid excessive amounts of air accumulation in the bowel. A nasogastric tube should be rapidly placed to decompress the stomach. The infant must be rapidly evaluated by a pediatric surgeon after ventilation is stabilized and venous access is achieved.
FIGURE 4.21 Left diaphragmatic hernia radiograph.
Stabilization and Transport
After appropriate resuscitative efforts, continuous monitoring and anticipation of complications must occur until the patient is safely transported to a neonatal unit or a facility with a neonatal unit. Priority must be given to thermoregulation. If mechanical ventilation is required, a pressure ventilator should be used. Peak pressures are determined by clinical evaluation of adequate chest wall rise and blood gas analyses. A good starting point for peak pressure is that needed for good chest wall rise and breath sounds during hand ventilation as shown on the manometer, usually between 15 and 30 cm H2O. Use the lowest pressure necessary for good clinical response and continue to monitor closely. Excessive positive pressure decreases venous return to the heart and thus cardiac output, and causes injury to lung tissue. The patient should be transported by personnel with appropriate expertise along with appropriate equipment and medications.
TEAMWORK IN RESUSCITATION FOR ALL PEDIATRIC PATIENTS
The new PALS and ACLS curricula have incorporated learning modules on leadership, role clarity, and communication. Effective leaders and team members must have cognitive skills (fund of knowledge), technical and procedural skills, and behavioral skills. Postresuscitation team debriefing is increasingly recognized as a critical component in maintaining effective teamwork and communication skills in pediatric resuscitations. Recent information reveals lack of effective teamwork skills and their negative impact on outcomes. Effective education includes challenging active exercises, such as high-fidelity simulation. Video-recording of resuscitation provides another venue for identifying opportunities for improving care processes and for providing constructive feedback on team management skills. Maintenance of competency for physicians working in ED settings includes procedural competency and leadership competency. Developing and maintaining the ability to effectively lead a multidisciplinary team in a high-stakes, high-risk, error-prone environment is necessary and must be thoughtfully considered in this era of decreasing frequency of individual exposure to these patients.
Finally, parental presence in the resuscitation room is recommended by the AAP and should be routine practice. All EDs should have a written policy and a process in place; and all families should be offered this opportunity (see Chapter 1 A General Approach to Ill and Injured Children).
DISCONTINUATION OF LIFE SUPPORT IN CHILDREN
If well-executed resuscitative measures fail to achieve ROSC, resuscitative efforts should be discontinued. There is now good evidence to support that there is no chance for meaningful survival in patients who remain unresponsive to aggressive airway intervention, chest compressions, and two doses of epinephrine after unwitnessed cardiac arrest. Thus, a brief, well-executed resuscitation is indicated for the child who arrives to the ED with cardiopulmonary arrest. This includes definitive airway management; vigorous, monitored chest compressions; IO access; and one to two doses of epinephrine. During this time, the leader of the resuscitation can review the history and complete the primary and secondary survey. Prolonged resuscitation efforts past 20 minutes, without ROSC, are usually futile unless other treatable problems exist such as hypothermia, drug overdose, or VT/VF. Prolonged resuscitation may be indicated for witnessed collapsed arrest, with short onset of effective BLS/ACLS, especially if a cardiac etiology is suspected. Ultimately, the diagnosis of death and subsequent discontinuation of resuscitative efforts is a judgment that is made by the team leader in conjunction with the team. A decision not to begin resuscitation is generally not made in the ED unless there is a written do-not-resuscitate (DNR) document provided by the child’s parent or guardian.
A well-prepared ED should consider and have a plan in place for issues such as advanced directives, palliative care, bereavement measures and postmortem care, survivor follow-up, and request for autopsy and organ donations as outlined in the recent AAP guidelines. Proper documentation of a death is essential, as is notification of medical legal authorities, donor programs, and referring physicians and consultants.
CEREBRAL RESUSCITATION
Permanent brain damage following arrest is determined by many factors and includes arrest time (no-flow state), CPR time (low-flow state), and temperature. Cardiopulmonary–cerebral resuscitation is needed to prevent brain injury. Oxygen stores are depleted within 20 seconds following arrest, and glucose and adenosine are depleted within 5 minutes. During no-flow states, multiple complex chemical derangements occur that contribute to the death of neurons. With ROSC, there is impaired cerebral blood flow. Circulatory and pharmacologic interventions to prevent postanoxic brain injury have yielded disappointing results to date.
Hypothermia
The use of mild hypothermia after OHCA due to VF in adults has been associated with improved neurologic outcome and is generally tolerated without significant complication. Current AHA recommendations are to begin therapeutic hypothermia for all adult patients after VT/VF arrest with ROSC to a goal temperature of 32 to 34°C for 12 to 24 hours. The AHA also recommends the use of therapeutic hypothermia in adult patients with ROSC after arrest from other nonshockable rhythms. Data from large, multicenter, randomized controlled trials have demonstrated that therapeutic hyphothermia for asphyxiated newly born infants ≥36 weeks’ gestation can reduce death and neurologic disability when initiated within 6 hours of birth. AHA guidelines recommend that all such infants with moderate to severe hypoxic-ischemic encephalopathy be offered therapeutic hypothermia.
However, the data for pediatric patients is less clear. Current AHA guidelines state that therapeutic hypothermia may be considered for children who remain comatose after return of spontaneous circulation following cardiac arrest to a level of 32° to 34°C. It is most reasonable to consider for adolescent patients who are resuscitated from a sudden witnessed VT/VF arrest. Fever adversely affects recovery from ischemic brain injury, and should be treated aggressively; avoiding T >38°C is recommended. A large, multicenter, randomized controlled trial of therapeutic hypothermia after out-of-hospital arrest (published after the most recent AHA guidelines) comparing therapeutic hypothermia to therapeutic normothermia found no difference on survival with good functional outcome.
QUALITY IMPROVEMENT
EDs represent a high-risk environment for the medical care of patients due to factors such as clinical uncertainty, frequent interruptions, and the need for haste. Children are at particular risk in emergency care because of their physical and developmental vulnerabilities, their inability to accurately describe symptoms or past medical history, the complexity of weight-based treatment, and the relative discomfort of some providers in treating pediatric patients. This risk is particularly heightened during emergency resuscitation, which is a team-dependent and information-intensive process of rapidly treating acute life-threatening and organ-threatening diseases. The medical resuscitation environment is especially prone to medical errors due to its fast-paced, complex and information-technology poor environment. Therefore, ongoing surveillance of resuscitation events, with an eye to process and system changes which could support the resuscitation team, minimize distraction from patient care and maximize protocol adherence is vital. A video review process in which all resuscitation events are video recorded, and a subset are reviewed in detail by an open, multidisciplinary committee provides an opportunity to improve. The review process focuses on time to critical events (completion of primary/secondary survey, vascular access, fluid/med administration, etc.), adherence to expected protocols, teamwork measures (including closed loop communication, ambient noise, anticipation of medications and equipment, etc.), specific role performance, and identification of barriers and facilitators to care. Specific resuscitation events are chosen for review through a combination of provider request and identification of the highest-risk scenarios, including the most complex and least common events. Process and system issues, educational deficits, and other barriers to protocol-compliant care identified through the video review process are addressed on an ongoing basis. Interventions to improve quality of care in the resuscitation room can include changes in the physical resources available, such as medications, fluids and equipment, or personnel available. For example, movement of the EZ-IO introducer and needles to the bed during room preparation prior to patient arrival can decrease the time to vascular access. Assigning a second senior physician to supervise CPR and other procedures in resuscitation events requiring CPR, can decrease the burden on the leader physician and increase protocol compliance with CPR. Other interventions to improve care may include simulated resuscitative events to allow for practice of uncommon events. Multidisciplinary simulations that occur in the actual resuscitation environment are most likely to maximize learning and may identify latent barriers to care. In addition, participation in video review, itself, allows for participants to experience patient resuscitations that simulate cognitive decision making for the viewer. Finally, intermittent group review of important findings from the quality improvement efforts, targeted group education modules and personal feedback on given resuscitation events can support improvements in provider behavior to maximize protocol adherence and decrease variability in resuscitation care. It is important that EDs identify a feasible means to monitor resuscitation care and provide ongoing local quality improvement to insure that optimal care is provided in this high-stakes, error-prone environment.
ETHICAL ISSUES IN PEDIATRIC CARDIOPULMONARY RESUSCITATION
There are many ethical issues surrounding pediatric resuscitation: When are resuscitation attempts futile? Is the ED physician obligated to provide care at the families’ insistence? How do family religious beliefs play a role in decision making? What is the role of parental presence? Should procedures be performed on the recently dead? Can resuscitation research be performed without informed consent? Some of these issues have been addressed in policy statements made by professional organizations, but each question needs to be considered in discussions that occur at the local ED level.
In response to these varied, complex, and often highly charged issues, postresuscitation debriefing has become a vital component of the pediatric resuscitation. Consider taking a few minutes for critical reflection following the completion of the resuscitation event; this has the potential to enhance teamwork and communication, and provides an opportunity to improve future performance through group reflection on the shared experience.
KEY POINTS
The vast majority of pediatric cardiac arrests are asphyxia and both survival and neurologic outcome is poor.
Recognition of impending respiratory and circulatory failure and immediate intervention can be truly life-saving.
VT/VF is estimated to occur in approximately 10% of OHCA.
IO is the preferred access for arrested patients, and patients with severe hypotension or other severely ill patients where attempt at IV access is prolonged.
Airway management and high-quality chest compressions are the key resuscitation interventions.
All EDs should have program for continuous quality improvements around the care of critically ill children.
Strong leadership and teamwork with closed loop communication is essential.
EDs should have practice simulations and skills sessions to assure competency in knowledge and critical resuscitation skills.
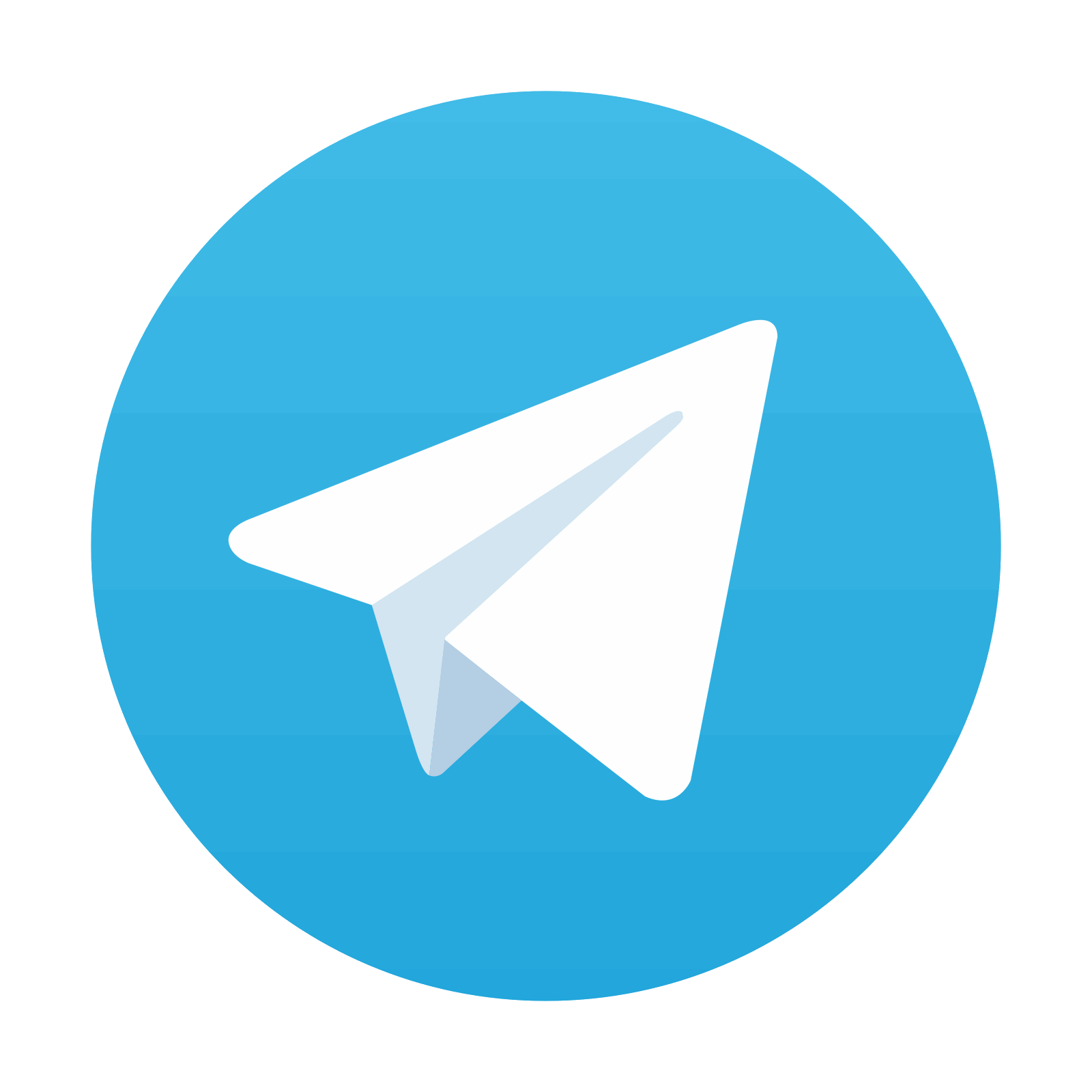
Stay updated, free articles. Join our Telegram channel

Full access? Get Clinical Tree
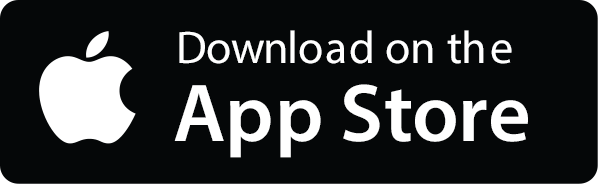
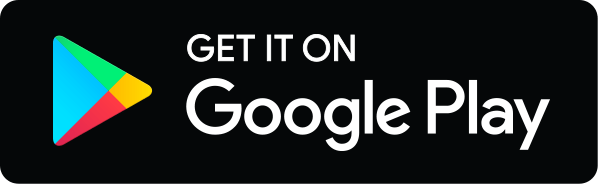