Two-dimensional glycocalyx–cleft model of capillary fluid exchange: the sub-glycocalyx space is protected and is protein free, forming the basis for the “no-reabsorption rule.” The glycocalyx–cleft model identifies glycocalyx as a semipermeable layer. Its underside is subjected to the colloid osmotic pressure of fluid high inside the intercellular cleft rather than interstitial fluid (ISF), with important functional consequences. Pc, plasma capillary hydrostatic pressure; Pi, interstitial hydrostatic pressure; πc, oncotic pressure of plasma; πi, oncotic pressure of interstitial fluid; πg, oncotic pressure of intercellular endothelial cleft; EC, endothelial cell.
Types of resuscitation fluids
Colloids
Human albumin solutions
In healthy humans, albumin contributes 80% of the plasma oncotic pressure (25 mmHg) whereas in critically ill patients there is considerable reduction (to approx. 19 mmHg) and associated poor outcomes.[17] Albumin is involved in transport and metabolism of bile acids, eicosanoids, copper, zinc, folate and aquacobalamin, and drugs. Albumin imparts half of the normal anion gap, has significant antioxidant properties, and maintains vascular nitric oxide (NO) levels via stable S-nitrosothiol. Human albumin solutions (HAS) are produced by fractionation of blood and then heat treated to prevent transmission of viruses. The Saline versus Albumin Fluid Evaluation study (SAFE trial), on 6,997 patients randomized to either albumin or saline, concluded that albumin was not superior to saline when analyzed for 28-day mortality (relative risk, 0.99: 95% confidence interval, 0.91–1.09, p = 0.870).[18] Since then, follow-on trials such as FEAST study and ALBIOS have not shown any superiority of human albumin over crystalloids.[19,20]
Semisynthetic colloids
Hydroxyethyl starches (HES)
The high cost of human albumin dictated the development of synthetic colloids. Currently HES are the most commonly used synthetic colloids (Table 22.1). HES are produced by hydroxyl substitution of amylopectin obtained from sorghum, maize, or potatoes. A high degree of substitution on glucose molecules protects against hydrolysis by non-specific amylases in the blood, but the action increases accumulation in liver and spleen macrophages, which constitute the reticuloendothelial system, probably because the reticuloendothelial system recognizes HES as a foreign substance and stores it (in the liver, spleen, and skin).[21] HES administration causes changes in coagulation and fibrinolysis.[22] The effect on fibrinolysis is driven by reduction in alpha2-antiplasmin/plasmin interactions with simultaneous reduction in all measures of coagulation such as clotting time, maximum clot firmness, and alpha-angle; these changes are driven by platelet dysfunction and loss of coagulation factors.[22,23] Growing evidence now questions the safety of traditional and modern HES owing to an increased incidence of mortality and renal failure observed in large randomized controlled trials (RCTs). However, these trials have only focused on critically ill septic patients, and have ignored trauma or cardiac surgical populations. Currently used HESs have a reduced concentration (6%) with a molecular weight (MW) of 130 kDa and a substitution of 0.38–0.45. HES is widely used for patients undergoing anesthesia for major surgery and in patients in ICU.
Preparationa | Concentration | Trade name | MMW (kDa) | Specification range (kDa) | Top fractionb (kDa) | Bottom fractionc (kDa) | MS | C2/C6 ratio | In vitro COP (mmHg) | Initial volume effect | T½alpha (h) | T½beta (h) | Clearance (ml/min) |
---|---|---|---|---|---|---|---|---|---|---|---|---|---|
Hetastarch | |||||||||||||
HES 450/0.7 | 6% | Plasmasteril, Hespan | 450 | 150 | 2,170 | 19 | 0.7 | 4–5 | 26 | 100 | n/a | 300 | n/a |
HES 670/0.7 | 6% | Hextend | 670 | 175 | 2,500 | 20 | 0.75 | 4 | n/a | 100 | 6.3 | 46.4 | 0.98 |
Hexastarch | |||||||||||||
HES 200/0.62 | 6% | Elohes | 200 | 25 | 900 | 15 | 0.62 | 9 | 25 | 110 | 5.08 | 69.7 | 1.23 |
Pentastarch | |||||||||||||
HES 70/0.5 | 6% | Rheohes, Expafusin | 70 | 10 | 180 | 7 | 0.5 | 3 | 30 | 90 | n/a | n/a | n/a |
HES 200/0.5 | 10% | HAES-steril, Hemohes | 200 | 50 | 780 | 13 | 0.5 | 4–5 | 50–60 | 145 | 3.35 | 30.6 | 9.24 |
HES200/0.5 | 6% | HAES-steril, Hemohes | 200 | 50 | 780 | 13 | 0.5 | 4–5 | 30–35 | 100 | n/a | n/a | n/a |
HES 200/0.5 | 3% | HAES-steril, Hemohes | 200 | 50 | 780 | 13 | 0.5 | 4–5 | 15–18 | 60 | n/a | n/a | n/a |
Tetrastarch (waxy-maize-derived) | |||||||||||||
HES 130/0.4 | 10% | Voluven | 130 | 20 | 380 | 15 | 0.4 | 9 | 70–80 | 200 | 1.54 | 12.8 | 26 |
HES 130/0.4 | 6% | Voluven, Volulyte | 130 | 20 | 380 | 15 | 0.4 | 9 | 36 | 100 | 1.39 | 12.1 | 31.4 |
Tetrastarch (potato-derived) | |||||||||||||
HES 130/0.42 | 10% | Tetraspan | 130 | 15 | n/a | n/a | 0.42 | 6 | 60 | 150 | n/a | n/a | n/a |
HES 130/0.42 | 6% | Venofundin, Tetraspan, VitaHES | 130 | 15 | n/a | n/a | 0.42 | 6 | 36 | 100 | n/a | 12 | 19 |
Derived and modified from Ertmer et al. [24].
a Data extracted from manufacturer’s product information.
b Bottom fraction: <10% of molecules are less than the molecular weight defined by bottom fraction.
c Top fraction: <10% of molecules exceed the molecular weight defined by bottom fraction.
COP, colloid osmotic pressure; MMW, molecular weight; MS, molar substitution; n/a, not applicable or not available; T½ alpha, distribution half-life; T½ beta, elimination half-life.
The raw material from which HES is synthesized is amylopectin (starch). HES is generated by nucleophilic substitution of amylopectin to ethylene oxide in the presence of an alkaline catalyst. Residual solvents are removed by repeated ultrafiltration. HES with a molar substitution of 0.5 or 0.6 is denoted as “pentastarch” or “hexastarch,” respectively. In this regard, first-generation HESs consist of heta- and hexa-starches, whereas pentastarch is allocated to the second generation. The latest, third-generation HESs consist of modern tetrastarches (HES 130/0.4 and HES 130/0.42).
Gelatins
These are prepared by hydrolysis of bovine collagen. Succinylated gelatin (Gelofusine™) is produced by enzymatic alterations of the basic gelatin peptide and is presented in isotonic saline. Urea-linked gelatin (Polygeline, Haemaccel™) is produced by thermal degradation of the raw material to small peptides (12,000–15,000 Da) followed by urea cross-linking to produce polymers of around 35,000 Da that are suspended in isotonic sodium chloride with 5.1 mmol/l potassium and 6.25 mmol/l calcium.[24] Concerns have been raised about association between the use of bovine-derived gelatin (but not pharmaceutically derived), bovine spongiform encephalitis, and Creutzfeld–Jakob disease.[24]
Dextrans
These are biosynthesized from sucrose by Leuconostoc bacteria using the enzyme dextran sucrase. This enzyme catalyzes the alpha-1,6-glycosidic linkage of glucose monomers. Dextrans are defined by their MW, with dextran 40 and 70 having MW 40,000 and 70,000 Da, respectively. Allergic reactions (<0.35%) have been associated with dextrans.[5] Injection of a hapten, dextran 1, before administering dextran solutions has significantly reduced the incidence to <0.0015%.[25] This involves a 20 ml injection of low-MW dextran 1 (1,000 Da MW) prior to infusion of a dextran volume expander, which leads to inactivation of anti-dextran IgGs in the recipient.
Crystalloids
The most commonly used crystalloid is normal (0.9%) saline (Na concentration = 154 mmol/l). The term “normal” was coined by Dutch physiologist Hartog Jacob Hamburger, who in 1882 suggested human blood salt to be 0.9% rather than 0.6%.
Concerns have been raised that normal saline (NS) induces hyperchloremic metabolic acidosis (NaCl + H2O ➔ HCl+ NaOH), but the clinical consequences of this are unclear.[26] In addition, owing to concerns regarding a fluid overload condition induced by large volumes, small-volume resuscitation with hypertonic saline (3%, 5%, or 7%) has been introduced.
Hypertonic saline
In 1980, de Felippe reported almost miraculous recovery from near-fatal hemorrhagic shock in 11 patients resuscitated with hypertonic saline (HS).[27] Various concentrations of HS have been used, and these are provided in Table 22.2. The plasma volume expansion is derived from increased fluid shift ability, secondary to the hyperosmotic and hyperoncotic abilities of these solutions. The plasma volume expansion is approximately 750 ml for infusion of 1 liter of HS vs. 300 ml derived from 1 liter of crystalloid. Hypertonicity leads to vasodilatation by improving endothelial cell volume, improves cardiac output, and has also shown to reduce neutrophilic activation.[28] However, HS have not improved outcomes in patients with traumatic brain injury.[29]
Solution | Osmolarity (mosmol/l) | Sodium concentration (mosmol/l) |
---|---|---|
0.9% normal saline | 308 | 154 |
Ringer’s lactate | 275 | 130 |
1.7% saline | 291 | 582 |
3% saline | 1,026 | 513 |
7.2% saline/6% HAES (200/0.6) | 2,464 | 1,232 |
7.5% saline | 2,566 | 1,283 |
7.5% saline/6% dextran 70 | 2,568 | 1,283 |
10% saline | 3,424 | 1,712 |
23% saline | 8,008 | 4,004 |
30% saline | 10,000 | 5,000 |
Derived from Strandvik.[28]
Physiologically “balanced” and “unbalanced” fluids
Balanced or physiological fluids contain inorganic ions (calcium, potassium, magnesium), molecular glucose, and buffer components such as lactate or bicarbonate, and have a lower chloride component to prevent hyperchloremic metabolic acidosis. There is evidence that patients randomized to these vs. unbalanced fluids had less impaired coagulation (less effect on thromboelastography and platelet aggregation).[30] Renal function may also be better preserved.
A typical example of balanced crystalloids is Hartmann’s solution/Ringer’s lactate. Balanced colloids are also available as 6% HES (Hextend®, Volulyte®). Table 22.3 summarizes the advantages and disadvantages of colloids and crystalloids, while Table 22.4 details compositions of commonly used colloids and crystalloids.
Solution | Advantages | Disadvantages |
---|---|---|
Colloids | 1. Smaller infused volumes 2. Prolong increase in plasma volumes 3. Less peripheral edema 4. Endothelial protection | 1. Renal dysfunction (dextran>HES>albumin) 2. Coagulopathy (older HES>tetrastarch>albumin) 3. Pulmonary edema (capillary leak syndrome) 4. Pruritus (HES, dextran>albumin) 5. Anaphylaxis (dextran>HES>albumin) 6. Greater cost (albumin>other synthetic colloids) |
Crystalloids | 1. Lower cost 2. Higher glomerular filtration rate 3. Interstitial fluid replacement | 1. Short-term increase in intravascular volume 2. Short-term hemodynamic improvement 3. Interstitial fluid accumulation |
Variable | Human plasma | 4% albumin | 10% (200/0.5) HES | 6% (450/0.7) HES | 6% (130/0.4) HES | 6% (130/0.42) HES | 4% succinylated modified fluid gelatin | 3.5% urealinked gelatin | 0.9% saline | Compounded sodium lactate | Balanced salt solution | ||
---|---|---|---|---|---|---|---|---|---|---|---|---|---|
Trade name | Albumex | Hemohes | Hextend | Voluven | Volulyte | Venofundin | Tetraspan | Gelofusine | Haemaccel | Normal saline | Hartmann’s or Ringer’s lactate | Plasma-Lyte | |
Colloid source | Human donor | Potato starch | Maize starch | Maize starch | Maize starch | Potato starch | Potato starch | Bovine gelatin | Bovine gelatin | ||||
Osmolarity (mosmol/l) | 291 | 250 | 308 | 304 | 308 | 286 | 308 | 296 | 274 | 301 | 308 | 280.6 | 294 |
Sodiuma | 135–145 | 148 | 154 | 143 | 154 | 137 | 154 | 140 | 154 | 145 | 154 | 131 | 140 |
Potassiuma | 4.5–5.0 | – | – | 3.0 | – | 4 | – | 4 | – | 5.1 | – | 5.4 | 5 |
Calciuma | 2.2–2.6 | – | – | 5 | – | – | – | 2.5 | – | 6.25 | – | 2 | – |
Magnesiuma | 0.8–1 | – | – | 0.9 | – | 1.5 | – | 1 | – | – | – | – | 3 |
Chloridea | 94–111 | 128 | 154 | 124 | 154 | 110 | 154 | 118 | 120 | 145 | 154 | 111 | 98 |
Acetatea | – | – | – | – | – | 34 | – | 24 | – | – | – | – | 27 |
Lactatea | 1–2 | – | – | 28 | – | – | – | – | – | – | – | 29 | – |
Malatea | – | – | – | – | – | – | – | 5 | – | – | – | – | – |
Gluconatea | – | – | – | – | – | – | – | – | – | – | – | – | 23 |
Bicarbonatea | 23–37 | – | – | – | – | – | – | – | – | – | – | – | – |
Octanoatea | – | 6.4 | – | – | – | – | – | – | – | – | – | – | – |
Derived from Myburgh et al. [31].
a Units of measurement are mmol/l.
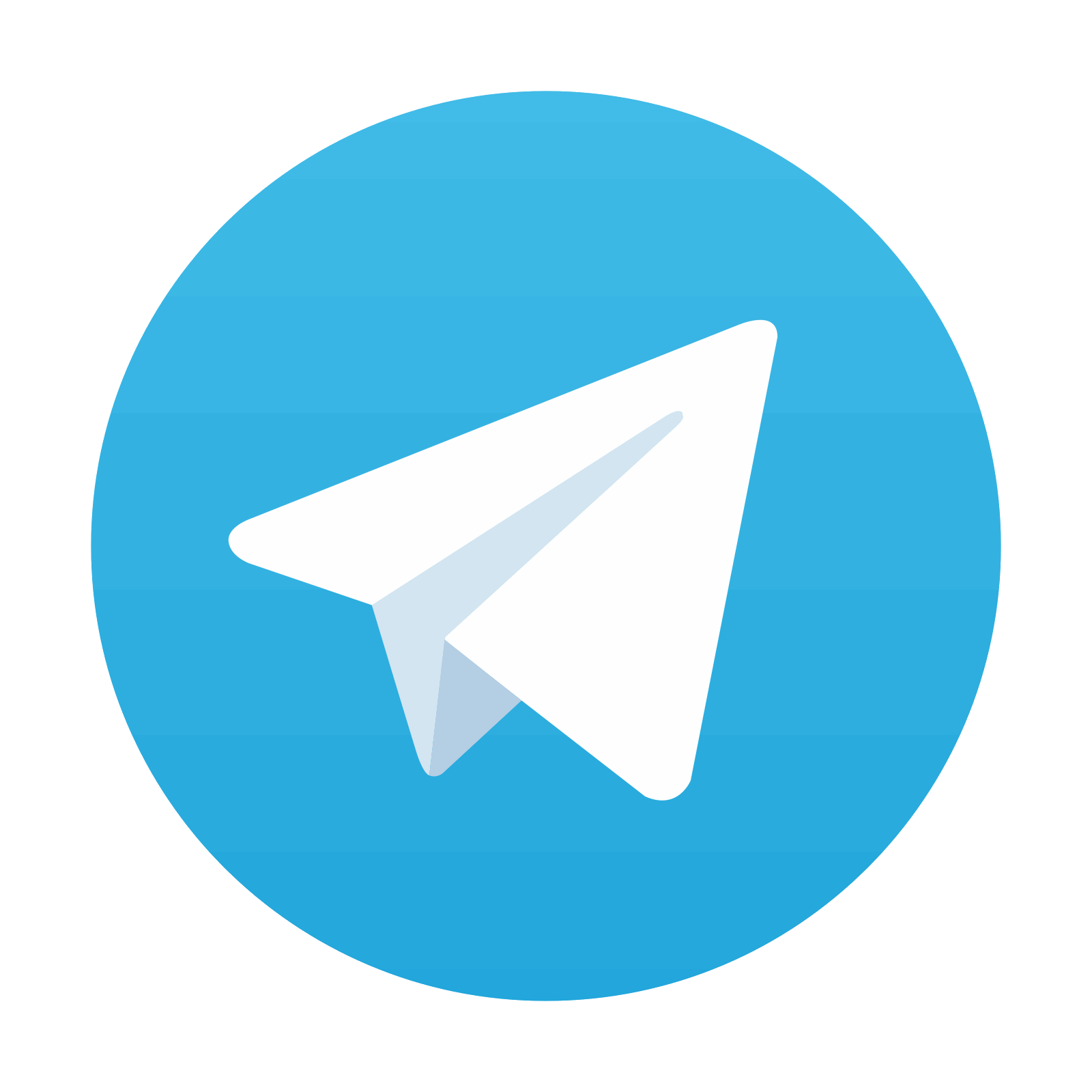
Stay updated, free articles. Join our Telegram channel

Full access? Get Clinical Tree
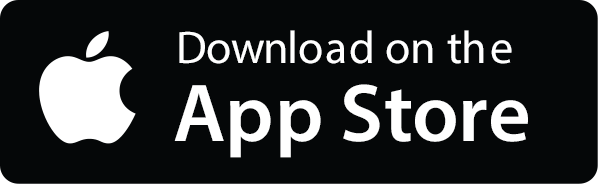
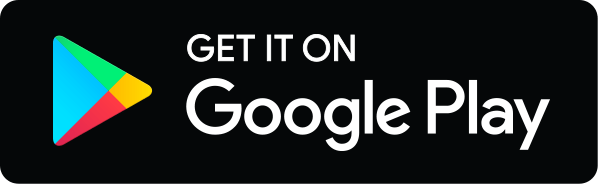
