Fig. 51.1
Schematic diagram of the gate control theory of pain mechanisms: L the large-diameter fibers, S the small-diameter fibers. The fibers project to the substantia gelatinosa (SG) and first central transmission (T) cells. The inhibitory effect exerted by SG on the afferent fiber terminals is increased by activity in L fibers and decreased by activity in S fibers. The central control trigger is represented by a line running from the large-fiber system to the central control mechanisms: these mechanisms, in turn, project back to the gate control system. The T cells project to the entry cells of the action system. + excitation, − inhibition [3]
Based on this theory , a new technological application was then forged by the neurosurgeon C. Normal Shealy. Dr. Shealy extrapolated that most chronic pain signals projected from diffusely organized pain fibers in the periphery and concentrated their branches with the larger diameter A fibers upon entering spinal cord. Using the ideas from the Gate Control Theory, he applied electrodes to the spinal cord to focus on stimulating the dorsal columns, where the large fibers were also compactly arranged and could induce an “electronarcosis .” [5, 6] When a pulsed D.C. current of 2 mamps, at 0.3 volts, programmed at 50 cycles per second, was applied to a dorsal column electrode over the cervical cord, animals could be awake and could tolerate noxious stimuli, such as tail pinching or intense heat applied to their ears, with no apparent distress [5, 6].
Later research found out just how important the A fibers were in the pathology of medically intractable neuropathic pain. Researchers found that, in the absence of the large A fibers, the small unmyelinated C fibers started to fire spontaneously in a burst pattern or in a series of rapid action potentials, followed by a period of quiescence [7]. Thus, without the inhibitory presence of the A fibers, the spontaneous firing of the C fibers produced hyperalgesia, which subsequently lead to the development of neuropathic pai n. Therefore, by activating these A fibers, as with spinal cord stimulation, the pain generating activity of the C fibers could be overpowered, which would result in analgesia [8]. Electrical stimulation is essential, as these A fibers are normally inactive at baseline.
Neuropathic pain is very well treated using spinal cord stimulation. Conditions that lead to neuropathic pain include diabetic neuropathy, failed back surgery syndrome, complex regional pain syndrome, ischemic limb pain, post-herpetic neuralgia, acute herpes zoster pain, refractory angina, and abdominal pain resulting from chronic pancreatitis [9]. The mechanism of spinal cord stimulation treatment is still not well understood, but it seems to involve a combination of local spinal and supra-spinal mechanisms. At the spinal level, the ascending dorsal column fibers, as well as the descending opioidergic and serotoninergic [10] pain modulatory systems, might be implicated in the pain-suppressing effect [11]. Spinal cord stimulation is also associated with enhanced gamma aminobutyric acid, acetylcholine release, and reduced glutamate transmission in the dorsal horn [12].
To achieve pain relief for these painful conditions, factors such as the configuration of active electrodes, the stimulation frequency, pulse width, and pulse amplitude are adjusted to the patient’s needs. The electrical stimulation of the large-diameter fibers in the dorsal columns elicits tingling sensations (paresthesias) in most patients. These paresthesia perceptions vary greatly among patients and can be the source of consternation. Some patients reject the device based on these paresthesias and others prefer to feel them so that they know their pain is being treated [13].
After a trial of the implanted electrodes has shown that a patient’s pain will be significantly reduced and that the patient’s function will be subsequently increased with the epidural lead configuration and amount of electrical energy required, an implantable pulse generator (IPG) is placed. The generator utilizes either a constant current (CC) or a constant voltage (CV) power source. A CC source supplies current to the tissue by adjusting the voltage, in response to impedance resulting from lead positioning, the presence of fibrous encapsulation, and the presence of scar tissue [14]. A CV source adjusts current in response to impedance, thereby maintaining a constant voltage. These changes in impedance will impact stimulation strength during a stimulus pulse, as well as the efficacy of stimulation over the long term [15]. Both systems produce paresthesia and both systems have been shown to effectively treat chronic pain. However, recent evidence suggests that patients prefer CC over CV systems. In a recent study, patients previously implanted with a CV spinal cord stimulation (SCS) system for chronic pain of the trunk or limbs were switched to a CC system, and patient preference was then assessed [9]. Nearly all patients preferred CC stimulation, describing it as more comfortable and noting it to provide better pain relief.
Why patients prefer CC over CV stimulation remains unresolved. One thought is that the pulse shape generated by CV is spiked-shaped and steepens with the rise of impedance at the beginning of the pulse. CC sources produce a smooth, rectangular-shaped voltage pulse in response to increased impedance (see Fig. 51.2). These subtle changes in waveforms may selectively activate nerve fibers of varying diameters under specific conditions [16]. For example, spiked-shaped pulses have been shown to selectively activate small myelinated fibers, as well as un-myelinated C fibers, without activation of the larger Aβ fibers [9]. So, large spiked pulses, generated in response to high impedance, may not be tolerable, as they are activating some of C and A-delta fibers, which could result in painful stimulation at the beginning of the pulse.
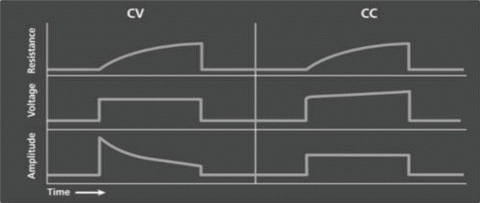
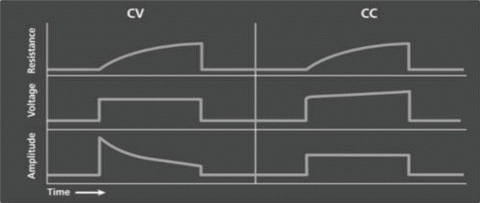
Fig. 51.2
Constant voltage (CV) and constant current (CC) pulse shapes in response to residence
Pulse shape is one factor that determines nerve fiber response to SCS. Another essential factor is the frequency of the pulses used to activate the large fibers in the dorsal columns. The frequencies of SCS impulses that are most often used are usually in the range of 50 Hz but can vary between 30 and 120 Hz. New types of stimulation paradigms for SCS have used high-frequency stimulation up to 10 kHz [17]. The 10 kHz setting is a very energy demanding form of stimulation, which taxes the IPG battery life, requiring frequent charging of the device. An ideal alternative would be a stimulation paradigm that combined elements of high-frequency stimulation with the less energy demanding requirements of tonic stimulation. Burst stimulation offers a more concise signal transmission, resulting in a waveform that allows for passive discharge during the recovery phase between each pulse within the burst pulse train, and between each group of burst pulse trains. This differs from cycling, as cycling requires an active discharge in the recovery phase. The DeRidder burst waveform uses pulse trains of five high-frequency spike pulses at 500 Hz, occurring 40 times per second [13].
Burst stimulation mirrors some of the neuronal firing patterns in the spinal cord. These neurons fire in groups of action potentials, followed by periods of quiescence, just like the burst program generated by the IPG. Other neurons, at the same stage of sensory processing, fire in a tonic or continuous manner. These neuronal languages are transmitted as firing patterns and allow communication from the spinal cord to the brain. To intervene effectively, an SCS device should speak the same language. The experimental data extracted from laboratory and clinical studies suggests that both bursting and tonically firing neurons efficiently transmit information to the thalamus (Fig. 51.3) [18, 19]. The laboratory animal studies suggest that burst firing is more powerful than tonic firing in activating the cerebral cortex [20]. These studies have been interpreted as showing that burst activation requires less temporal integration and may activate dormant neurons, not otherwise activated by tonic stimulation [21].
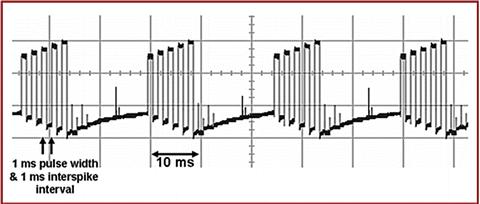
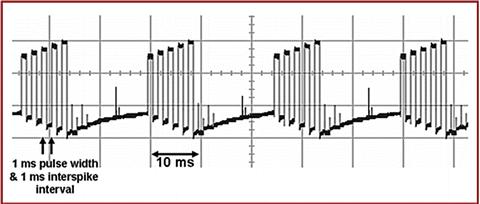
Fig. 51.3
Constant current burst mode (mA): 1-ms spikes with a 1-ms spike interval (500-Hz spike mode) and 5-ms charge balance firing at 40 Hz (40-Hz burst mode). Stimulation delivered by the Eon Implantable Pulse General (Advanced Neuromodulation Systems, Inc., Plano, Texas) via a custom-made program [18]
Evidence
When applied to the spinal cord, burst stimulation delivers paresthesia-free stimulation, which some patients find to be more comfortable, as opposed to the vibrations felt during tonic stimulation. The landmark clinical trial, performed in the United States, identified a place for both the paresthesia and the paresthesia-free modality. In addition, this finding allowed for the capability to design double-blind, placebo-controlled studies of this burst pattern, in order to test its clinical effectiveness. The lack of paresthesia meant that patients and researchers would be unable to tell when the IPG was active, allowing for analysis between placebo and burst stimulation [18]. Studies can now be designed to test the hypothesis that the more physiologic burst firing pattern treats neuropathic pain more effectively than tonic firing patterns [8].
One study examined 48 patients, with at least 6 months of conventional tonic stimulation, and changed their IPG programming to burst stimulation for a period of 2 weeks. They were classified into three different groups: one cross-section of patients with painful diabetic neuropathy (PDN) , a cross-section of failed back surgery syndrome (FBSS) patients, and finally, a cross section of FBSS patients who had become poor responders (PR) to SCS. Visual analog scale scores for pain were assessed prior to implantation with tonic stimulation, and after 2 weeks of burst stimulation. The results of this study showed that burst stimulation caused pain reduction in almost all patients. On average, burst stimulation lead to greater pain reduction in all three patient groups, as compared to tonic stimulation. In total, about 60% of patients (67% for PDN, 58% for FBSS, and 50% for the PR group) experienced further pain reduction when applying burst stimulation, as compared to tonic stimulation. An increase in perceived pain reduction with burst stimulation started after 1–7 days (Fig. 51.4) [13].
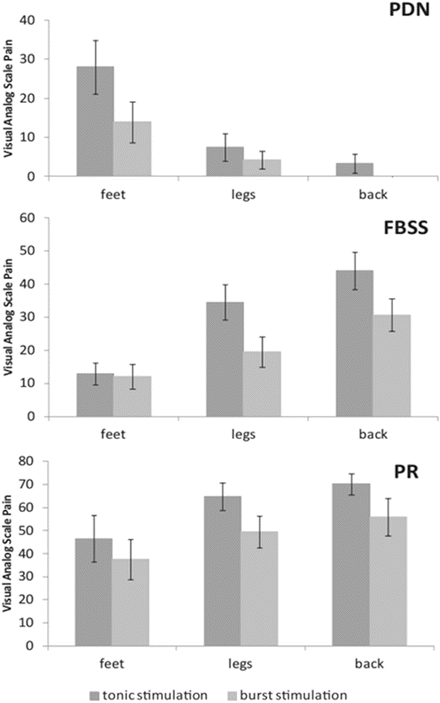
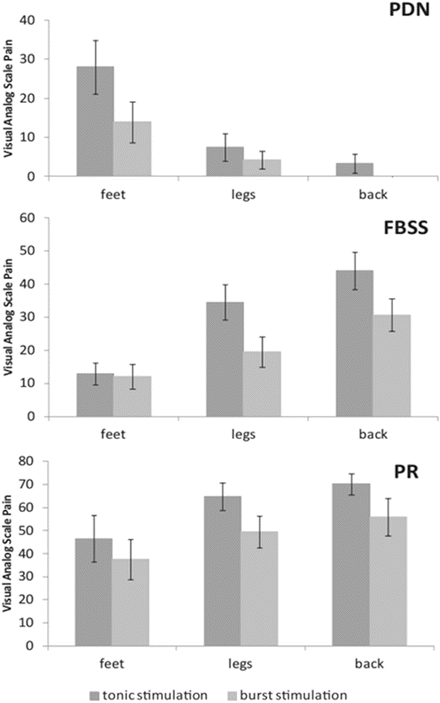
Fig. 51.4
VAS scores for pain of the patients with painful diabetic neuropathy (PDN) , failed back surgery syndrome (FBSS) , and the poor responders (PR) perceived in their feet, legs, and back, with tonic and burst stimulation. Bars represent the average pain score in a body part; error bars represent standard errors [13]
In addition to the spinal cord, the thalamus communicates to the cortex in a burst firing pattern [22]. Burst generated activity from the SCS mimics this thalamocortical firing pattern. The activation of specific regions of the thalamus by the burst stimulation initiated in the spinal cord appears to exploit another pathway, which further reduces the patient’s affective response to pain. This hypothesis is supported by electroencephalogram recordings made during placebo, burst, and tonic stimulation [23]. This effect takes time to reach its full potential, as seen in examinations during a multi-week trial between burst and tonic stimulation. One study found that in a 1-week trial, burst stimulation was no better than tonic stimulation for leg and back pain; however, over a longer period, burst stimulation was superior to tonic for pain suppression, which was possibly due to the affective mechanism [24].
Burst stimulation seems to have a dramatically different effect on the attention paid to pain and pain changes, analogous to the effect of a cingulotomy [25]. It is known that attention to pain is mediated via the anterior cingulate cortex. In one study, the use of functional magnetic resonance imaging (fMRI) , which was performed during SCS, has demonstrated that tonic stimulation modulates, predominantly, the lateral pain system, which includes the primary sensorimotor area, the posterior insula, and the secondary somatosensory cortex [26]. Burst stimulation activates not only the lateral pathway but also the medial pain pathway transmission system, which stimulates the cingulate cortex. Based on these results, it can be hypothesized that burst stimulation not only modulates the lateral discriminatory pain system but also the medial affective/attentional pain system. The mechanisms of pain suppression during burst stimulation combine to allow more patients to find relief when tonic stimulation has failed.
Another study has shown that burst stimulation can rescue about 60% of SCS failure patients who do not respond to tonic stimulation [24]. This predicts yet another benefit of burst stimulation, in that it may be better at improving long-standing pain when tonic SCS becomes dramatically less effective over time [27]. The results from this study by De Ridder et al. suggest that there is no reason to exclude patients with long-term pain from a trial of SCS. Indeed, some patients who had suffered pain for over 20 years prior to implantation had very good pain suppression effects with burst stimulation. Further analysis demonstrated that burst stimulation was superior to tonic stimulation , irrespective of how long the patients experienced pain prior to implantation. This shows that modulating the affective and attentional component of pain, via the medial pain pathway, can bring relief to those with decades of pain. De Ridder’s study demonstrated that even though patients were only stimulated for 2 weeks, this duration was long enough to permit a large degree of pain suppression [8].
There are other important factors that predict successful outcomes with burst or tonic stimulation, such as selecting the patients most likely to benefit. There are certain patient characteristics, which make successful pain reduction more likely, and others that predict a less favorable outcome. First, a neuromodulation provider must be sure to establish realistic goals and patient expectations regarding treatment. The patient must understand that success with SCS is typically defined as a 50% or greater reduction in pain, leading to an improvement in function. In addition, patients must possess the cognitive ability to understand the device’s purpose and how to manage the various stimulation patterns and settings available. Without these mental faculties, the therapy is destined to fail.
Cognition is affected by psychological factors, which, in and of themselves, can be predictive of successful therapy. These psychological factors can predict a poor response, even when the procedure is clinically indicated and the procedure is performed perfectly. The analysis necessary to assess psychological readiness for SCS is still highly controversial, because of considerable differences in the design of different studies and the opinions generated by various investigators [28].
This problem has been recognized for some time. One series of recommendations came from the European Federation of IASP Chapters (EFIC), which in 1998 presented a consensus document on neuromodulation treatment, that established exclusion criteria for SCS. The consensus was that major psychiatric disorders , which included active psychosis, severe depression, hypochondriasis, and somatization disorder predicted failure. This was based on earlier studies, which suggested that anxiety (either trait or state), other mood disorders, active suicidal behavior, active homicidal behavior, serious alcohol or drug addiction problems, and severe sleep disturbance should exclude patients from therapy [29]. Other studies predicted therapy failure in patients showing poor medical compliance, lack of appropriate social support, history of drug and/or alcohol abuse, and drug-seeking behavior [30]. Some contraindications can be more insidious than others and can be missed by practitioners performing psychosocial evaluations. One example includes somatoform disorders, which are characterized by the presence of physical symptoms suggesting a medical condition, but in fact are derived from a patient’s inability to accept unresolved emotional issues [28]. Another example involves personality disorders, which include borderline, avoidant, dependent, and obsessive-compulsive personality disorders . Thus, effective psychological screening should include both a personal structured psychological interview and appropriate psychometric testing to formalize a meaningful diagnosis; however, providers are still sometimes unable to predict, with certainty, which patients will do well with SCS and which will not [28].
In addition, there are physical characteristics that must be considered prior to electrode implantation. Patients should have thoracic imaging to ensure that there is adequate space to accommodate the device, without producing iatrogenic spinal cord compression. These include flexion and extension X-rays, which should assess for scoliosis, as well as severe anterior or retrolisthesis, which may obviate the need for corrective spine surgery instead of SCS. An MRI would also help to determine the presence of severe soft tissue disturbances, such as ligamentum flavum hypertrophy or severe epidural lipomatosis. Any sign of local infection near the surgical site, sepsis, coagulopathy, or condition that prevents fluoroscopic needle guidance or appropriate consent should be avoided.
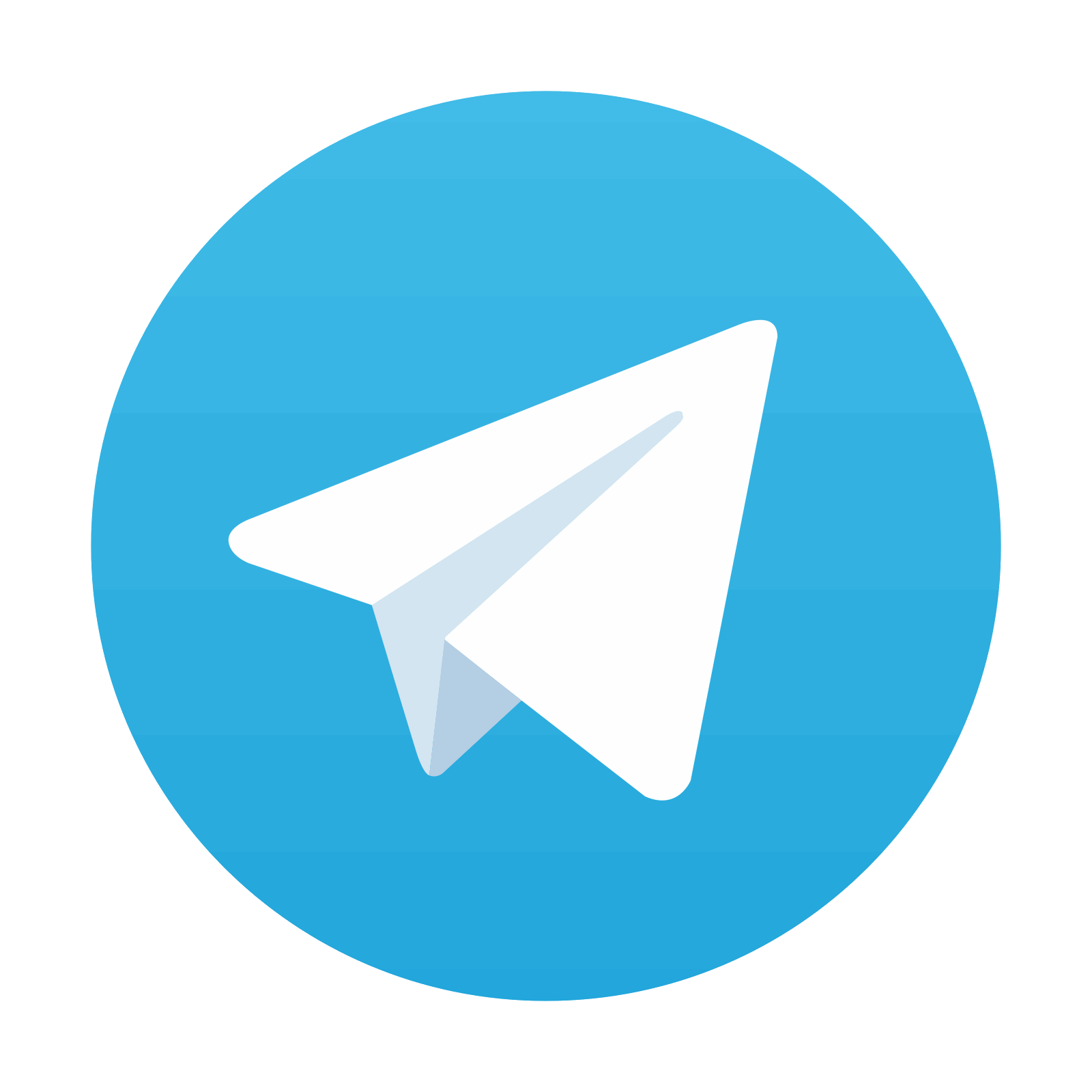
Stay updated, free articles. Join our Telegram channel

Full access? Get Clinical Tree
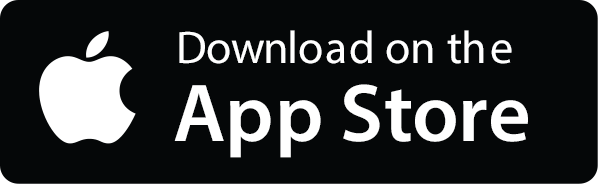
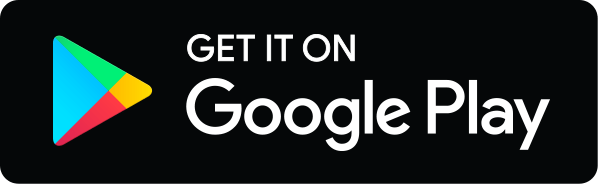