© Springer International Publishing AG 2018
Ehab Farag, Maged Argalious, John E. Tetzlaff and Deepak Sharma (eds.)Basic Sciences in Anesthesiahttps://doi.org/10.1007/978-3-319-62067-1_2727. Blood Products Transfusion
(1)
Department of General Anesthesiology, Cleveland Clinic, Cleveland, OH, USA
27.1 Introduction
27.2 Donation of Blood
27.2.1 Compatibility Testing
27.3.1 Whole Blood
27.3.2 Red Blood Cells
27.3.3 Platelets
27.3.4 Frozen Plasma
27.3.5 Cryoprecipitate
27.4 Factor Concentrates
27.4.1 Factor VIII
27.4.2 Factor IX
27.4.3 Antithrombin III Concentrate
27.4.4 Fibrinogen Concentrate
27.5 Albumin
27.5.1 Hydroxyethyl Starch
27.5.2 Dextrans
27.6.1 Immune Reactions
27.6.2 Febrile Reactions
27.6.5 Bacterial Contamination
27.6.6 Post-transfusion Purpura
27.6.7 Infections
27.6.8 Massive Transfusion
27.6.12 Immunomodulation
Keywords
TransfusionRed blood cellsFrozen plasmaPlateletsCryoprecipitateTransfusion reactionCross matchCoagulopathyMassive transfusionKey Points
- 1.
Transfusion of blood products can be lifesaving, however, it is not risk free. Therefore it is the responsibility of the physician to use appropriate triggers for blood component therapy.
- 2.
ABO and the Rh systems are the most important in the majority of blood transfusions, although human red cell membranes contain as many as 300 different antigenic determinants.
- 3.
Indication for red blood cell (RBC) transfusion is the need to increase the oxygen-carrying capacity.
- 4.
The US Food and Drug Administration (FDA) recommends avoiding HES in critically ill adult patients and septic patients requiring ICU care.
- 5.
Transfusion-related acute lung injury (TRALI) is currently the leading cause of transfusion-related death. Clinical presentation of TRALI may be indistinguishable from acute respiratory distress syndrome (ARDS) and is characterized by acute onset, bilateral pulmonary infiltrates and hypoxia without evidence of congestive heart failure (CHF).
27.1 Introduction
During the perioperative period, some patients may require transfusion of blood products. The most common cause and indication for administration of blood components is acute surgical blood loss. In such a situation, transfusion of blood products can be lifesaving, however, it is not risk free. Therefore it is the responsibility of the physician to use appropriate triggers for blood component therapy. When there is clinical evidence of a deficiency in oxygen-carrying capacity, red cell transfusion should be considered; and if clinically significant coagulopathy is present, transfusion of hemostatic blood product should be considered. There are no mandatory thresholds for the transfusion; the clinician should take into consideration the patient’s medical condition, the surgical procedure, symptoms, and the rate of blood loss when deciding if and when to start blood therapy.
Although transfusion of whole blood is still used in certain clinical circumstances, blood components are transfused more often to correct specific deficiencies. The primary advantage of component therapy is that only the needed, specific fraction of the blood is administered, allowing several patients to benefit from 1 donation and administration of unnecessary or unneeded components is avoided. In addition, separation of the whole blood into components permits each to be stored under optimal conditions to enhance and preserve efficacy. The primary disadvantage of component therapy is encountered in treating patients with massive blood loss requiring massive transfusion, since these patients would benefit from whole blood to restore oxygen carrying capacity as well as hemostatic function. Multiple components are more expensive and more difficult to transfuse in such situations as compared to whole blood, and exposure to various complications of transfusion can be increased as the number of donor exposures increases.
27.2 Donation of Blood
A blood donor may donate whole blood or donate a specific component of blood using apheresis technology. At the time of whole blood donation, blood is collected into a sterile plastic blood reservoir containing an anticoagulant and preservative. Integral tubing connected with satellite bags allows for the separation of whole blood into various components using differential speed centrifugation techniques. One whole blood unit may be separated into 1 unit of plasma, 1 unit of red cells, and 1 unit of whole blood-derived (“random donor”) platelets. Each of these components is then stored under optimal conditions. Apheresis technology is used to collect needed components (red cells, platelets, or plasma) from a donor and then return the remaining constituents to the blood donor.
In order to provide adequate safety for the donors and recipients of blood, all blood donors are screened to determine suitability for donation. This process includes donor history and a physical examination, and then if the donor meets criteria, testing of the donor blood specimen. Donor blood is routinely tested for human immunodeficiency virus (HIV) l/2, human T-cell lymphotropic virus (HTLV) I/II, hepatitis C virus (HCV), hepatitis B virus (HBV), West Nile virus, and Treponema pallidum. Since the use of the newest nucleic acid testing technology, the seroconversion window has decreased to 11 days for HIV and 8–10 days for hepatitis C. Hence donation by seronegative individuals during the period of seroconversion can pose the risk of transmitting infection.
Some patients may request “designated donors”: ABO compatible donors known to a patient and selected for donation with the stipulation that their blood be reserved for a specific patient’s use. However, the concept that “designated donors” provide safer blood than units collected from the volunteer donors is not valid. Because of the potential increased risk for alloimmunization of an Rh negative female who receives a blood transfusion from a Rh positive male sexual partner and subsequent hemolytic disease of the newborn, blood transfusions from a male donor to a female sexual partner are not recommended. Cellular blood components from blood relatives carry an increased risk of causing transfusion associated graft-versus-host disease (TA-GVHD), even in an immunocompetent recipient, and should be irradiated to prevent TA-GVHD.
Limited donor exposure transfusion is based on the assumption that decreasing the number of donor exposures will result in a concurrent decrease in transfusion-related complications. This is most often used in the pediatric and neonatal patient populations. A donor, often a parent, may donate multiple units of blood over a period of time designated for a particular patient. Transfusion services may assign a particular RBC unit to a pediatric patient and take aliquots from the RBC unit for the shelf-life of the unit.
27.2.1 Compatibility Testing
Compatibility testing is done to prevent transfusion of incompatible blood that may result in a hemolytic transfusion reaction. ABO and the Rh systems are the most important in the majority of blood transfusions, although human red cell membranes contain many more different antigenic determinants. Individuals generate antibodies to the alleles they lack within each system or generate them in response to sensitization from a previous transfusion or pregnancy.
A type and screen (T&S) consists of typing the patient’s red cells for ABO and Rh blood groups and screening the patient’s plasma or serum for the presence of unexpected non-ABO antibodies. The ABO group is determined by typing the patient’s red cells using anti-A and anti-B reagents (forward type) and by testing the patient’s serum against A and B reagent cells (reverse type). The patient’s RBCs are also tested with anti-D for the presence (Rh positive) or absence (Rh negative) of the D antigen. Also, the patient’s plasma is screened for the presence of unexpected antibodies by incubating it with 2 selected screening cells that contain all of the critical non-ABO antigens using an antihuman globulin (AHG) technique (indirect antiglobulin test or Coombs test). If an antibody is detected in the patient’s plasma, the screen is considered positive. ABO group, Rh type, and antibody detection screening take about 45 min to perform. If the antibody screen is negative and the patient has no history of detected antibodies, the patient may receive RBCs that are tested for ABO compatibility by performing an immediate spin crossmatch or an electronic/computer crossmatch. ABO- and Rh-compatible blood is selected from the inventory and issued within 5–10 min. If the antibody screen is positive, the unexpected antibody or antibodies must first be identified before antigen negative-compatible RBC units can be found and then crossmatched—all of which usually takes several hours [1].
During a crossmatch, the patient’s serum is incubated with red cells from a specific donor unit to verify in vitro compatibility. A crossmatch is performed with a short incubation time at room temperature (immediate spin) intended solely to verify ABO compatibility or as a long incubation time at 37 °C (AHG crossmatch) intended to verify compatibility for clinically significant non-ABO red cell antigens. The immediate spin crossmatch takes 5–10 min, while the AHG crossmatch takes at least 45 min. The electronic/computer crossmatch may be performed instead of an immediate spin crossmatch and uses a computer system to select an RBC unit based on a series of validated computer algorithms. Serologic testing of the donor RBC unit with patient specimen is NOT performed in this scenario. An AHG crossmatch is only required for patients with a current or past history of clinically significant unexpected antibodies in their plasma or when a patient develops unexplained, acute anemia after recent transfusion [1].
In an emergency situation when there is a need for transfusion, type-specific or type O Rh-negative red cells can be administered while awaiting a formal crossmatch to be performed. Type O Rh-positive red blood cells for males or postmenopausal females can be transfused in this setting as well. Administration of Group O uncrossmatched blood is safe as long as the patient has not been already alloimmunized to any non-ABO red cell antigens. Administration of a substantial number of Group O Rh-negative blood may potentially lead to hemolysis if multiple units of Group O whole blood (containing anti-A and anti-B antibodies) have been transfused to patients with Group A or B blood. The patient can be switched back to native type-specific blood after subsequent compatibility testing.
27.3 Whole Blood and Blood Components
27.3.1 Whole Blood
Indications
restoration of oxygen carrying capacity and restoration of hemostatic function in setting of massive blood loss.
Whole blood units contain approximately 450–500 mL donated blood plus approximately 70 mL of a citrate-based anticoagulant-preservative solution, which helps to maintain the viability of red blood cells. The whole blood collected and stored with citrate-phosphate-dextrose-adenine (CPDA-1) solution has a 35-day shelf life and a hematocrit of approximately 35%. Whole blood is rarely transfused in a current clinical practice but it may be indicated for acute, massive blood loss. In cardiac surgery, transfusion of fresh whole blood was tested as an alternative to using red blood cells, platelets, and fresh frozen plasma (FFP) and decreased need for component transfusion. Although 1 study showed less postoperative blood loss in infants, the majority of the studies concluded that the logistic problems of obtaining fresh blood from a prescreened donor (to be transfused within 12 h of collection) outweighed any advantages. Nonetheless, its indication for massive transfusion—particularly in combat and in disasters—is still under investigation. Because of the logistic, very few blood centers or hospitals maintain an inventory of CPDA-1 whole blood.
27.3.2 Red Blood Cells
Indications
increasing the oxygen-carrying capacity.
One unit of whole blood is separated into red blood cells and platelet-rich plasma by centrifugation and collected in CPDA-1 anticoagulant-preservative solution with a hematocrit of approximately 70% and a shelf life of 35 days. If 100 mL of Adsol® (AS-1), Nutricel® (AS-3), or Optisol® (AS-5) is added to red blood cells with CP2D or CPDA-1, shelf life is prolonged to 42 days reducing the hematocrit to approximately 60%. Red blood cells are only indicated for raising the oxygen-carrying capacity, although they also provide volume when given to patients who are acutely hemorrhaging.
Glycerolized red blood cells are stored frozen at temperatures of −65 °C or lower for up to 10 years. Glycerol is used to protect the red cells during freezing and thawing and is removed by washing before transfusion. An automated closed cell processing systems in conjunction with using nutrient-additive solutions, such as AS-3, have extended the post-wash storability of the blood to 14 days following thawing. This approach is indicated for prolonged storage of rare red cells for patients with antibodies to red cells with rare red cell antigen phenotypes, storage of Group O red blood cells to treat patients during times of shortage.
Leukocyte-reduced red blood cells were primarily indicated in the past for patients with a history of multiple febrile nonhemolytic transfusion reactions, for select patients who were frequent transfusion candidates and thus at risk for alloimmunization to leukocyte antigens, and for prevention of cytomegalovirus (CMV) infection in high-risk patients who were immunocompromised. For the same reasons, leukocyte-reduced red blood cells are increasingly being used in the USA general population of transfused patients, and universal leukocyte reduction is now mandated in Canada and many European countries as well. Certain patient populations at high risk (eg, trauma, cardiac surgery), with systemic endothelial activation/dysfunction related to the systemic inflammatory response, may benefit from leukoreduced units to attenuate target organ injury. This is supported by randomized, controlled trials (RCTs) involving nearly 2500 patients, revealing a 50–70% reduction in mortality in patients who were randomly assigned to receive leukoreduced packed red blood cells (PRBC) units [2]. Third-generation adsorption filters enable the removal of 99.9% of donor leukocytes and are more effective than the cell washing and centrifugation techniques used previously.
Washed red blood cells are prepared by centrifugation with saline to remove almost all plasma. They are indicated only for patients who have had severe allergic reactions associated with transfusion or immunoglobulin A (IgA) deficiency. Washed red blood cells must be given through a standard blood filter, and can be stored no longer than 24 h because of the risk of bacterial contamination following washing in an open system. Washing of red cells may be used to remove excess potassium from older units as well.
Irradiated whole blood or red blood cells are blood components that have been exposed to a standard dose of ionizing (gamma) radiation to render viable lymphocytes incapable of engraftment in premature newborns, highly immunocompromised patients (e.g., bone marrow or solid organ transplant), and blood relatives of directed donations to reduce the possibility of transfusion-related graft-versus-host disease. Increased membrane permeability has been noted after irradiation, with viability of the irradiated red cells leaking potassium at an accelerated rate. Mild functional impairment manifested by significant leakage of potassium and accumulation of plasma hemoglobin has been demonstrated subsequent to gamma irradiation. This issue can become especially problematic if blood is stored for extended periods of time following irradiation, resulting in rare but serious incidences of hyperkalemic cardiac arrhythmia or serious conduction abnormality. Washing the red cell component can be used to remove excess potassium. Irradiated red blood cells have a reduced storage period (not to exceed 28 days after irradiation) in order to limit the deleterious effects this treatment can have [3].
In general, red blood cells are indicated in symptomatic, anemic patients to restore oxygen-carrying capacity. Red cells may be used in the setting of severe bleeding (e.g., >1–2 liters/hour) in an attempt to manage ongoing hypovolemia/anemia. Transfusion volume required for individual patients can be estimated using the patient’s hematocrit, blood volume, and state of hydration. In many cases, transfusion rates and/or amounts can be effectively reduced by employing blood conservation techniques. One unit of red blood cells will increase the hematocrit by approximately 3% and the hemoglobin by about 1 g/dL in the average adult. Ten mL/kg of red blood cells will raise hematocrit by 10%. The increase in the recipient’s hematocrit will vary depending upon many factors, which include the donor’s hematocrit, the recipient’s fluid status and size, the anticoagulant-preservative solution utilized, the rate of active bleeding, and the duration of storage of the unit transfused.
Calcium-containing solutions must not be added to blood, particularly at slow infusion rates, because small clots may form due to the presence of calcium in excess of the chelating ability of the citrate anticoagulant. Hypotonic solutions such as 5% dextrose in water should not be used to dilute red cells since clumping of the cells or hemolysis may occur.
Even though an anticoagulant is added to blood at the time of the collection from the donor, small clots are occasionally present, which requires filtration at the time of infusion. Standard blood administration sets usually have a clot-screening filter with a pore size of 170–200 μ(mu)m. These filters permit rapid transfusion and should be used for administration of red cell components, platelet and granulocyte concentrates, FFP, and cryoprecipitate.
During storage of red cells, microaggregates consisting of platelets, leukocytes, and fibrin form. These microaggregates are able to pass through 170 μ(mu)m filters and lodge in the pulmonary circulation. For that reason use of microaggregate (20–40 μ[mu]m) filters has become increasingly popular, although it has not been proven to reduce the incidence of respiratory distress syndrome in patients receiving multiple transfusions. The usefulness of microaggregate filters is still debated and there is no firm indication for their use during routine transfusions, even when large volumes of blood are administered (massive transfusion).
27.3.3 Platelets
Indications
correction of a deficiency in either platelet number or function in clinical situations of ongoing or anticipated bleeding.
Random-donor platelets can be prepared from whole blood stored at 22 °C within 8 h of collection. After the collection of approximately 500 mL of whole blood into citrate-based anticoagulant-preservative containing collection bags, the blood is centrifuged; the platelet-rich plasma (PRP) is separated into an attached empty satellite bag. This PRP is centrifuged again and separated into 1 unit of platelet concentrate and 1 unit of plasma. Each unit of platelets contains approximately 5 × 1010 platelets in 50–70 mL of plasma [4].
Platelets can be also isolated from the buffy coat layer, following centrifugation of whole blood in specific bags that remove RBC and plasma through tubing in the bottom and top of the bags. The platelet-enriched buffy coat is further processed (through centrifugation and/or leukoreduction filters) to eliminate white blood cells (WBCs) and remaining RBCs. This method is currently employed mostly in Europe and Canada and it permits storage of whole blood at 22 °C for up to 24 h prior to platelet removal [4].
Another method to obtain platelet concentrate is apheresis. Platelets (single-donor) are obtained by performing apheresis on volunteer donors. During this procedure, large volumes of donor blood are processed into an extracorporeal circuit and centrifuged to separate the components. The red blood cells and a certain percentage of the plasma are returned to the donor. A single donor donates the equivalent of 3–5 × 1011, or 4 to 6 units, of platelets suspended in a volume of 200–400 mL of plasma. Pheresis-derived platelets minimize the number of donor exposures and increases the amount of collected platelets and it has become the primary source of platelets in the US [4].
Platelets should be stored at room temperature (20–24 °C) for up to 5 days with continuous gentle agitation to prevent platelet aggregation. All platelet products should be tested for bacterial contamination prior to transfusion. The administration of ABO-specific platelets is not strictly (ie, usually limited to 300–500 mL of out-of-group plasma) required because platelet concentrates contain few red blood cells. However, administration of non-ABO specific platelets may be of concern with transfusion of pediatric patients with a small blood volume because of anti-A and/or anti-B in the plasma. The administration of out-of-group pooled platelet components leads to transfusion of plasma containing anti-A and/or anti-B, resulting in passive alloimmunization and may cause a weakly positive direct antiglobulin test due to anti-A and or anti-B from the plasma.
Platelets transfusion is indicated to correct a deficiency in either platelet number (thrombocytopenia) or platelet function (thrombocytopathy or qualitative platelet disorders). One unit of apheresis platelets or a pool of 4–6 whole blood-platelets (derived from 4–6 donors) increases the platelet count by approximately 3–5 × 1010/L in the average adult. For pediatric patients, a dose of 10 ml/kg or 1 unit of platelets/10 kg will generally increase the platelet count to adequate levels. Factors to consider for the transfusion of platelets for counts between 5 and 10 × 1010/L are the type of surgery, extent of actual blood loss or microvascular bleeding, presence of potent antiplatelet medications (e.g., clopidogrel, IIb/IIIa antagonists, etc.) and disorders, such as uremia, known to affect platelet function and coagulation. Operations at closed sites (e.g., neuro or ophthalmic surgery) usually require increasing the platelet count 100 × 109/L in order to ensure adequate hemostasis. Surgical procedures associated with insignificant blood loss may be undertaken in patients with platelet counts less than 50 × 109/L. The platelet count alone does not guarantee adequate platelet function and platelet transfusion may be indicated, even above 100 × 109/L count, if platelet dysfunction is suspected and/or there is a recent history of taking aspirin or other more potent or longer half-life platelet-inhibiting drugs (e.g., clopidogrel). Potent agents such as glycoprotein IIb/IIIb antagonists may require 2 or more apheresis platelet units to achieve normal hemostasis while effects of some other agents (e.g., clopidogrel) have not consistently been shown to be reversed with platelets. Whole blood coagulation tests such as thromboelastography or thromboelastometry testing can identify platelet dysfunction more precisely. The prophylactic administration of platelets is not recommended in patients with chronic thrombocytopenia caused by increased platelet destruction (e.g., idiopathic thrombocytopenic purpura) and, in fact, may be ineffective in a substantial percentage of these patients [3].
Platelets can be infused through a platelet or standard component administration set with a 170-micron filter; platelets should not be transfused through fluid warmers or rapid infusion systems. Microaggregate filters (20-micron to 40-micron) should not be used because they will remove most of the platelets.
27.3.4 Frozen Plasma
Indications
correction of coagulopathy related to the deficiency of clotting factors.
After removal of red blood cells from the whole blood, the remaining platelet-rich plasma is further centrifuged to separate the platelets from the plasma. Separated plasma contains all the blood coagulation factors, fibrinogen, and other plasma proteins in a volume of 170–250 mL. The plasma is then frozen within 8 h of phlebotomy to prevent complete inactivation of temperature-sensitive (“labile”) coagulation factors V and VIII and stored in temperatures colder than −18 °C for up to 1 year with minimal loss of coagulant activity. Prior to the administration of FFP, the plasma must be thawed in a waterbath at 37 °C on, which takes approximately 30 min. After thawing, the units of FFP are stored at 1–6 °C and are generally transfused within 24 h. FFP that has been thawed but not used within 24 h can be relabeled as “thawed plasma” (TP) and stored at 1–6 °C for an additional 4 days. Thawed plasma maintains normal levels of all factors except factor V, which falls to 80% of normal, and factor VIII, which falls to 60% of normal. TP can be used as a substitute for FFP [5].
FFP is used for the treatment of microvascular bleeding due to congenital and acquired coagulopathies resulting in a prolongation of either the activated partial thromboplastin time (aPTT) or prothrombin time (PT) greater than 1.5 times normal, or a coagulation factor assay of less than 25%. Evidence-based data supporting administration of FFP in patients with international normalized ratio (INR) values <2.0 are lacking. In emergent situations, FFP may be used to reverse the effect of warfarin prior to surgery or during active bleeding episodes. However, if time permits, oral or parenteral vitamin K will produce the same effect in 6–12 h without exposing patients to the risks associated with allogeneic blood components. In the patient who has been transfused with more than 1–2 blood volumes and PT and PTT cannot be obtained in a timely fashion, FFP may be administered after administration of platelets to correct microvascular bleeding believed to be due to coagulation deficiency. When FFP is indicated, it should be administered in a dose calculated to achieve a minimum of 30% of plasma factor concentration. Ten to 15 mL/kg of FFP will usually result in an increase of most coagulation factors by 25–30%. FFP should be administered through a blood administration set with a 170-micron filter [3].
27.3.5 Cryoprecipitate
Indications
low fibrinogen levels or von Willebrand’s disease (deficient or abnormal von Willebrand molecule).
Cryoprecipitate is prepared from a unit of FFP; it is the cold-insoluble white precipitate that forms when a bag of FFP is thawed at 1–6 °C. This cold-insoluble material is removed following centrifugation and immediately refrozen at −18 °C to be stored at this temperature for up to 1 year. Each unit of cryoprecipitate contains 80–150 units of factor VIII, 150–250 mg of fibrinogen, von Willebrand factor, factor XIII, and fibronectin in a volume of 5–15 mL. Cryoprecipitate must be transfused within 4–6 h of thawing if given to increase factor VIII levels.
Cryoprecipitate is used primarily to augment fibrinogen levels depleted because of massive hemorrhage or disseminated intravascular coagulation (DIC). Rarely, it is used for the treatment of congenital or acquired factor XIII deficiency. For fibrinogen replacement therapy, 1 unit of cryoprecipitate per 10 kg body weight increases plasma fibrinogen by approximately 50–70 mg/dL in the absence of continued consumption or massive bleeding. The minimum hemostatic level of fibrinogen is less than or equal to 80–100 mg/dL, but many experts regard that minimal level as too low. The national guidelines in Germany and Austria recommend higher levels of 150–200 mg/dL [7]. Because cryoprecipitate does not contain factor V, it should not be the sole replacement therapy for DIC, which is almost always associated with a variety of factor deficiencies and thrombocytopenia. Hence, fresh frozen plasma also needs to be administered along with platelet concentrates in those settings where a coagulopathy secondary to DIC is likely occurring.
Cryoprecipitate should be infused through a 170- to 260-micron component filter.
27.4 Factor Concentrates
A number of plasma derivatives are available to treat coagulation deficiencies. The main advantage of their use is administration of specific deficient factor(s) and avoidance of transfusion of unnecessary blood components.
27.4.1 Factor VIII
Factor VIII concentrates are indicated to correct factor VIII deficiency (hemophilia A). Historically administration of human concentrated factor VIII was associated with a relatively high incidence of infectious disease transmission; however, advances in purification techniques and screening tests have dramatically reduced that risk. Also the development of recombinant factor VIIIC in many instances has replaced human-based blood derivatives for the treatment of factor VIII deficiency. Recombinant factor VIIIC has the major advantage of not carrying the risk of transmitting viral diseases. Mild factor VIII deficiency and Type 1 (80% of von Willebrand’s disease) may be partially corrected with desmopressin (DDAVP). Administration of DDAVP is typically associated with a significant increase in both circulating factor VIII and von Willebrand’s factor (vWf).
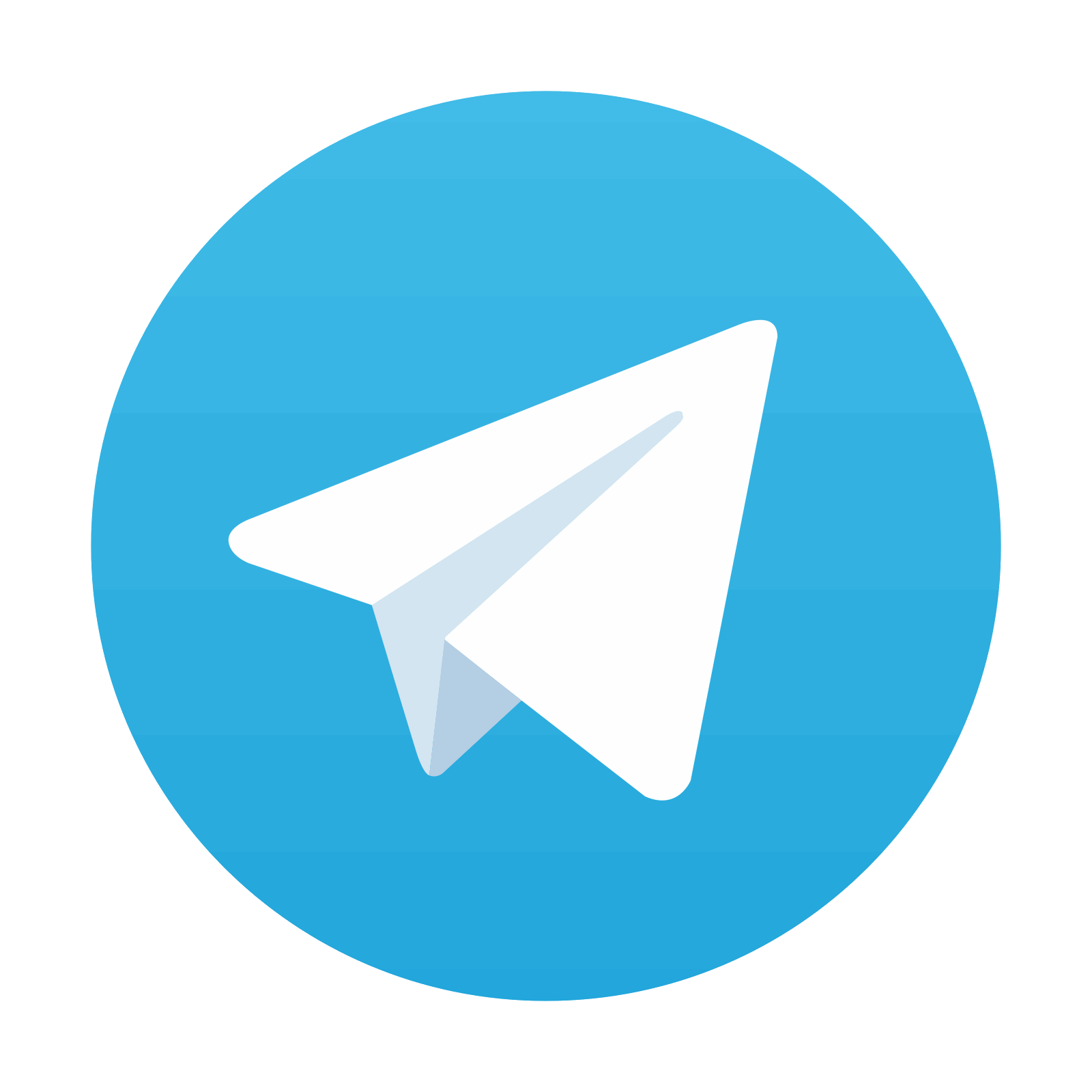
Stay updated, free articles. Join our Telegram channel

Full access? Get Clinical Tree
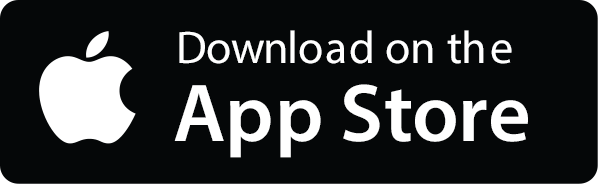
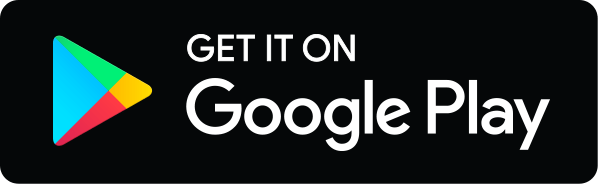