Chapter Outline
What Is an Anesthesia Machine? 23
Fresh Gas Flow 24
Fresh Gas Flow and the Rate Of Change of Gas and Vapor Concentrations 24
Total Fresh Gas Flow and the Conservation (Waste) of Anesthetic Gases 26
Using the Circle System as an “Open Circuit” 26
Using the Circle System as a “Closed Circuit” 27
Using “Minimal or Low Fresh Gas Flow” with a Circle System 28
Is It Possible to Deliver Too Little Oxygen? 29
Vaporizers 29
Scavenging Systems 30
The Anesthesia Ventilator 30
Basic Anesthesia Ventilator Design 31
Selecting the Ventilation Mode: Volume Control, Pressure Control, and Pressure Support Ventilation 32
Avoiding and Troubleshooting Anesthesia Machine Problems 36
Specific Hazards Related to the Anesthesia Machine 38
Future Possibilities 39
I recall the first time I administered anesthesia. The attending anesthesiologist injected the thiopental and muscle relaxant and the patient peacefully closed their eyes and stopped breathing. He then turned to me and said: “What are you going to do now?”
Of course, I was not completely unprepared to deliver this first anesthetic. The preoperative evaluation process was just a modified version of taking a history and performing a physical exam. I set up the room according to a straightforward protocol given to me by a more experienced person. I had even already developed some endotracheal intubation skills. In contrast, the anesthesia machine beside me was completely new. Sure, it had some resemblance to the ventilators I had seen used in the intensive care unit (ICU), but it was obviously different. Fortunately, a more experienced person guided my hand as I adjusted flows, administered potent anesthetic vapors, and adjusted the ventilator. As I reflect on the experience, I now understand how little I knew about the anesthesia machine that I relied upon to keep the patient safe and comfortable.
The anesthesia machine is essential to the practice of anesthesiology. We use it in some fashion for almost every patient we anesthetize. At the same time, the manner in which the machine interacts with the patient is complex. This chapter is intended to help the reader to develop an intuition for how the anesthesia machine can be used most effectively to anesthetize patients. Although specific machine designs will be used to illustrate the concepts, the intention is not to focus on a particular machine design. Virtually all modern anesthesia machines can be used safely and effectively if the anesthesia provider understands the capabilities and limitations of the machine. The most commonly used design of a machine with a circle system and anesthetic vaporizer will be the focus of this chapter ( Figure 3–1 ).

The ultimate goal of this chapter is to help the novice to use the anesthesia machine safely and effectively. By the end of the chapter, the reader will understand how to set the fresh gas flow, deliver anesthetic vapor effectively, and use the ventilator. Machine checkout and common causes of patient injury and machine failure are also reviewed. The engineering of the machine will not be discussed because that information is readily available in other sources. Hopefully, the information in this chapter will stimulate the curiosity to explore some of the suggested references at the end of the chapter, which will lead to mastery of the anesthesia machine.
What Is an Anesthesia Machine?
Simply put, an anesthesia machine is designed to deliver fresh gas (typically oxygen and air or nitrous oxide) and anesthetic vapor to a patient through a breathing circuit and allow for spontaneous, manual, or mechanical ventilation. Much of the design is focused on minimizing the waste of anesthetic vapors and preventing contamination of the operating room (OR) environment with anesthetic gases. There are typically monitoring functions built into the machine that verify proper machine function and help to detect potentially unsafe conditions. Various anesthesia machine designs are available, all of which have differing capabilities, but the basic function of the machine is universal.
The ideal anesthesia machine design would provide instantaneous control of concentrations of oxygen and anesthetic vapor delivered to the patient, and have the capabilities of an intensive care ventilator. Further, the machine would be easy to use and never break down or malfunction. It would be entirely safe without any potential to injure a patient. Unfortunately, the ideal anesthesia machine does not exist, so the informed clinician must understand the capabilities and limitations of the machine(s) they use for patient care.
Fresh Gas Flow
All anesthesia machines provide controls to select and adjust the fresh gas that is introduced into the anesthesia circuit. Typically, these controls are mechanical flowmeters with valves that can be opened to precisely control the flow of pressurized gases into the breathing circuit. Newer machines may have electronic flowmeters but the basic function is the same. There is always an oxygen flowmeter and usually additional flowmeters for nitrous oxide and air. Specialty anesthesia machines can also have flowmeters for helium and carbon dioxide but the use of these gases is beyond the scope of this chapter.
So how should fresh gas flow be adjusted? The operator can make choices about the types of gases to deliver and the flow rate of each gas to be delivered.
Which Types of Gases Should Be Mixed in the Fresh Gas Flow?
The oxygen flow setting is probably the most used control on the anesthesia machine. The novice may decide to only administer oxygen to a patient and feel comfortable that the patient will be as safe as possible. Selecting the anesthetic gases to be delivered should, however, be carefully considered ( Table 3–1 ).
Gas | Advantages | Disadvantages |
---|---|---|
O 2 |
|
|
Nitrous Oxide |
|
|
Air |
|
|
If oxygen alone is delivered, the patient will receive 100% oxygen in the inspired gas. Although oxygen is generally considered beneficial, there are disadvantages to delivering only oxygen to the patient. Oxygen toxicity is classically described as a disadvantage to delivering high oxygen concentrations, especially in neonates who are at risk for retinal disease in the presence of high oxygen concentration. It is arguable whether or not exposure to high oxygen concentrations for the relatively brief periods during anesthesia leads to complications of oxygen toxicity. Nevertheless, it is accepted practice to reduce the oxygen concentration especially for neonates.
There are disadvantages to delivering 100% oxygen that are more commonly applicable to the anesthetized patient. It is well documented that 100% oxygen will lead to absorption atelectasis—a common cause of lung dysfunction during and after anesthesia. Pulse oximetry is typically used to assess oxygenation during anesthesia, but only estimates oxyhemoglobin saturation. In the presence of an enriched oxygen concentration, the oxyhemoglobin saturation may be 100% despite the presence of significant ventilation perfusion mismatch. Using an increased oxygen concentration will potentially hide an oxygenation problem that could otherwise be identified by the pulse oximeter as a reduced oxyhemoglobin saturation. Oxygen also supports combustion and it must be avoided in high concentrations when there is a risk of fire (e.g., airway surgery) or by open delivery (e.g., nasal cannula) during head and neck surgery.
There are situations when the patient’s medical condition dictates the need for inspired gas consisting of 100% oxygen. Most commonly, 100% oxygen is used during induction of, and emergence from, general anesthetic to reduce the risk for significant hypoxemia if there are problems maintaining an adequate airway. Patients with critical lung disease due to ARDS or an acute lung injury often require 100% oxygen to maintain an acceptable oxyhemoglobin saturation. Lung isolation procedures where only one lung is ventilated can facilitate surgery, but are associated with pulmonary shunt and ventilation perfusion mismatch often requiring 100% oxygen. There is some debate in the surgical literature about the potential for increased oxygen concentrations to reduce the risk of postoperative wound infection, but the evidence is not sufficiently conclusive to guide anesthetic practice.
If gas exchange in the lungs is normal, oxyhemoglobin should be 100% saturated with oxygen when only 21% oxygen is present in the inspired gas. Given the disadvantages of using 100% oxygen and the fact that it is not required to maintain adequate oxygen delivery, oxygen is typically mixed with another gas by the anesthesia provider.
Oxygen is mixed with nitrous oxide when a reduced inspired oxygen concentration is acceptable and the anesthetic properties of nitrous oxide are desired. Nitrous oxide is a relatively weak anesthetic agent and will increase the effective delivered MAC, but is most useful at concentrations of at least 50% and ideally 70%. When nitrous oxide is used, the ratio of nitrous oxide to oxygen flow should provide concentrations of N 2 O and O 2 of 50% each or even 70%:30%, respectively. These ratios can be achieved with a variety of total flow settings and the rationale for selecting a particular magnitude of flow will be discussed below. Nitrous oxide can be especially useful during inhalation inductions with a potent anesthetic, such as sevoflurane, where the so-called second gas effect enhances the uptake of the potent anesthetic vapor. Nitrous oxide is often avoided for different reasons. It is not desirable when there are confined air spaces in the body where expansion of the air space in the presence of N 2 O is not desired. Examples include bowel surgery especially for intestinal obstruction or ear, eye, or intracranial surgery. There is arguable evidence that N 2 O increases the incidence of postoperative nausea and vomiting which can be the reason for avoiding N 2 O. Like oxygen, N 2 O also supports combustion and it should be avoided if there is a risk of fire.
Air is the most common gas delivered with oxygen when N 2 O is not administered. Air does not have any anesthetic properties but is an ideal balance gas for reducing the inspired oxygen concentration. Air contains 21% oxygen and can be used as the only fresh gas supplied to the patient assuming that ventilation is adequate and there is no lung pathology impairing gas exchange. Due to the oxygen already present in air, not much additional oxygen flow is needed to significantly enrich the inspired oxygen concentration ( Table 3–2 ).This is an important consideration when it is important to minimize the inspired oxygen concentration such as when there is potential for an OR fire. Some anesthesia machines are only equipped with oxygen and nitrous oxide flowmeters. This configuration is a significant limitation and leaves the anesthesia provider with only the ability to deliver 100% oxygen or oxygen and nitrous oxide. When the risk of fire is a concern, this type of anesthesia machine should not be used.
Air Flow Setting | O 2 Flow Setting | Resulting Inspired O 2 Concentration |
---|---|---|
1 | 0.5 | 47% |
1 | 1 | 60.5% |
2 | 0.5 | 37% |
2 | 1 | 67.6% |
5 | 0.5 | 28% |
It is useful to have an intuition for which gases to select to achieve a desired inspired oxygen concentration. Fortunately, inspired oxygen concentration monitors are standard on most anesthesia machines and considered a standard of care in much of the world. The inspired oxygen monitor should be used, especially by the novice, to confirm that the desired oxygen concentration has been achieved or to guide adjusting the gas flows accordingly.
How Much Flow Should Be Set for Each Gas?
In the previous section, the considerations for selecting the types of gases in the fresh gas were discussed. The combination of flows of each of the gases set to be delivered will determine the concentrations of each of the gases that result. Many combinations of flows can be used that will result in the same concentrations of gases in the circuit. For example, it would seem that 50% O 2 and 50% N 2 O could be achieved by using equal flows of each gas at any setting, the only difference being the magnitude of the total flow. However, as we will see, as the total flow is lowered, the actual concentrations that result will be influenced by the patient’s oxygen consumption. There are other implications that should be considered when selecting the actual gas flow setting. The total flow set to be delivered is dictated in part by the desired concentrations of the individual gases, but also by 1) the desired rate of change of gas and anesthetic vapor concentration and 2) the desire to minimize the waste of anesthetic vapor and gases by rebreathing exhaled gases.
Fresh Gas Flow and the Rate of Change of Gas and Vapor Concentrations
In our standard anesthesia machine, the gases delivered by the flowmeters pass through the anesthetic vaporizer and enter the anesthetic circuit as a mixture of vapors and gases. That mixture then combines with the existing gases in the breathing circuit to determine the combination of gases delivered to the patient. It is often desirable to change the concentrations of gases and vapor in the circuit rapidly. The most notable examples are during induction and emergence when the inspired anesthetic vapor concentration must be increased and decreased respectively. Although the actual factors that determine the rate at which the inspired concentration delivered to the patient changes are complex, the total fresh gas flow and the volume of the breathing circuit are major factors.
The rate of change of gas concentrations in the anesthetic circuit can be estimated by dividing the total volume of the circuit by the total fresh gas flow. The resulting number is the time constant, or the time it takes for the concentration of gases in the system to reach 63% of the final concentration. For example, consider the case where the total volume of the anesthetic system is 5 liters. If the total fresh gas flow is 5 liters per minute, the time constant is one minute, and it will take four time constants (4 minutes) for the concentrations in the system to reach 98% of the concentrations in the fresh gas. Similarly, if the fresh gas flow is 1 liter per minute, the time constant will be 5 minutes and it will take 20 minutes to reach 98% of the concentrations in the fresh gas. This estimation process is useful to gain an intuition for the impact of the total fresh gas flow and the volume of the circuit on the rate of change of concentration. It is not entirely accurate since the actual time depends upon a complex relationship of the geometry of the anesthetic system, the volume delivered to the patient with each breath and the ventilator settings. A complete explanation of these relationships is beyond the scope of this chapter. Suffice it to say that the higher the fresh gas flow, the more rapidly the concentrations of gases in the circuit will change and based upon the time constant estimation, relatively high fresh gas flows are needed to effect a rapid change in concentrations ( Figure 3–2 ).

Total Fresh Gas Flow and the Conservation (Waste) of Anesthetic Gases
Before the introduction of the circle anesthesia circuit, anesthetic vapors were delivered in an open circuit configuration. Patients inhaled the anesthetic gases and exhaled gases never returned to the patient. This design was inherently wasteful as any anesthetic vapors that were exhaled were basically lost, primarily as contaminants, into the OR environment. In an effort to reduce the amount of waste, the circle circuit was designed to allow for exhaled gases to return to the patient. The total amount of fresh gas flow determines how much of the exhaled gases return to the patient. This important fact will be used to explain the concepts of an Open Circuit, Closed Circuit, and Minimal (Low) Fresh Gas Flow approach to fresh gas delivery ( Table 3–3 ).
Functional Circuit Configuration | O 2 Only Flow (L/min) | O 2 /Nitrous Oxide Flow (L/min) | O 2 /Air Flow (L/min) | Comments |
---|---|---|---|---|
Open Circuit | 7 | 2/5 | 1/6 | Assumes minute ventilation of 7 L/min or less. No requirement for increased O 2 concentration. |
Closed Circuit | 0.4 | — | — | Only O 2 is given to match O 2 consumption (assumed 400 mL/min) once concentrations of other gases and vapors are achieved. |
Minimal Flow | ||||
Induction | 7 | 2/5 | 1/6 | Higher flows reflect need to change concentration in the circuit rapidly and make up for rapid uptake of anesthetic from the lungs. |
Maintenance | 0.4 | 0.4/1.0 | 0.2/1.0 | Based on estimated O 2 consumption of 400 mL/min Air adds to available O 2 . Use inspired O 2 monitor to ensure that inspired O 2 concentration is not decreasing. |
Using the Circle System as an “Open Circuit”
The concept of the open circuit is fairly intuitive. In essence, if the total fresh gas flow is so great that it supplies all of the inspired gas to the patient, then none of the exhaled gas returns to the patient, i.e., all of the exhaled gas leaves the circuit as waste. The open circuit is desirable when a rapid change in inspired gas concentration is desired since the inspired gas concentrations will equal those in the fresh gas flow, and any changes in gas or vapor concentrations will be reflected in the inspired gases rapidly ( Table 3–3 ).
So how much fresh gas is needed to achieve an open circuit? A general rule of thumb is that total fresh gas flow in excess of minute ventilation will result in an open circuit, i.e., no rebreathing of exhaled gases. The exact amount of fresh gas needed to achieve an open circuit will vary between anesthesia machine designs. Breath to breath monitoring of fresh gas and vapor concentrations is common in modern anesthesia practice. Inspired gas concentration monitoring

It is interesting to note that the open circuit can be useful in the event of a failure of the carbon dioxide absorbent material. Since no exhaled gases return to the patient when fresh gas flow is sufficient to create an open circuit, CO 2 absorption is not required. When using a properly functioning circle system, the inspired carbon dioxide should be zero. If the CO 2 absorbent becomes saturated and can no longer absorb carbon dioxide, inspired CO 2 will increase and the capnogram will indicate inspired carbon dioxide throughout inspiration. The definitive solution is to replace the absorbent material, but increasing the fresh gas flow to exceed minute ventilation will “open the circuit “ and eliminate the inspired CO2 until the absorbent can be replaced.
Using the Circle System as a “Closed Circuit”
Delivering anesthesia via a closed circuit is an interesting and challenging activity. The basic concept is to deliver only enough fresh gas and anesthetic vapor to replace what is used by the patient. In essence, this means setting the oxygen flowmeter to deliver only enough oxygen to meet the metabolic demands or oxygen consumption, and only enough anesthetic vapor to replace the amount of vapor taken up by the body. Delivering anesthesia by closed circuit is not particularly convenient. A leak proof anesthesia circuit is required and a means to add anesthetic vapor rapidly to the inspired gas despite a low total fresh gas flow intended to only match oxygen consumption. Due to the practical constraints, the details of performing closed circuit anesthesia are primarily of historical interest although the science and methods are well described. Anyone interested in developing a thorough understanding of the circle system will benefit from learning about closed circuit anesthesia ( Figure 3–4 ; see also Chapter 4 ).

Using “Minimal or Low Fresh Gas Flow” with a Circle System
Determining the total magnitude of fresh gas flow to provide at any given time is a balance between providing enough fresh gas flow to effect a change in inspired gas or vapor concentrations at the desired rate, and conserving gases and vapors by returning as much of the exhaled gas to the patient as possible. The open circuit and closed circuit conditions exemplify the opposite extremes of these two goals. Low flow anesthesia is a term often used to describe the use of reduced fresh gas flows to conserve gas without the challenges of a true closed circuit anesthetic. “Minimal flow anesthesia” may be more descriptive of the goals since it indicates the lowest flow needed to achieve the desired balance between rate of change of concentrations and conservation.
Initial delivery of anesthetic vapor to a patient requires a fairly high total gas flow if a rapid induction is desired. A minimal flow anesthetic would require much higher fresh gas flows in this phase of the anesthetic to achieve the desired goal of a rapid induction than during the maintenance phase of anesthesia. These relationships can be observed during induction by comparing the vaporizer setting with the measured concentrations of inspired and expired anesthetic vapors. If the fresh gas flow is high enough to approach an open circuit, the inspired concentration of vapor should approximate the vaporizer setting. The expired vapor concentration will initially be much lower than the inspired concentration but as induction proceeds, the difference between inspired and expired concentrations will diminish to reflect a reduced amount of uptake of anesthetic vapor. As the anesthetic vapor delivery continues, the rate of uptake of anesthetic vapor by the body will diminish, and the difference between inspired and expired anesthetic concentrations will become small. The total fresh gas flow can then be reduced to minimize waste by returning exhaled gas to the patient.
It is possible to approach a closed circuit configuration during maintenance of anesthesia by reducing the total amount of oxygen to approximate oxygen consumption. It is important to ensure that total oxygen flow equals the sum of oxygen consumption and any leaks from the circuit. If an aspirating (sidestream) gas analyzer is being used to sample gas from the breathing circuit, the volume removed must be replaced by the fresh gas flow unless it is returned to the breathing circuit via a return circuit. The volume of oxygen provided is the total flow from the oxygen flowmeter and 21% of the flow from the air flowmeter if it is being used. If air or nitrous oxide are being delivered, it is difficult to approximate a true closed circuit and some exhaled gas will be wasted.
Special Case: Sevoflurance and Minimal Flows
The total fresh gas flow required when administering sevoflurance has been somewhat controversial. Sevoflurance is known to interact with carbon dioxide absorbents, primarily those containing sodium, barium, or potassium hydroxide, to produce Compound A ∗
∗ Compound A is short for fluoromethyl-2-2-difluoro-1-(trifluoromethyl) vinyl ether
, which has been shown to be nephrotoxic to animals. The degree of nephrotoxicity is directly related to the total concentration of Compound A and duration of exposure. Although the nephrotoxic potential in humans does not seem to be clinically important, there are recommendations to limit the exposure to Compound A. Specifically, the labeling for sevoflurance approved by the Food and Drug Administration indicates that fresh gas flow should never be less than 1 L/min and not less than 2 L/min for more than 2 MAC-hours. Fresh gas flow is used in this case to limit the exposure to Compound A by ensuring that a certain amount of fresh gas continuously enters the circuit, washing out exhaled gas and any products of chemical decomposition within the circuit.Is It Possible to Deliver Too Little Oxygen?
An adequate supply of oxygen is essential to safe anesthetic care. Unfortunately, patients have died or been permanently injured as a result of hypoxia during anesthesia. For that reason, modern anesthesia machines are designed with a variety of safeguards to ensure the availability of oxygen. Even with all of these safeguards, it is still possible to deliver too little oxygen when using a minimal or low flow anesthetic technique.
The first safeguard monitors oxygen supply pressure to the anesthesia machine and sounds an alarm if oxygen pressure falls below a preset minimum. This alarm will sound regardless of whether the machine is supplied from a piped or a cylinder source and is a safety feature required by international standards. Some oxygen flowmeters are designed to provide a minimum oxygen flow at all times, typically about 175 mL/min. Some machines allow for air alone, which contains 21% oxygen, to be administered without any minimum oxygen flow. Another safeguard required by the standards organizations is an interlock between the oxygen and nitrous oxide flowmeters, which ensures a ratio of gases that cannot provide less than 21% oxygen in the fresh gas. All anesthesia machines in use should have this feature.
Since the minimum concentration of oxygen supplied by the flowmeters is always 21%, no matter which gases are used, how then is it possible to provide too little oxygen? The important concept here is that the concentration of oxygen, and the total amount of oxygen, are two different things. If 21% oxygen is supplied, but the total amount of oxygen is less than the patient’s oxygen consumption, then the concentration of oxygen in the breathing circuit will diminish. Consider, for example, a mixture of oxygen and nitrous oxide of 300 mL/min and 700 mL/min, respectively. This would be a minimal flow approach, whereby the oxygen flow is set to approximate oxygen consumption, and the total nitrous oxide flow would be set to achieve an effective concentration of nitrous oxide. The concentration of oxygen in the fresh gas flow would be 30%. If, however, the patient’s oxygen consumption was greater than 300 mL/min, then insufficient oxygen would be supplied. The result would be a gradual decrease in the inspired oxygen concentration in the breathing circuit as the patient consumed more oxygen than was being supplied, leading ultimately to hypoxemia.
This observation underscores the importance of the last safeguard to adequate oxygen delivery—continuous monitoring of inspired oxygen concentration. This safeguard is mandated by both anesthesia machine design standards and patient monitoring standards. Every anesthesia machine must be equipped with the capability to monitor the inspired oxygen concentration delivered to the patient. Alternatively, one can use a separate monitoring system or device to provide that capability. In any event, a functioning inspired oxygen monitor must be used during every anesthetic procedure. Not only will this device facilitate a minimal fresh gas flow technique and confirm adequate oxygen delivery, but it will also ensure that the gas actually delivered to the anesthesia machine from the pipeline or cylinder is indeed oxygen.
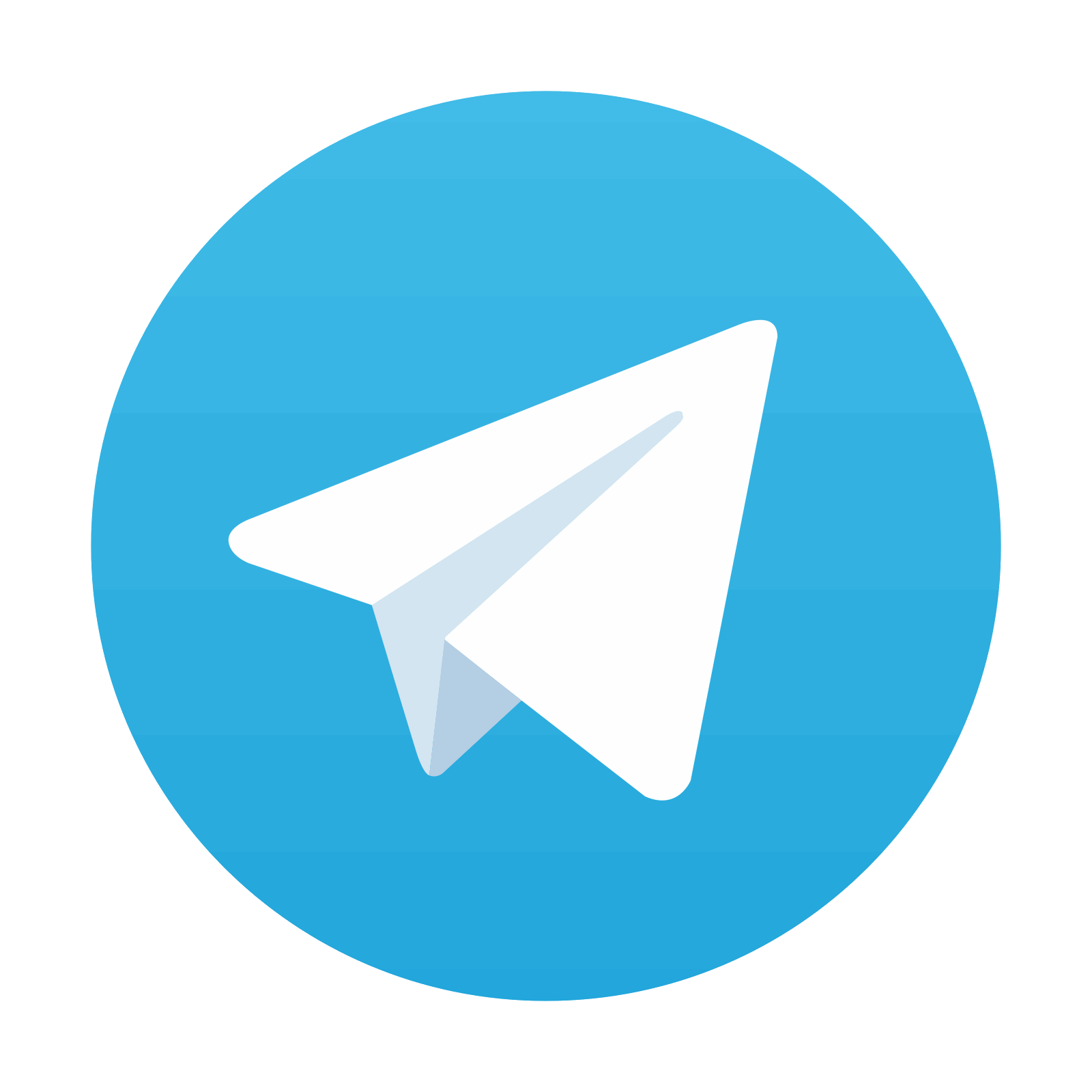
Stay updated, free articles. Join our Telegram channel

Full access? Get Clinical Tree
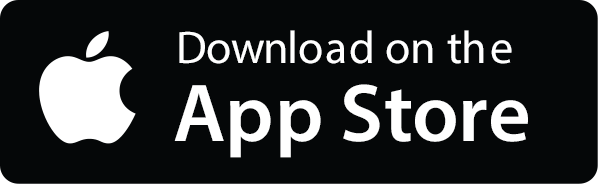
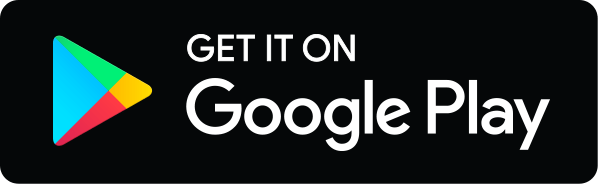