Chapter Outline
Noninvasive Oxygen Delivery 72
Nasal Cannulas and Simple Face Masks 73
Continuous Positive Airway Pressure (CPAP) 76
Masks 77
Oropharyngeal and Nasopharyngeal Airway 80
Laryngeal Mask Airways(LMA) 82
The Laryngeal Mask Airway (LMA) 82
Other Non-intubating Airway 87
Conclusion 89
One of the most important interventions the anesthetist makes for his or her patient is to secure and maintain a patent airway. The anesthetist delivers therapeutic oxygen and maintains ventilation via this airway. This chapter will review a variety of devices used above the glottic opening to supply oxygen and other inhalational gases/agents and to maintain airway patency.
Supraglottic airway devices are simple to use and have qualitatively changed the practice of anesthesiology. However, a supraglottic airway device is commonly considered to be less secure because the larynx, trachea, and distal airways are not protected by the inflated cuff of an endotracheal device. Laryngospasm and active or passive aspiration of gastric contents are always possible when using the supraglottic devices discussed within this chapter.
Supraglottic airways have also changed the considerations when approaching unusual airway situations. For example, the prone position poses additional concerns about maintaining the supraglottic airway, yet these devices are easy to insert in the prone position. Supraglottic airway devices have a place in difficult airway management. Any patient with a known or suspected difficult airway requires the utmost diligence, planning, and back-up planning, and equipment and personnel readily available should an airway emergency occur. Part of this planning is to ensure that supraglottic devices are available. Moreover, the supraglottic airway is a first-line alternative when unrecognized airway problems are encountered.
A recent trend in supraglottic airway usage is to employ the devices in patients traditionally considered to have a full stomach, and hence to be at risk for regurgitation and aspiration. There exists debate whether or not cricoid pressure should be applied during the insertion of a supraglottic airway device in such a patient with full-stomach precautions. Cricoid pressure impedes insertion of laryngeal mask airways and similar devices. Once inserted, these devices do not continue to protect the airway from gastric aspirate because they do not have endotracheal cuffs.
Supraglottic devices for oxygen delivery and airway maintenance are one area in anesthesiology where innovation and invention are rapidly changing practices. Thus this chapter serves as a starting point, but the reader is encouraged to follow the primary literature for the latest developments in practice.
Noninvasive Oxygen Delivery
Oxygen delivery systems are commonly divided into variable and fixed performance devices. The F io 2 provided to the patient with variable performance devices can fluctuate and is sometimes difficult to predict. However, arterial blood gas sampling, along with oxygraphy is a possible method for quantifying oxygen delivery to the patient when using any device. The delivered F io 2 changes with a number of factors ( Table 6–1 ), mainly those associated with equipment and the patient.
Equipment: Oxygen flow rate, mask reservoir volume, quality of fit, hole number, and size/area |
Patient: Respiratory rate, tidal volume, peak inspiratory flow rate |
Other: Presence of other gases (i.e., water vapor from humidification, rebreathed CO 2 ) |
Understanding the interplay between these factors is key to understanding how variable performance devices function. Here, we will use a simple adult face mask as an example. A simple adult face mask holds a small volume of about 200 cc. The mask is continuously supplied with oxygen and fills when the patient is between inspiration and expiration. Oxygen can leak from the holes of the mask and from an imperfect seal at the face. As the patient inhales, the oxygen-rich air is first to enter the airway. As the patient continues to inhale, room air then enters the gas mixture through holes in the mask. This entrained room air reduces the delivered F io 2 . Therefore, in this variable performance system, the F io 2 delivered to the patient is increased when the mask is tightly fitted, and with high oxygen flow rates-in a patient with a low peak inspiratory flow rate, tidal volume and respiratory rate. During this process, there is often some degree of CO 2 rebreathing.
The design of the variable performance device can be changed to increase the F io 2 delivered to the patient, for example, by increasing the reservoir size. The above mentioned face mask used a 200 cc volume of oxygen—in other words, the reservoir size for oxygen was 200 cc. Variable performance devices are often categorized by their reservoir capacity ( Table 6–2 ).
Reservoir Capacity | Volume (cc) | Example |
---|---|---|
Zero capacity | 0 cc | Nasal cannulas |
Low capacity | 70-100 cc | Tracheostomy mask, pediatric face mask |
Medium capacity | 100-250 cc | Adult face mask |
High capacity | 250-1500 cc | Face mask with reservoir bag |
Very high capacity | >1500 cc | Pediatric incubator, headbox, tent Oxygen bar/surgery bar under surgical drapes |
Nasal Cannulas and Simple Face Masks
Zero Capacity Variable Performance Devices—Nasal Cannulas
Nasal cannulas (NC) may be classified as a zero capacity device because there is no built-in oxygen reservoir. The “reservoir” is the volume of the patient’s nasopharynx and is best used when the patient is not mouth breathing. However, there is some debate about this, as mouth breathing may still allow for inhalation of some oxygen from the nasopharyngeal reservoir. Specifically, “Mouth breathing causes inspiratory air flow. This produces a Venturi effect in the posterior pharynx entraining oxygen from the nose.”
NC consist of plastic tubing, two firm 1 cm plastic prongs which seat in the nose, and are held to the face by an adjustable strap, which wraps around the patient’s ears. Oxygen is usually not humidified when delivered by NC.
NC are typically used with an oxygen flow rate of 2 to 4 L/min to allow for an F io 2 of about 0.26 to 0.36. High flow rates are uncomfortable because they dry and irritate (or can even damage) the nasopharynx. Table 6–3 lists pros and cons of nasal cannulas.
PROS |
Less claustrophobic than face masks |
Allow access to the face (i.e., for eating, speaking, brushing teeth, etc.) |
Convenient for home usage |
Improved patient compliance |
CONS |
Very widely variable delivery (i.e., volume of patient’s nasopharynx, seating of prongs in patient’s nose, patient’s respiratory pattern, etc.) |
Prong discomfort |
Drying or damage to the nasal mucosa |
Dilution of delivered F io 2 by ambient air via nostrils and during mouth breathing |
Blocked nasal passages prevent oxygen from entering nasopharynx |
Risk for surgical fire, especially if 100% F io 2 delivered under surgical drapes |
Technically challenging to quantitate end tidal CO 2 during NC use, however, devices for this purpose are being studied and developed, and some are commercially available |
A nasal sponge-tipped catheter ( Figure 6–1 ) may be used to enhance the reservoir produced by NC oxygen delivery. The nasal catheter is a single lumen device that is placed into one nostril and held in place by a spongy foam cuff. The catheter is then taped to the patient’s face—there are no straps. This device “should not be used when a nasal mucosal tear is suspected because of the risk of surgical emphysema.”

New zero capacity variable performance devices are being developed and tested to improve patient comfort and safety. For example, the OxyArm, ( Figure 6–2 ) which is shaped like a cellular phone headset, does not contact the patient’s face and allows for greater comfort. It produces an “oxygen cloud” by the nose and mouth. At oxygen flow rates of 2 to 4 L/min, 0.28 to 0.35 F io 2 can be administered.

Medium Capacity Variable Performance Device—Simple Face Mask
Simple adult face masks ( Figure 6–3 ) are considered medium capacity devices because they have a reservoir size of 100 to 250 cc, which covers the nose and mouth.

These masks are made of soft, clear plastic materials that are secured to the patient’s face with an adjustable elastic strap, which fits around the head. Many of these masks include a bendable metal piece over the bridge of the nose, which can be used to improve mask fit. The body of the mask contains an entry for oxygen supply tubing, and holes which help prevent mask collapse around the face during inspiration, and allow for exchange of ambient, exhaled, and excess gases.
Simple face masks deliver 2 to 6 L/min of oxygen, allowing for a widely variable F io 2 . However, “a typical example of 4 L/min of oxygen flow delivers an F io 2 of about 0.35 to 0.40, providing there is a normal respiratory pattern.” Oxygen flow rates below 2 L/min (or a very high minute ventilation) makes for excessive rebreathing of CO 2 ; flow rates above 2 L/min help with ventilation of exhaled gas out of the reservoir through the holes of the mask, especially during respiratory pauses. The total dead space of the mask is about 100 cc, which is usually inconsequential. Large mask bodies and high resistance through the mask holes may increase CO 2 rebreathing.
The F io 2 supplied to the patient by these masks is affected by several factors, including the supplied oxygen flow rate, patient respiratory pattern, and mask fit. Increased oxygen flow rates and tight mask fit will increase the F io 2 . For anxious pediatric patients who cannot tolerate wearing a mask, oxygen can be “wafted” by placing the mask within close proximity, although the F io 2 supplied will decrease as a function of increasing distance. Table 6–4 lists the functional properties and some of the pros and cons of simple face masks.
Lengthened duration of pause between inspiration and expiration → more time for reservoir filling with supplied oxygen |
Low peak inspiratory flow rate → less room air entrained through mask holes |
Decreased minute ventilation/low respiratory rate/hypoventilation → less CO 2 rebreathing |
Patient inspiratory flow rate < oxygen supply rate |
PROS |
Low cost, convenient, easy usage |
Patient comfort |
CONS |
Delivered F io 2 varies widely with oxygen flow rate, mask fit, patient respiration. |
Small reservoir causes most adult patients to breathe ambient air through holes. |
Vented masks allow for spread of infected respiratory droplets. |
Entrainment of ambient or exhaled gases. |
In theory, patients who are dependent on hypoxia or hypercarbia for respiratory drive (i.e., patients with COPD) may lose respiratory drive when using this device. An alternative explanation is that increased F io 2 deranges VQ matching, leading to worsened gas exchange. |
CO 2 rebreathing (may be indicated by patient feeling warmth or humidity during respiration). |
Similar to the simple face mask is the aerosol face tent ( Figure 6–4 ). The face tent is also a variable performance, clear plastic device with adjustable face strap. The bottom is shaped to fit around the patient’s chin, and the device is open at the top. This presumably produces less claustrophobia, easier phonation for the patient, and allows better access to the mouth for food, liquids, and suction. It is also useful in patients recovering from nasal surgery who cannot tolerate pressure on the nose. The oxygen reservoir is slightly larger than that of the simple face mask; however, more fresh gas is lost to the environment through the open top (see Figure 6–4 ).

High Capacity Variable Performance Devices—Partial Rebreather Masks
The above discussed medium capacity device, the simple face mask, may be converted to a high capacity device with the addition of a 600 to 1100 cc reservoir bag, increasing the F io 2 delivered to the patient. The F io 2 is increased by virtue of the oxygen supply flow rate and; reservoir oxygen, which is composed of exhaled and supplied oxygen as well as room air. These masks are sometimes referred to as partial rebreather masks, or nonrebreathing masks.
Simple face masks often waste supplied oxygen, especially when the patient’s inspiratory flow rate is below that of the supplied oxygen flow rate and during patient expiration. Nonrebreather masks help prevent this by filling with directly supplied and exhaled oxygen.
Reservoir bags are preferred to increasing mask size, which can increase CO 2 rebreathing, and require increased supplied oxygen flow rates to inefficient amounts (greater than 60 L/min) when attempting to provide an F io 2 near 100%. Rather, these partial rebreather masks “probably deliver an F io 2 between 0.75 to 0.90 at oxygen flow rates of 12 to 15 L/min.”
CO 2 rebreathing is further decreased with partial rebreather masks with the addition of flap valves over the holes of the mask’s body ( Figure 6–5 ). These allow for vented gases to enter the environment and help to prevent entrance of these gases and ambient air into the system. Patients will preferentially breathe oxygen from the large reservoir bag. However, a poorly fitted mask allows ambient air to flow around the edges of the device to dilute the supplied oxygen.

Additional features sometimes associated with nonrebreather masks are one-way valves seated between the oxygen reservoir bag and the body of the mask itself. This valve decreases CO 2 rebreathing and allows the patient to preferably inhale oxygen from the reservoir bag. The addition of this valve requires a higher supplied oxygen flow rate because exhaled oxygen from the patient and oxygen from the mask body are vented into the environment.
Fixed Performance Oxygen Delivery Devices: Venturi Masks
Fixed performance devices provide a more consistent and predictable F io 2 to the patient. The most common such device is the venturi oxygen mask ( Table 6–5 ).
Goal is to provide a known F io 2 to the patient who is dependent on their hypoxic/hypercarbic drive (i.e., during a COPD exacerbation to preserve respiratory drive) |
Calculation of the Pa O 2 / F io 2 ratio to determine if a patient requires endotracheal intubation with mechanical ventilation to diagnose acute respiratory distress syndrome or respiratory failure |
When prescribing an exact F io 2 for oxygen therapy, rather than flow rate, to a hospitalized or critically ill patient |
Providing a known F io 2 in the setting of a patient with a varied or unstable respiratory pattern |
Venturi masks consist of a clear plastic mask, similar to the simple adult face mask detailed in the prior section, and a plastic venturi device ( Figure 6–6 ). The venturi device itself fits between the oxygen supply tubing and body of the mask ( Figure 6–7 ). Each is color coded and has listed on it an oxygen flow rate that is needed to achieve a given F io 2 . Each venturi device allows for a certain flow of air through open windows in the device and supplied oxygen through tubing.


It is important to understand how venturi devices work so they can be optimally used. These devices make use of the Bernoulli effect, which can be stated mathematically:
P + 1 2 ( ρ ν 2 ) = κ
where P = pressure, ρ = density, ν = velocity, and к = constant. Applying this principle to the venturi mask—as supplied oxygen flows through a fixed space within the device and into a larger chamber, velocity and kinetic energy of the oxygen increase distally. Because the total energy must be constant, the potential energy in the system simultaneously decreases. This creates a negative pressure gradient, causing room air to mix with the supplied oxygen in the venturi device in a predictable fashion. This allows for a fixed and constant F io 2 to be delivered to the patient. Larger air entrainment windows are needed for more room air to mix, so that the delivered F io 2 is decreased relative to venturis with smaller windows.
During the design and manufacturing of the venturi, these devices are calibrated such that a known oxygen flow rate and air entrainment window size will provide a known F io 2 . Large oxygen and total gas flow rates are used in this system. No rebreathing can occur because both the supplied fresh gas flow rate exceeds the patient’s peak inspiratory flow rate, and holes in the mask allow the fresh gas to displace expired CO 2 and other waste and ambient gases.
Most commonly, the clear masks are fitted with plastic conduits and venturi devices are packaged separately. The devices are manually assembled by linking together the venturi, conduit, and mask. This allows the F io 2 to be changed by changing just the venturi. Alternatively, some vendors have designed single use masks with preattached venturi devices. These devices typically give overall flow rates of approximately 60 L/min, with each requiring a particular oxygen flow to give a fixed F io 2 (usually 24%, 28%, 31%, 35%, 40%, and 60%). See Table 6–6 . Similar previously “calibrated variable venturi devices can be used to deliver the desired F io 2 ” as well ( Table 6–7 ).
F io 2 Provided | Oxygen Flow to Venturi | Air Entrained | Total Flow to Patient |
---|---|---|---|
(%) | (L/Min) | (L/Min) | (L/Min) |
0.24 | 2 | 51 | 53 |
0.28 | 4 | 41 | 45 |
0.31 | 6 | 41 | 47 |
0.35 | 8 | 37 | 45 |
0.40 | 10 | 32 | 42 |
0.60 | 15 | 15 | 30 |
PROS |
See indications table. |
Low cost, disposable device. |
Simple to use, robust to use-error. |
CONS |
Delivered F io 2 on average is 5% above desired F io 2 . |
Highest F io 2 venturi device may underperform by 5%-10%. |
Most reliable when delivering low F io 2 with more air entrapment. |
Mask is cumbersome, noisy, and uncomfortable. |
Continuous Positive Airway Pressure (CPAP)
Continuous positive airway pressure (CPAP) and bilevel positive airway pressure (BiPAP) are both modes of noninvasive ventilation (i.e., not involving placement of an endotracheal tube and mechanical ventilation). They are used in several clinical situations, most commonly in the intensive care unit (ICU) for hospital applications. These include therapy for obstructive sleep apnea (OSA) both in hospital and at home, recovery from general anesthesia or ventilator weaning, acute respiratory failure, or other respiratory emergencies (i.e., COPD exacerbation, pulmonary edema, etc.).
As the name implies, CPAP provides a constant positive pressure to the airway in an effort to prevent airway collapse, and increasing the patient’s functional residual capacity. CPAP valves deliver a set amount of positive pressure, regardless of respiratory cycle phase, in the range of 2.5 to 20 cm H 2 O. On the other hand, BiPAP improves ventilation by decreasing the pressure provided during expiration, facilitating CO 2 release ( Table 6–8 ). The anesthetist should check that the CPAP valve is continuously functioning during the entire respiratory cycle. “It is important to check that the CPAP valve is being kept open by an adequate flow throughout the respiratory cycle.”
PROS |
Spares the patient invasive airway device and mechanical ventilation. |
Can be used as temporizing measure to avoid intubation if the patient’s primary problem is expected to improve. |
Can be used at home for treatment of OSA. |
Oxygen can be humidified for patient comfort. |
CONS |
CPAP/BiPAP may suffocate patient if fit and settings are inappropriate. |
BiPAP must allow for ventilation of CO 2 via loose fit. |
Exact mask fits difficult to achieve. |
Patients often noncompliant because of comfort and noise, especially during sleep. |
Water condensation in tubing may make nasal CPAP less reliable. |
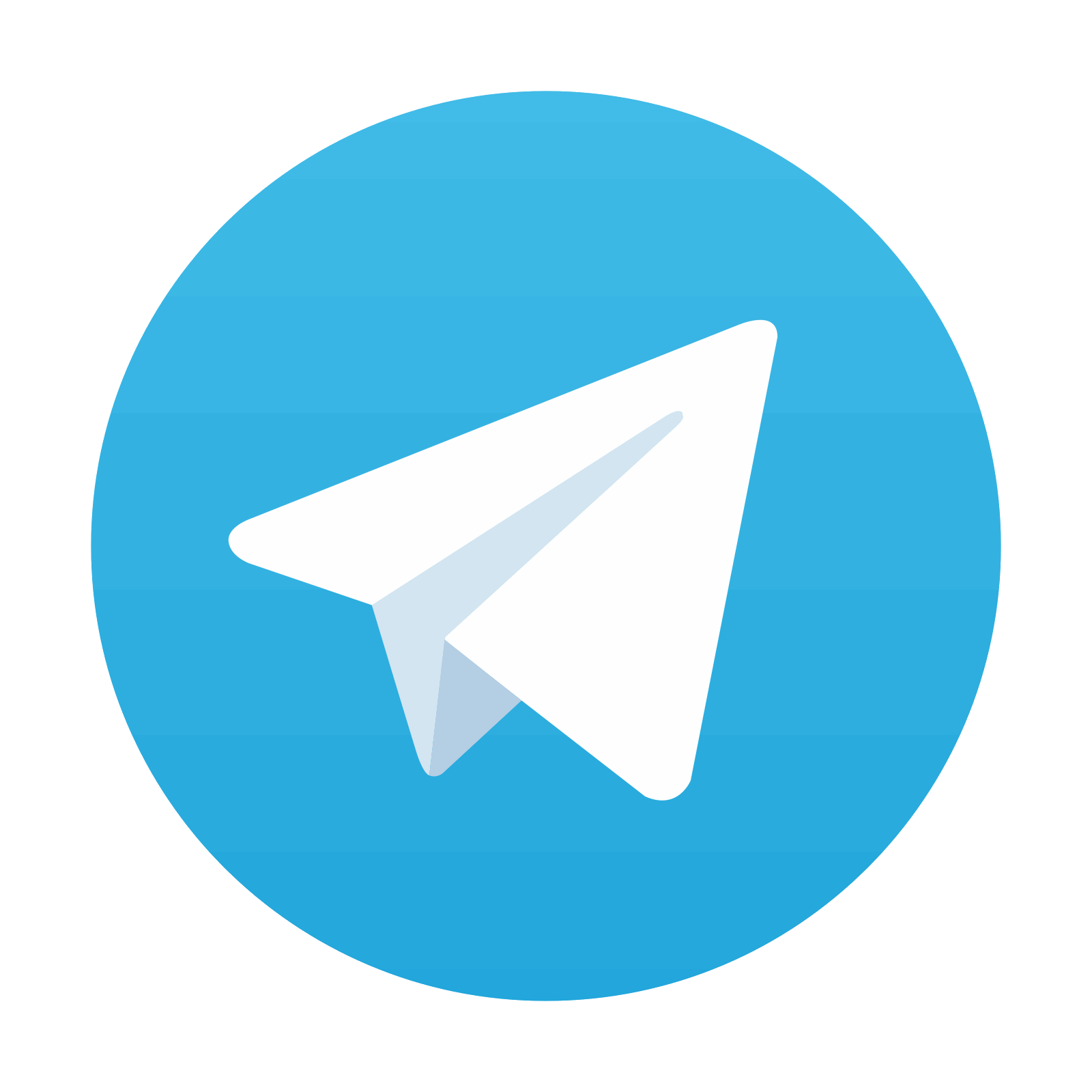
Stay updated, free articles. Join our Telegram channel

Full access? Get Clinical Tree
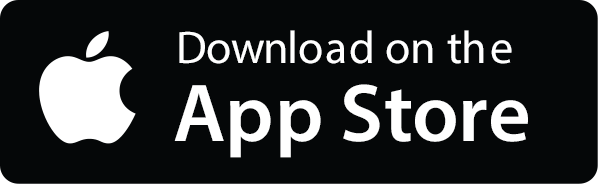
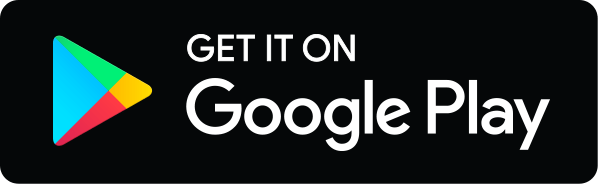
