Chapter 39
Anesthesia for Ophthalmic Procedures
Ophthalmic Anatomy
The eye is surrounded by six extraocular muscles (Figures 39-1 through 39-3). The superior rectus muscle, located at the 12-o’clock position on the globe, moves the eye upward, or supraducts the eye. The inferior rectus muscle, located at the 6-o’clock position on the globe, moves the eye downward, or infraducts the eye. The medial rectus muscle, located 90 degrees medially to the 12-o’clock position on the globe, moves the eyeball nasally, or adducts the eye. The lateral rectus muscle, located 90 degrees laterally to the 12-o’clock position on the globe, moves the eyeball laterally, or abducts the eye. The superior oblique muscle, located on the superior aspect of the eye, rotates the eyeball on its horizontal axis toward the nose, or intorts the eye and depresses the eyeball. The inferior oblique muscle, located on the inferior aspect of the globe, rotates the eyeball on its horizontal axis temporally, or extorts the eye and elevates the eyeball (Table 39-1).
TABLE 39-1
Orbital Muscles and Innervation
Muscle | Function | Cranial Nerve |
Superior rectus | Supraduction | III |
Inferior rectus | Infraduction | III |
Medial rectus | Adduction | III |
Lateral rectus | Abduction | VI |
Superior oblique | Intorsion Depression | IV |
Inferior oblique | Extorsion Elevation | III |
All the ocular muscles except the inferior oblique originate in the orbital apex around the annulus of Zinn (Figure 39-4), which is a fibrinous ring that encircles the optic foramen. The four rectus muscles move forward in a conal pattern that forms the muscle cone around the globe. These muscles, which are about 40 mm long, insert into the globe just anterior to its equator.1 The superior oblique muscle arises just superior to the annulus of Zinn and moves forward, becoming a tendon. This tendon passes through a cartilaginous ring called the trochlea, which is located on the medial supranasal orbital wall. After passing through the trochlea, the tendon is redirected in a posterolateral direction and inserts on the superolateral aspect of the globe under the superior rectus muscle. The inferior oblique muscle originates from the anterior nasal orbit and moves in a posterolateral direction to the globe, inserting along the lateral aspect of the globe. The arching of both the inferior and the superior oblique muscles around the globe allows for the torsional movements of the eye.
Eyelid Muscles
The levator muscle of the upper eyelid is the primary muscle used for raising the upper eyelids. This muscle originates near the annulus of Zinn (see Figure 39-1, B). It moves forward just superior and slightly medial to the superior rectus muscle, inserting into the upper eyelid. Because the levator muscle only retracts and does not contract the eyelid, akinesia of this muscle is not necessary.
The orbicular muscle of the eye (Figure 39-5) causes the eyelids to contract. This muscle has three divisions—orbital, palpebral, and tarsal—which are concentrically arranged around the eyelid. Akinesia of these muscles is generally desired for ocular procedures, because if the muscles were allowed to contract around the globe, intraocular pressure would increase. However, the recent success of cataract and glaucoma procedures performed with the use of topical and subconjunctival anesthesia has demonstrated that akinesia of the orbicular muscle of the eye is not always mandatory.
Cranial Nerves
The orbital portion of the optic nerve (cranial nerve II) (Figure 39-6) is from 25 to 30 mm long and travels posteriorly within the muscle cone from the globe into the cranial cavity. This distance is actually longer than that from the posterior portion of the globe to the orbital apex, giving the optic nerve an S-shaped configuration. This shape allows free movement of the nerve so that the many positions of the eye are accommodated. The optic nerve is myelinated and is about 4 mm in diameter.2 The optic nerve extends through the optic canal and continues until it meets the optic chiasm intracranially. The optic chiasm is the junction of both optic nerve tracts. Here, suspended in and surrounded by cerebrospinal fluid, the optic nerve fibers partially decussate, sending visual fibers to the contralateral eye.
The optic nerve is not a true cranial nerve but is actually an outgrowth of the brain.1 As a result, the optic nerve is also covered by the meninges, the fibrous wrappings of the arachnoid, dura, and pia mater, which envelop the central nervous system (CNS). Therefore any anesthetic agent injected into the optic nerve sheath can find its way back to the midbrain through the cerebrospinal fluid and result in CNS depression and even lead to respiratory arrest. The optic nerve also carries the central retinal artery and vein into the globe. The central retinal artery and vein exit the optic nerve about 8 to 15 mm posterior to the globe.3
The oculomotor nerve (cranial nerve III) innervates the following muscles of the orbit: the superior rectus muscle, the inferior rectus muscle, the inferior oblique muscle, the medial rectus muscle, and the levator muscle of the upper eyelid. The oculomotor nerve is the primary motor nerve to the extraocular muscles of the orbit; this nerve branches superiorly and inferiorly (Figure 39-7). The superior branch innervates the superior rectus muscle and the levator muscle of the upper eyelid. The inferior branch of the oculomotor nerve innervates the medial rectus muscle, the inferior rectus muscle, and the inferior oblique muscle. This nerve also sends parasympathetic fibers to the ciliary ganglion (Figure 39-8), which is located adjacent to the optic nerve in the posterior portion of the orbit. The ciliary ganglion receives parasympathetic fibers from the oculomotor nerve and also sympathetic fibers from the carotid artery plexus and a sensory branch from the nasociliary nerve, a branch of the ophthalmic nerve. The parasympathetic fibers move from the ciliary ganglion forward to innervate the iris sphincter muscles, which cause constriction of the pupil. The sympathetic motor fibers move forward to control the radial muscle of the iris for pupillary dilation.1–3
The trochlear nerve (cranial nerve IV) (see Figure 39-7) provides the motor fibers for the superior oblique muscle. This nerve enters the orbit through the superior orbital fissure outside the muscle cone. It is the only orbital cranial motor nerve that enters the orbit from outside the muscle cone. Once inside the orbit, the nerve root moves in a medial direction to innervate the superior oblique muscle.
The trigeminal nerve (cranial nerve V) (see Figure 39-8) has sensory and motor components. In ocular anesthesia, the sensory component is of primary importance. The intracranial portion of the nerve forms the trigeminal ganglion, which has three main divisions: the ophthalmic, the maxillary, and the mandibular nerves. The ophthalmic branch provides for the sensation of pain, touch, and temperature to the cornea, ciliary body, iris, lacrimal gland, conjunctiva, nasal mucosa, eyelid, eyebrow, forehead, and nose. The maxillary branch provides for the sensation of pain, touch, and temperature to the upper lip, nasal mucosa, and scalp muscles.4
The ophthalmic nerve has three main branches: lacrimal, frontal, and nasociliary. The lacrimal nerve branch innervates the lacrimal gland in the superior lateral aspect of the orbit. The frontal branch is the largest branch of the ophthalmic nerve. This branch enters the orbit from outside the muscle cone through the superior orbital fissure and travels anteriorly outside the muscle cone superior to the levator muscle. The frontal nerve itself splits into two branches. The larger, supraorbital branch continues forward into the orbit and exits the orbit through the supraorbital notch; this branch innervates the forehead. The smaller branch is the supratrochlear nerve, which moves in a medial direction, supplying nerve roots to the forehead and the medial portion of the upper eyelid. The nasociliary nerve branch enters the orbit from inside the muscle cone and crosses over the optic nerve, sending nerve fibers medially and to the ciliary ganglion. The fibers to the ciliary ganglion form the short ciliary nerves, which continue anteriorly, penetrating the posterior portion of the globe near the optic nerve. The nasociliary nerve also gives rise to the long ciliary nerves, which continue anteriorly and enter the posterior portion of the globe supplying the ciliary muscle, iris, and cornea. The long ciliary nerves also carry sympathetic fibers to the dilator muscle of the iris from the superior cervical ganglion. The nasociliary nerve continues along the medial aspect of the orbit just superior to the medial rectus muscle until it passes through the orbital septum to become the infratrochlear nerve. The infratrochlear nerve provides sensory input to the side of the nose, the medial aspect of the eyelids, the medial conjunctiva, the caruncle, and the lacrimal sac.1–4
The abducens nerve (cranial nerve VI) (see Figure 39-7) provides motor function to the lateral rectus muscle. The nerve enters through the superior orbital fissure within the muscle cone and continues along the conal surface of the lateral rectus muscle, eventually inserting in the posterior one third of that muscle.
The facial nerve (cranial nerve VII) (Figure 39-9) is predominantly a motor nerve for the muscles of the face. This nerve exits from the stylomastoid foramen. The facial nerve travels underneath the external auditory canal to the parotid gland, where it divides into an upper and a lower branch. Ocular anesthesia is more concerned with the upper branch of the facial nerve than with the lower. The upper branch further divides into the temporal and zygomatic branches, which innervate the orbicular muscle of the eye, the superficial facial muscles, and the scalp muscles.
Orbital Fossa
The orbital fossa has been described as pear shaped. The medial walls of the orbit extend almost straight back, whereas the lateral walls diverge at about a 90-degree angle to each other (Figure 39-10). The superior and inferior orbital fissures are in the orbital apex, which is located in the posterior orbit. These fissures are the entry portals for the orbital nerves and vessels (Figure 39-11). The optic foramen lies just medial to the superior orbital fissure and is the entry portal for the optic nerve and the ophthalmic artery from the intracranial to the intraorbital area. In the medial nasal aspect of the fossa, just behind the orbital rim, is the lacrimal bone, which is used as a landmark for the medial peribulbar block (Figure 39-12). The ethmoid bone is just posterior to the lacrimal bone.4
The supraorbital nerve exits the orbit in the supraorbital notch, which is in the superior nasal aspect of the orbital rim. The infraorbital foramen, where the infraorbital nerve and artery exit, is just below the infraorbital rim at about the 6-o’clock position. The infraorbital nerve is the sensory branch of the maxillary nerve. The lacrimal, frontal, and trochlear nerves all enter through the superior orbital fissure outside the muscle cone. The oculomotor, abducens, and nasociliary nerves all enter the orbit from inside the muscle cone.4
The ophthalmic artery (Figure 39-13), which is the first branch of the internal carotid artery, passes into the orbit through the optic canal. The ophthalmic artery usually lies just inferolateral to the optic nerve. The artery extends along the optic nerve for a short distance, crossing over it in most cases and continuing medially.5 The first branch of the ophthalmic artery is usually the central retinal artery. The central retinal artery moves in an anterior direction underneath the optic nerve, usually entering the optic nerve on its inferomedial side 8 to 15 mm posterior to the globe.3 The artery continues forward into the optic nerve head and branches into the retinal arteries. The ophthalmic artery gives rise to the long and short posterior ciliary arteries. The short posterior arteries move anteriorly and divide into many small branches that penetrate the globe close to the optic nerve and supply the choroid and the optic nerve head. The ophthalmic artery also provides branches to the optic nerve. The orbital branches of the ophthalmic artery include branches to the supraorbital arteries, the rectus muscles, and the lacrimal gland.4
The dorsal nasal artery is one of the terminal branches of the ophthalmic artery. It exits the orbital septum above the medial canthal tendon and joins with the angular artery, thus establishing communication between the internal and external carotid arteries.1 The external carotid artery gives branches to the facial artery (the external maxillary artery). The facial artery originates near the angle of the mandible, extends toward the stylohyoid muscles, and then proceeds forward to the lower border of the mandible. The artery then turns upward and moves toward the nose, where it joins with the dorsal nasal artery in the medial canthal area. The inferior orbital fissure is the entrance site for the infraorbital artery. This artery moves anteriorly through the infraorbital canal and exits to the face through the infraorbital foramen.
The venous drainage system (see Figure 39-13) for the orbit includes the superior and inferior ophthalmic veins, which drain into the cavernous sinus that is located intracranially. Radiographic studies have demonstrated a unique characteristic of the orbital vascular system: the orbital veins are independent of the orbital arteries.5 The venous system of the orbit is valveless, and blood flow in this area is determined by pressure gradients. The primary vein of the orbit is the superior ophthalmic vein. This vein travels posteriorly to the medial side of the superior rectus muscle, then beneath the superior rectus muscle inside a support hammock. The vein then emerges on the muscle’s lateral aspect. The vein continues its posterior direction along the lateral aspect of the superior rectus, exiting the orbit through the superior orbital fissure, and terminating in the cavernous sinus.5
Several veins enter into the superior ophthalmic vein: the ciliary veins, the lacrimal veins, and the superior vortex veins, which are located on the posterior quadrants of the globe and drain the choroid, or second layer, of the globe. The inferior ophthalmic vein originates from a diffuse plexus on the floor of the orbit. This vein receives several branches, including the extraocular muscles and the inferior vortex veins located on the inferoposterior quadrants of the globe. The primary branch of the inferior ophthalmic vein also drains into the superior ophthalmic vein before the entrance of the superior ophthalmic vein into the cavernous sinus. The central retinal vein exits the globe inside the optic nerve. The central retinal vein then exits the optic nerve and enters the orbit between 8 and 15 mm posterior to the globe3 and usually passes directly to the cavernous sinus.1
Orbit
An evaluation of the patient’s orbit and globe size is important before ocular anesthesia is conducted. The usual volume of the orbit is 30 mL (Box 39-1). The volume of a typical globe (which has a diameter of about 25 mm) is 6.5 to 7 mL.5 The balance of the orbital volume is approximately 23 mL and is composed of muscles, vessels, nerves, and fat. Katsev et al.6 measured 120 orbits from 60 adult skulls and found an average orbital depth of 48 mm. The distance from the middle third and lateral third of the infraorbital rim to the superior aspect of the optic foramen was also measured and ranged from 42 to 52 mm. This distance should not be confused with the depth of the orbital floor. Because of the pear shape of the orbit, the orbital floor does not extend directly to the orbital apex. The orbital floor extends only to the posterior wall of the maxillary sinus, about two thirds of the depth of the orbital apex.
The orbital septum is a fibrinous tissue that defines the anatomic anterior boundary of the orbit and keeps the adipose tissue from protruding forward. The visual axis (also known as the optic axis or the geometric axis) is an imaginary line from the midpoint of the cornea (anterior pole) to the midpoint of the retina or macula (posterior pole) (Figure 39-14). The horizontal (anteroposterior) diameter of the globe is an important consideration for ocular blocks. This measurement of the visual axis is referred to as the axial length. The axial length is measured preoperatively to determine the appropriate intraocular lens that should be placed in the eye after cataract removal. The axial length of the globe can be used only when measurements for intraocular lens implants are performed by the ophthalmologist. Normal axial lengths range from 23 to 23.5 mm. In the hyperopic (farsighted) eye, the globe is less than 22 mm long. This shorter eye length may allow a little more working area behind the eye during an ophthalmic block; however, this advantage may be offset by a smaller overall orbit.4,6
The main concern regarding ophthalmic blocks involves the longer, myopic (nearsighted) eye, whose axial length is greater than 24 mm. As the globe stretches, it is believed that the fibrinous scleral layer thins, making the globe easier to penetrate by the needle. This increased posterior length of the globe also increases the chance of globe puncture. Therefore, because of a greater chance of contact in the posterior aspect of the orbit, the axial length of the eye (if this measurement is available) should be considered in the planning for ocular block. If the axial length is unknown, which may be the case in glaucoma surgery, corneal transplants, retinal procedures, or muscle surgery, the practitioner’s preoperative questions should include a history of nearsightedness or previous retinal procedures.
The separate coats, or tunics, of the eyeball (see Figure 39-14) start with the sclera, which is the outer, fibrinous protective layer. The sclera is white and opaque and lies just posterior to the cornea. The cornea is the outer, fibrinous protective layer located anteriorly, and it is transparent and colorless. The middle, or vascular, layer is called the choroid. The retina is the inner layer of the posterior half of the eye. The limbal area is defined as the area at the junction between the cornea and the sclera.1 The conjunctiva is a thin, transparent mucous membrane that covers the posterior surface of the eyelids and the anterior surface of the sclera.
A staphyloma is a bulging of the uvea, which comprises the iris, the ciliary body, and the choroid, into a thin and stretched sclera. Staphylomas may occur in the anterior, equatorial, and posterior areas.2
Tissue Systems of the Orbit
Three connective tissue systems within the orbit have been defined by Koornneef.7 They are the Tenon capsule, the orbital connective tissue, and the fascial sheaths of the extraocular muscles (Figure 39-15).
FIGURE 39-15 A, Lateral view of the orbital connective tissue. B, Superior view of the orbital connective tissue. m., Muscle.
Orbital Connective Tissue
Koornneef demonstrated the presence of connective tissue attachments between both the globe and the periorbital area. The connective tissue begins at the orbital apex and continues anteriorly, becoming more complex and more clearly defined at the level of the globe. Koornneef7 also noted that the tissue septa are in a 360-degree encapsulation of the globe (Figure 39-15). These connective tissue septa encircle and support the globe within the bony orbit. Connective tissue septa were also noted between the superior and inferior oblique muscles, the Tenon capsule, the rectus muscles, and the ligaments stabilizing the globe within the orbit. This connective tissue septa meshwork limits displacement of the globe.
Fascial Sheaths
In the posterior orbit, the fascial sheaths of the extraocular muscles are not as well defined as they are immediately behind the globe.8 Koornneef was not able to identify a common muscle cone throughout the orbit (Figure 39-16). The muscle sheaths themselves contribute fibrinous septa to the periorbit; these septa serve as ligaments for the extraocular muscles. These fascial extensions promote the efficiency of the extraocular muscle functions.7–10
Pharmacology: Ocular Medications and Anesthetic Agents
A number of drugs are used in ophthalmology practice including mydriatics, miotics, cycloplegics, antibiotics, anti inflammatory drugs, viscoelastics, and glaucoma therapies. Ocular medications are listed in Table 39-2. The local anesthetics and hyaluronidase are covered in detail in Chapter 10.
TABLE 39-2
Class | Generic Name (Trade Name) | Comments |
α2-Agonist | brimonidine tartrate (Alphagan P) apracionidine (Lopidine) | Glaucoma: Reduces aqueous humor production Contraindicated with MAO inhibitors |
Cholinesterase inhibitors | ecothiophate iodide (Phospholine Iodide) | Glaucoma: Produces miosis by allowing acetylcholine to continually stimulate iris and ciliary muscles, improving uveoscleral outflow of aqueous humor May prolong effects of succinylcholine; however, rarely used |
β-Blockers | timolol (Timoptic) levobunolol (Betagan Liquifilm) betaxolol (Betoptic) metipranolol (OptiPranolol) carteolol (generic) | Glaucoma: Reduces aqueous humor production Caution in patients with asthma, COPD, heart block, heart failure, and hypotension |
Carbonic anhydrase inhibitors | acetazolamide (Diamox) dorzolamide (Trusopt) brinzolamide (Azopt) | Glaucoma: Reduces aqueous humor production |
Cholinergic agonists | pilocarpine, topical carbachol (Miostat), intraocular acetylcholine chloride (Miochol-E), intraocular | Miotics; used to constrict pupil for surgical procedures |
Cycloplegics | atropine homatropine cyclopentolate | Pupillary dilators; cause temporary paralysis of ciliary muscle and muscles of accommodation |
Intraocular gases | sulfur hexafluoride perfluoropropane | Retinal detachment: Intravitreal insufflation to tamponade retina in place Avoid nitrous oxide for up to 3 months |
Mydriatics | phenylephrine tropicamide epinephrine | Pupillary dilators; cause either a direct or indirect effect on dilator muscle of iris |
Nonsteroidal antiinflammatory agents | flurbiprofen sodium (Ocufen) | Preserves pupillary dilation during surgical procedure by inhibiting prostaglandins, which cause miosis |
Osmotic diuretics | glycerin (oral agent) mannitol | Reduce intraocular pressure |
Prostaglandins | latanoprost (Xalatan) bimatoprost (Lumigan) travoprost (Travatan) tafluprost (Zioptan) | Glaucoma: Promotes uveoscleral outflow of aqueous humor |
Viscoelastics | hyaluronate sodium (Healon, Amvisc) | Protect endothelial cells of cornea during surgical procedures |
COPD, Chronic obstructive pulmonary disease; MAO, monoamine oxidase.
Systemic Absorption of Eye Drops
The lacrimal apparatus includes the lacrimal gland, the puncta, the inferior and superior canaliculus, the common canaliculus, the lacrimal sac, and the nasolacrimal duct (Figure 39-17). The lacrimal gland is located in a depression of the frontal bone in the superior temporal orbit.1–2 The gland has several ducts that lead to the conjunctival surface of the upper eyelid. Tears pass from the lacrimal gland through the ducts, over the cornea and conjunctiva, keeping the eye moist. Near the medial canthus, tears enter the puncta, travel through the canaliculus to the lacrimal sac, and drain into the nasolacrimal duct before entering the nasal mucosa.
• Have the patient close the eyes for 60 seconds after drops are instilled to encourage absorption by the eye and minimize drainage to the nasal mucosa.
• Have the patient avoid blinking, which rapidly moves the medication into the tear outflow canal and the systemic circulation.
• Block the tear outflow canal by placing the index finger over the medial canthus after the eye is closed.2
Select Ocular Anesthesia Techniques
Topical/Intraocular Anesthesia
Cataract and vitreoretinal surgeries are the most frequently performed intraocular surgical procedures.11,12 Topical anesthesia for cataract surgery (e.g., 2% lidocaine) has proved to be effective in providing adequate analgesia for the surgical procedure and is commonly used with phacoemulsification. Topical anesthesia is applied as drops or gels and may be supplemented by intracameral injection by the surgeon for better intraoperative pain control. Vitreoretinal surgery usually requires at least a sub-Tenon block and more frequently injection anesthetic techniques.12 Today’s smaller-incision surgical techniques with foldable intraocular lenses provide a safer surgical experience for the patient and a more rapid recovery. Intraocular anesthesia can further enhance the analgesia for the surgical procedure; lidocaine (preservative free) has been studied and recognized as safe for intraocular administration.13 However, topical anesthesia may not be appropriate in all cases for the surgeon or the patient, because it provides a lesser degree of analgesia and no akinesia of the ocular muscles or eyelids. There is wide variability in operative conditions, sensations, and pain relief, depending on the type of local anesthesia administered for intraocular surgery. Using data that present the strength of evidence as “strong evidence,” “weak evidence,” or “no evidence,” the differences between local/regional anesthetic techniques for variables such as pain (during placement of the block and during the surgery), eye akinesia, eyelid sensation, and visual sensations were quantified on a + or − scale, and the conflicts of evidence are presented as a range in Table 39-3.12
TABLE 39-3
Comparisons of Local/Regional Anesthesia Techniques
From Vann MA, et al. Sedation and anesthesia care for ophthalmologic surgery during local/regional anesthesia. Anesthesiology. 2007;107(3):502-508.
Sub-Tenon Block
The sub-Tenon block will produce a more profound analgesia; however, motor movement of the globe may still be present. The Tenon tissue, as described earlier, encapsulates the globe posteriorly and fuses with the conjunctiva anteriorly. Anteriorly it is inferior to the conjunctiva. The sub-Tenon block is a procedure performed between the rectus muscles of the globe. The conjunctiva is incised, the Tenon tissue is elevated and incised, and a short cannula is inserted into the sub-Tenon space. Local anesthetic is injected with the objective of a posterior spread of the agent. The dose is usually 3 to 4 mL to achieve analgesia; however, larger doses of up to 10 mL have been reported to achieve some degree of akinesia.14
Ocular Regional Anesthesia
The ocular regional needle block still remains the most common and effective way to consistently produce a profound analgesia and akinesia of the eye and eyelids.
Retrobulbar and peribulbar injections are categorized under regional anesthesia methods. These blocks are designed to anesthetize multiple cranial nerves (III, IV, V, VI, and VII). As described earlier, the optic nerve is a continuation of the brain. The dura mater divides at the entrance of the optic nerve into the orbit. The visceral layer of the dura covers the intraorbital part of the optic nerve, and the parietal layer blends into the periosteum of the orbit.1 Therefore, by anatomic definition, this procedure is performed in the orbital epidural space. As has been demonstrated in the anatomic reviews by Koornneef, no true muscle cone exists, especially in the posterior portion of the orbit.7–10 Therefore, old anatomic concepts such as the image of an intact muscle cone must be set aside in favor of concepts that illustrate a communication throughout the orbit.
Techniques and Modifications
The term retrobulbar block refers to an ophthalmic block technique originally described by Atkinson in 1936. The patient is instructed to look up and nasally (supranasal position). A 23-gauge retrobulbar (dull) needle is inserted through the skin in the infratemporal area, just above the inferior orbital rim and advanced toward the orbital apex 35 mm (1.38 inches) deep into the muscle cone (retrobulbar space). After negative aspiration, 2 to 4 mL of anesthetic solution is injected into the muscle cone. After the injection is completed, the eyelids are closed, and digital pressure is applied over the globe to the orbit. A few minutes later, the eyelids are opened, and the globe is inspected for akinesia.15 The popularity of the retrobulbar block for ophthalmic procedures grew, with more than 1 million blocks performed annually in the 1940s.16 Unfortunately, so also did the complication rates.
As a result of the increasing number of complications being reported, practitioners began to alter the Atkinson retrobulbar technique in an effort to increase the margin of safety for ocular anesthesia.17 Three major problem areas in the Atkinson technique are identified in this chapter, and technique modifications are discussed (Figure 39-18).
FIGURE 39-18 Superior view of the parallel and oblique approach to retrobulbar anesthetic blocks. n., nerve.
Eye Position
The primary gaze position, in which the patient is looking directly forward, allows the optic nerve to maintain its S-shaped curvature and also releases the tension on the blood vessels. The down-and-out gaze position allows the optic nerve and vessels to rotate toward the optic foramen and farther away from the needle path. Both of these eye positions have the potential disadvantage of needle visualization by the patient. The upward-gaze position should only be used as described by Gills and Lloyd.16 Their technique allows the use of the upward-gaze position because the needle is placed lateral and parallel to both the optic nerve and the vessels.
Needle Depth
A second problem is the depth of the needle insertion. The vital structures in the ocular anatomy are more crowded in the posterior orbit. Deep needle penetration in the orbit increases the likelihood of trauma to the optic nerve and vessels. If the depth of the needle insertion is decreased to approximately 25 mm (1 inch),17 the needle would lie just posterior to the globe, thereby reducing the risk of puncture of the vital structures. Studies have demonstrated that because of the wide variation in orbital and globe sizes, a needle depth of 19 to 31 mm (0.75 to 1.25 inches) is the safest.6
Needle Tip Shape
A pertinent issue debated in the literature is the use of sharp versus dull needles for ocular blocks. Dull or flat-grind needles made specifically for retrobulbar anesthesia are touted by some clinicians as the only safe needles for use in ocular blocks. It is not so much the type of needle but where the needle is placed that increases the risk.18 Dull retrobulbar needles may not be tolerated as well by awake patients because of the sensation of pressure they create on insertion. Other needles proposed for ocular blocks include a curved retrobulbar needle19 and a dull pinhead needle, in which the injection port is proximal to the head of the needle.20
Needle Angle
Gills and Lloy16 developed a technique that takes into consideration not only the aforementioned changes but also the length and spherical shape of the globe. This technique changes the oblique approach to a parallel approach. The lateral limbic margin, corneoscleral junction, is identified, and the needle is inserted in the inferotemporal area transconjunctivally, just lateral and parallel to the lateral limbic margin. The needle is inserted to a depth of approximately 25 mm (1 inch), entering the muscle cone just behind the globe. The advantages of this technique result from the needle position, which lies lateral and parallel to the optic nerve, the vasculature, and the posterior pole of the globe. However, the technique does not address the question, When does the needle tip pass the equator of the globe? The needle tip must pass the equator of the globe before it may be safely redirected cephalad and inserted into the intraconal space.
• Position the globe to decrease tension on the vital orbital structures and position them farther away from the needle—for example, the primary gaze position.
• Use a depth of needle insertion of about 25 mm (1 inch), which places the needle just behind the globe itself and avoids the structures deep in the orbit.
• Consider using a more lateral to parallel approach to the orbit than was originally demonstrated by Atkinson.
Some practitioners, in an effort to further improve the safety of ocular blocks, have advocated the use of extraconal peribulbar blocks.21
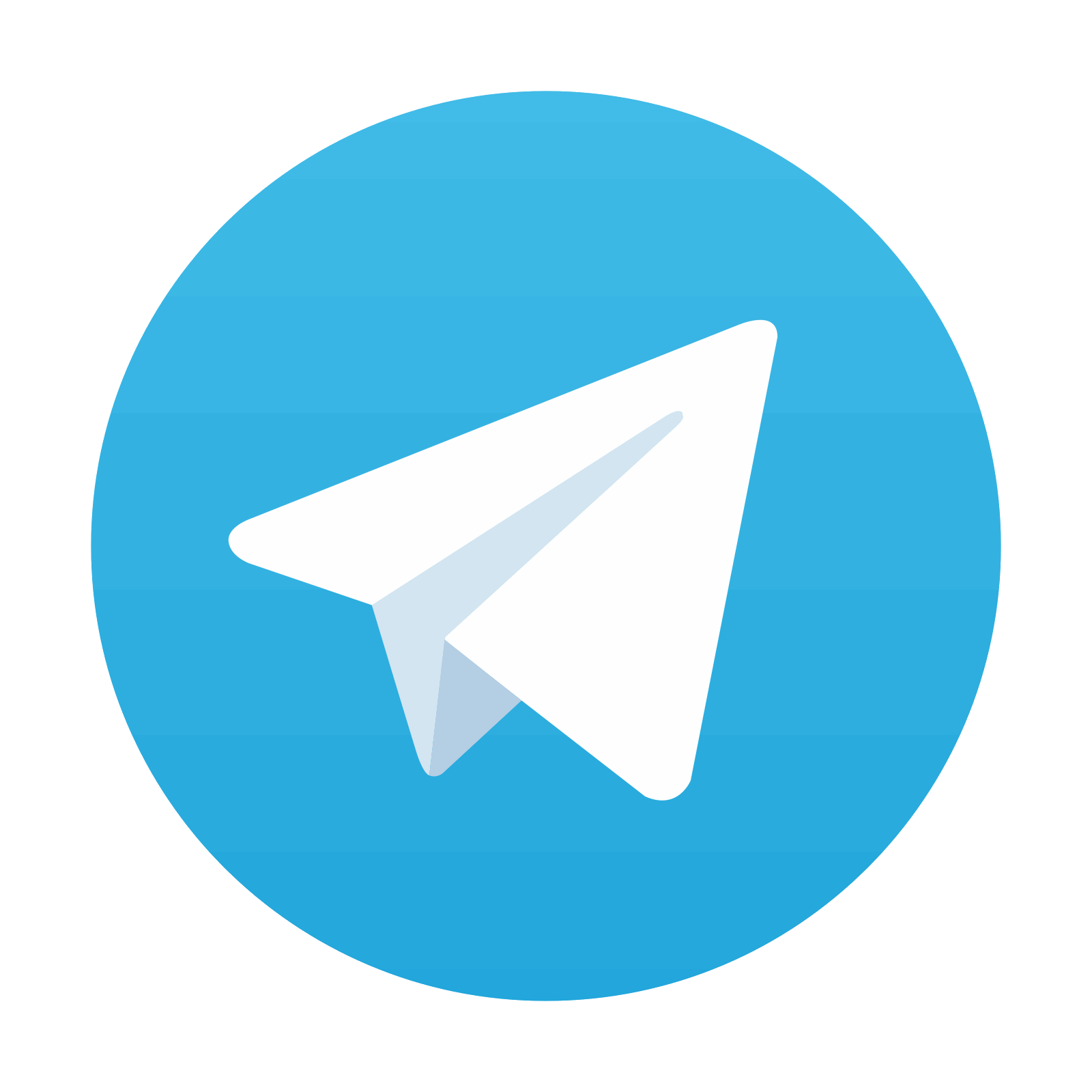
Stay updated, free articles. Join our Telegram channel

Full access? Get Clinical Tree
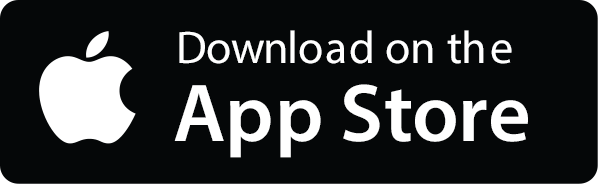
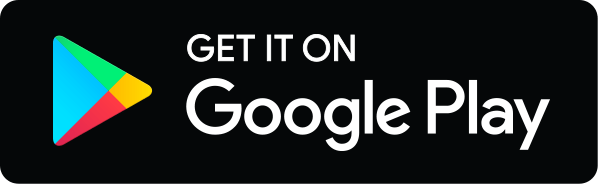