Chapter 31
Anesthesia for Laparoscopic Surgery
The advent and expansion of laparoscopic surgical techniques over the past 30 years has transformed the way in which practitioners approach the care of patients during the perioperative period. Surgeons in gynecology, urology, and general surgery are using laparoscopy to perform increasingly more complex diagnostic and therapeutic procedures (Box 31-1). In fact, in some cases laparoscopic approaches have almost entirely replaced the traditional open laparotomy. These “minimally invasive” surgical procedures offer many advantages over “open” procedures, but have also created unique challenges for the anesthesia community.
Although considered to be a relatively new phenomenon in health care, the origins of laparoscopic surgery date back over 100 years. In 1901 the first endoscopic examination of the peritoneal cavity, known at the time as “celioscopy,” was attempted by the German surgeon George Kelling to evaluate the effects of pneumoperitoneum on intraabdominal hemorrhage associated with such conditions as ectopic pregnancy and bleeding ulcers.1–3 Over the next 70 years several surgeons used laparoscopic techniques, but because of technologic limitations and a high complication rate from bowel and cautery injuries and vascular perforation, few mainstream surgeons embraced the techniques.2 With the development of the open entry Hasson trocar in 1971 and videoscopic imagining in the mid-1980s, the safety of laparoscopy improved. Finally, in 1988 the first videolaparoscopic cholecystectomy was performed by the French surgeon Philip Mouret and the technique soon spread worldwide as the healthcare community began to realize the true benefits of laparoscopy—a safer, less painful, minimally invasive alternative to open laparotomy with a faster recovery and return to normal function.2,4,5
After the success of laparoscopic cholecystectomy, surgeons began to explore minimally invasive approaches to a vast array of complex intraabdominal procedures including herniorrhaphy, prostatectomy, hysterectomy, nephrectomy, and many others. These techniques are now employed by many surgical specialties in patients across the life span and with significant coexisting disease. As a result, the challenges associated with laparoscopy, such as pneumoperitoneum and unique positioning, are coupled with increasingly critical patients adding unique complexity to the anesthetic management for these procedures. Figure 31-1 depicts a classic approach to a laparoscopic appendectomy.
Creation of the Pneumoperitoneum
To perform laparoscopic procedures, it is necessary to create an environment in which the surgeon can clearly view all intraabdominal structures and successfully manipulate the instruments required for surgical dissection. This is accomplished through the creation of an artificial pneumoperitoneum—the installation of air or gas into the peritoneal cavity under controlled pressure. Although complications associated with laparoscopic surgery are rare, initial entry into the abdominal cavity and establishment of the pneumoperitoneum are responsible for a significant proportion of those that do occur.6–9 Large multicenter studies have demonstrated the pooled risk of severe vascular or bowel injury at the time of abdominal entry to be 0.2 and 0.4 per 1000, respectively.6,8 In addition to the risks caused by the placement of the surgical trocars, insufflation of gas into the peritoneal cavity produces significant physical stress on multiple organ systems. These physiologic effects are seen intraoperatively and can carry over into the immediate postoperative period, increasing the morbidity and mortality associated with these procedures.
Two entry methods are used most commonly for the establishment of the pneumoperitoneum during laparoscopic surgery—the closed technique or the open (Hasson) procedure.6,8 Other techniques are available but are used less often because little evidence exists to support their superiority in respect to preventing the complications associated with needle and trocar placement. These techniques include direct entry without prior establishment of the pneumoperitoneum and the use of optical entry trocars.10–12 The choice of technique is determined by the surgeon; however, the evidence indicates that patients who are extremely thin, obese, or known to have abdominal adhesions are at increased risk for laparoscopic entry-related injuries at the umbilical entry point, and they may benefit from an alternative entry procedure such as the open (Hasson) or left upper-quadrant (Palmer’s point) entry technique.6,13,14
The closed technique involves the use of a spring-loaded needle known as a Veress needle to pierce the abdominal wall at its thinnest point, either in the infraumbilical or intraumbilical region. Various different techniques have been used to test for proper placement of the Veress needle including attempted saline aspiration and hanging-drop techniques. However, a review of recent case control and cohort studies of women undergoing laparoscopic procedures has shown that an intraabdominal pressure (IAP) of 10 mmHg or less reliably indicates correct placement of an umbilically placed Veress needle.6,15–17 An appropriate nonflammable gas, usually carbon dioxide (CO2), is then insufflated through the needle to increase the intraabdominal pressure, lift the abdominal wall, and create a space between it and the underlying organs. After insufflation of the abdomen, a trocar is inserted blindly or under direct vision to allow the surgeon to pass instruments into the abdominal cavity.6,7
The open technique was developed by H. M. Hasson in 1970 in an attempt to combine the benefits of laparoscopy with the safety of open laparotomy.18 The technique involves the development of a 1- to 2.5-mm midline vertical incision that begins at the lower border of the umbilicus and extends through the subcutaneous tissue and underlying fascia.19 The surgeon is then able to directly separate the abdominal wall from the underlying tissues, minimizing the risk of damage to the bowel and vasculature. Once the surgeon has entered the abdominal cavity, a trocar can be placed under direct vision and sutured in place. Gas can then be insufflated directly through a side port in the Hasson trocar establishing the pneumoperitoneum.
Physiologic Effects of the Pneumperitoneum
As the complexity of laparoscopic surgery and the severity of illnesses in patients undergoing these procedures increase, the need to have a comprehensive understanding of the physiologic effects of pneumoperitoneum becomes paramount. The magnitude of patient response to pneumoperitoneum depends upon multiple factors, including the degree of intraabdominal pressure generated during creation of the pneumoperitoneum, the length of surgery, patient position, patient age, perioperative volume status, and the presence of preexisting pulmonary and/or cardiovascular disease.9,20,21 Although no clinical complications have been demonstrated as being a result of transitory elevations in intraabdominal pressure, prolonged periods of high intraabdominal pressure and tension, such as those required for successful laparoscopy, have been associated with significant physiologic effects.9,22–24 These changes occur as a result of direct mechanical pressure and the stimulation of intrinsic neurocirculatory responses. When combined with surgery-specific conditions, unique patient positioning needs (i.e., steep Trendelenburg), and variations in anesthetic technique, the net effect of intraabdominal pressure on physiologic hemostasis may be unpredictable. Fortunately, long-term clinical complications are rare.
Cardiovascular Effects
Current research has shown that creation of a pneumoperitoneum is associated with significant changes in hemodynamic parameters (Box 31-2). Consistently, increases in mean arterial pressure (MAP), systemic vascular resistance (SVR), and heart rate (HR) that are sustained over the duration of insufflation have been demonstrated.9,20,25,26 Compression of the intraabdominal vessels and release of neuroendocrine hormones (i.e., vasopressin and renin) are implicated as causative factors in this hemodynamic response. The increases in MAP and SVR are observed regardless of whether the pneumoperitoneum is created under low pressure (12 mmHg) or high pressure (20 mmHg).9
Reports on the impact of abdominal insufflation on cardiac filling pressures are mixed. Several studies demonstrate increases in central pressures, whereas others show significant reductions.20,26–28 Confounding variables, such as the use of vasodilating anesthetics and perioperative fluid restrictions, can significantly alter preload. It is known that insufflation compresses abdominal vasculature, but the extent to which this impacts venous return is not fully understood. Changes in position appear to have a greater effect on central pressures than does the pneumoperitoneum itself.20,26 Steep Trendelenburg produces significant increases in central venous pressure because it increases hydrostatic pressure at the level of the external auditory meatus.20
Uniformly, studies demonstrate that creation of the pneumoperitoneum produces significant decreases in stroke volume.26,27 These changes appear to be secondary to decreases in venous return and not changes in myocardial function. Russo et al.26 demonstrated that in patients with healthy myocardium, left ventricular systolic function and ejection fraction as measured by transesophageal echocardiogram (TEE) were preserved regardless of surgical position. Changes in stroke volume were associated with early diastolic impairment as a result of prolonged deceleration time and augmented isovolumetric relaxation time. The decreases in stroke volume caused by increased intraabdominal pressures can be offset through adequate perioperative hydration, changes in patient position, and the application of compression stockings because these interventions augment venous return.27
Although it is clear that stroke volume decreases as a result of abdominal insufflation, the impact of pneumoperitoneum on cardiac output (CO) and cardiac index (CI) is variable because these parameters are influenced by a multitude of factors. In several studies, CO was maintained because reductions in stroke volume were offset by increases in heart rate and venous return, especially when patients were placed in the Trendelenburg position.26,28 Other researchers have observed significant reductions in CI after operative insufflation pressures are achieved.29,30 Healthy patients undergoing laparoscopic cholecystectomy have demonstrated 30% to 40% reductions in CO using a thermodilution technique.29 In addition, significant reductions in left ventricular end-diastolic pressures have been demonstrated, which in turn negatively impact cardiac function if not accompanied by sufficient increases in heart rate.30 Despite the variability in findings, patients who have been adequately fluid loaded and who have received therapies to augment venous return (e.g., compression stockings) experience significantly smaller reductions in CO as compared with control patients (20% versus 50%).27
Finally, the pneumoperitoneum appears to have an effect on the cardiac conduction system, even in healthy patients. A study by Ekici et al.31 showed that high-pressure insufflation significantly prolonged QT dispersion (QTd) in patients undergoing laparoscopic cholecystectomy as compared with a low-pressure control group. The QTd reflects ventricular instability, and prolongation of this parameter is associated with an increased risk of arrhythmias and cardiac effects. Although these changes would not be expected to have clinical significance in healthy patients undergoing elective laparoscopic procedure, they may produce significant hemodynamic effects in elderly patients with significant cardiac disease.
Hemodynamic Changes in the Elderly
Most research on the hemodynamic effects of abdominal insufflation has been conducted in young healthy patients. Therefore, little is known about the impact of the pneumoperitoneum in the elderly population, who often present with significant comorbidity. When compared with healthy patients, patients with significant comorbidity have been shown to exhibit exaggerated hemodynamic responses to pneumoperitoneum.32,33 The cumulative effects of carbon dioxide gas (CO2) in the pneumoperitoneum and the reverse Trendelenburg position can result in moderate decreases in CO, as well as significant increases in filling pressures and afterload in sick patients. In one study of patients undergoing laparoscopic colorectal surgery, elderly patients exhibited greater increases in central venous pressure (CVP) and decreases in MAP, as compared with younger, healthier patients.33 In addition, the magnitude of these changes at different time intervals was also greater in the elderly population. These findings may reflect baseline physiologic differences in organ function and compensatory mechanisms in the elderly population.33
Respiratory Effects
Although laparoscopic surgery is associated with a significant decrease in postoperative respiratory complications, intraoperative pulmonary function is greatly impacted by both the mechanical effects of the pneumoperitoneum and introduction of CO2 into the intraperitoneal cavity itself. Increases in intraabdominal pressure shift the end-expiratory position of the diaphragm cephalad, decrease functional residual capacity (FRC), and create areas of atelectasis, making ventilation difficult.34 In addition, CO2 insufflation causes acid-base alterations that can have deleterious effects on multiple organ systems.35
In the presence of fixed minute ventilation, CO2 pneumoperitoneum is associated with increases in the partial pressure of arterial CO2 (Paco2) and end-tidal CO2 (ETco2) with or without acidosis.35 The increases in Paco2 are primarily caused by CO2 absorption through the peritoneal serosa secondary to the increased intraabdominal pressure caused by the pneumoperitoneum. The resultant acidosis is considered to be respiratory rather than metabolic in nature. Studies show that during laparoscopy, decreases in arterial pH are accompanied by a rise in partial pressure of carbon dioxide (Pco2), but not by increases in lactate or decreases in the apparent strong ion difference (SIDa).35 These parameters return to baseline after discontinuation of CO2 insufflation. Maximum absorption of CO2 is noted with an intraabdominal pressure of 10 torr (mmHg pressure). Paco2 levels are noted to reach a plateau approximately 40 minutes after the induction of the peritoneum.35–37
A major concern during the creation of the pneumoperitoneum involves the risk of subcutaneous tracking of CO2 through misplaced trocars or rents in the peritoneum that develop during trocar placement. Compared with intraperitoneal insufflation of CO2, extraperitoneal insufflation (subcutaneous absorption) has been associated with an unusually rapid increase in Paco2 and exceptionally high, sustained levels of CO2.38–40 Cases of pneumopericardium and severe emphysema of the orbit have been documented after subcutaneous absorption of CO2.38,39 Factors that increase the incidence and degree include body mass index (BMI) less than 25, prolonged operative time, higher end-tidal carbon dioxide tensions, and operative approach.40,41 Although mild hypercapnia (45 to 50 torr) is not believed to be clinically significant, profound hypercapnia with Paco2 levels in the range of 50 to 70 torr is associated with physiologic effects such as increased cerebral blood flow, peripheral vasodilation, pulmonary vasoconstriction, and increased risk of cardiac dysrhythmia.20,42
The mechanical effects of peritoneal insufflation impair ventilation. Insufflation of the peritoneum displaces the diaphragm in a cephalad direction, decreases functional residual capacity, decreases vital capacity, and in turn induces collapse of the dependent regions of the lungs.34,43 Perfusion of these nonventilated alveoli causes the development of pulmonary shunt with impaired oxygenation and CO2 elimination.34,43 The development of atelectasis is also associated with an increase in the arterial to end-tidal Pco2 difference.34 Therefore, techniques such as transcutaneous carbon dioxide (PTco2) monitoring may serve as a better predictor of arterial carbon dioxide (Paco2) levels than end-tidal Paco2 monitoring.44 Increases in intraabdominal pressure also affect pulmonary compliance; in supine patients, pulmonary compliance has been observed to be reduced by 43%.20,32
The impact of atelectasis on gas exchange has been shown to be offset by a redistribution of perfusion away from collapsed lung units when the pneumoperitoneum is established.43,44 Although the exact mechanism is not known, it appears to be the result of activation of the hypoxic pulmonary vasoconstriction (HPV) reflex.43 Because many anesthetics inhibit the HPV reflex, increases in ventilation/perfusion () mismatching and changes in oxygenation during laparoscopic surgery may reflect the physiologic effects of the anesthetics used, rather than the impact of the pneumoperitoneum itself. This may have implications for the choice of anesthetics used during these procedures. Physiologic pulmonary changes associated with pneumoperitoneum are listed in Box 31-3.
An additive effect is observed when general anesthesia, which in and of itself reduces FRC and pulmonary compliance, is combined with pneumoperitoneum.34,43 The surgical position employed to facilitate exposure can either aggravate or attenuate pneumoperitoneum-induced pulmonary changes. The Trendelenburg position has been shown to increase the effects of pneumoperitoneum on pulmonary mechanics. During robotic laparoscopic prostatectomy in the steep Trendelenburg position, pulmonary compliance decreased approximately 50% and peak plateau pressures increased by 50%, and remained stable over the duration of the CO2 insufflation.20 In contrast, the reverse Trendelenburg position partially counteracts the effects of pneumoperitoneum on the diaphragm and improves diaphragmatic function.45
Another impact of pneumoperitoneum is the potential for the development of endobronchial intubation as a result of a shortening of the distance from the tip of the endotracheal tube to the carina.46–48 Since the carina is attached to the lungs, cephalad displacement of the diaphragm compresses the lungs and shifts the position of the carina upward. Studies indicate that displacement of the tube occurs within 10 minutes of creation of the pneumoperitoneum; therefore the authors recommend reconfirmation of tracheal tube position after establishment of the pneumoperitoneum.47,48
Controlled mechanical ventilation is necessary to maintain normocarbia in anesthetized patients undergoing laparoscopic surgery with CO2 pneumoperitoneum. Studies of patients in the Trendelenburg position during pneumoperitoneum reveal that a 20% to 30% increase in minute ventilation is necessary to maintain pre-pneumoperitoneum levels and prevent respiratory acidosis.35,49 Increasing the minute ventilation by preferentially increasing tidal volume rather than increasing respiratory rate was the preferred method. In addition, the use of the pressure control (PC) modes appears to be more effective in maintaining arterial pH when compared with the volume control (VC) modes of ventilation.50 Patients ventilated using PC were also easier to ventilate, generating significantly lower maximum peak airway pressures and increased mean airway pressures.
Patients with marginal cardiopulmonary function undergoing laparoscopic surgical procedures are at increased risk of decompensation when faced with the stress introduced by increases in intraabdominal pressure and CO2 insufflation. Patients particularly vulnerable to the effects of exposure to prolonged CO2 insufflation are those with increased metabolic rates (as in sepsis), large ventilatory dead space, and decreased cardiac output.21 Patients with chronic obstructive pulmonary disease (COPD) are at increased risk of developing postoperative complications after laparoscopic procedures.51,52 One study demonstrated the odds ratio for postoperative complication to be 1:63 in patients with significant COPD.51 Studies also indicate that although healthy patients experience minor changes in Paco2 and ETco2 during CO2 insufflation, patients with COPD show significantly higher levels of CO2 retention and subsequent respiratory acidosis.53 Although ETco2 is standard of care for all patients, careful intraoperative monitoring of CO2 levels during laparoscopic surgery in these patients is essential. Because of the significant arterial to end-tidal Pco2 gradient that develops during sustained pneumoperitoneum, and which is compounded in patients with chronic CO2 retention, ETco2 may underestimate arterial CO2. Therefore, direct measurement of Paco2 via arterial blood gases or PTco2 may be warrented.34,42 Recent studies show that PTco2 is an accurate, noninvasive predictor of arterial carbon dioxide levels and can be used as a proxy.42
Mild pulmonary dysfunction after laparoscopic procedures has been shown to persist into the immediate postoperative period in patients recovering from laparoscopic surgery. A slight restrictive breathing pattern in the postoperative period has been observed secondary to the residual effects of anesthesia, pain, and diaphragmatic dysfunction induced by stretching or reflex inhibition. In addition, after laparoscopic surgery, patients may still be subjected to an elevated CO2 load secondary to systemic absorption and subcutaneous tracking of gas during the procedure. Moreover, if surgery is prolonged and exposure to pneumoperitoneum is sustained for the majority of the procedure, CO2 may be stored in skeletal muscle and bone. It may take hours for this excess CO2 to be excreted from the patient.54
Renal Effects
The effects of pneumoperitoneum on renal physiology primarily manifest as transient increases in creatinine clearance and decreases in urinary output.23,55,56 Oliguria has been reported but most commonly occurs only during periods of sustained high intraabdominal pressures.23,55,56 It is proposed that the high intraabdominal pressures created during the pneumoperitoneum cause transient renal injury by reducing renal blood flow and subsequently causing hypoperfusion of the renal cortex. High intraabdominal pressures (15 mmHg or greater) create renal oxidative stress and the generation of tissue oxidases that promote tubular injury.23 In addition, the neuroendocrine response to pneumoperitoneum, the release of antidiuretic hormone (ADH), and the presence of respiratory acidosis induces a sympathetic response and renal vasoconstriction, which further diminishes renal blood flow.23,57,58
Hepatic and Splanchnic Effects
There is conflicting evidence regarding the effects of increased intraabdominal pressure on hepatic and splanchnic blood flow. Several animal studies have demonstrated that abdominal insufflation can cause marked decreases in splanchnic and liver perfusion, as well as intestinal ischemia secondary to the production of oxygen free radicals and bacterial translocation.59,60 This is further supported by the fact that approximately 50% of patients undergoing laparoscopic cholecystectomy demonstrate elevated liver enzymes.61,62 Other studies indicate that low-pressure insufflation of the abdomen either did not disrupt or improved hepatic and splanchnic perfusion, possibly secondary to the local vasodilatory effect of CO2 on splanchnic vasculature.63,64
Immunologic Effects
CO2 pneumoperitoneum also may have a negative effect on the local immune response by altering the concentrations of certain cytokine levels with the peritoneum. Several studies have demonstrated that CO2 pneumoperitoneum influences the growth of cultured human cancer cells, and that this effect is pressure dependent.65
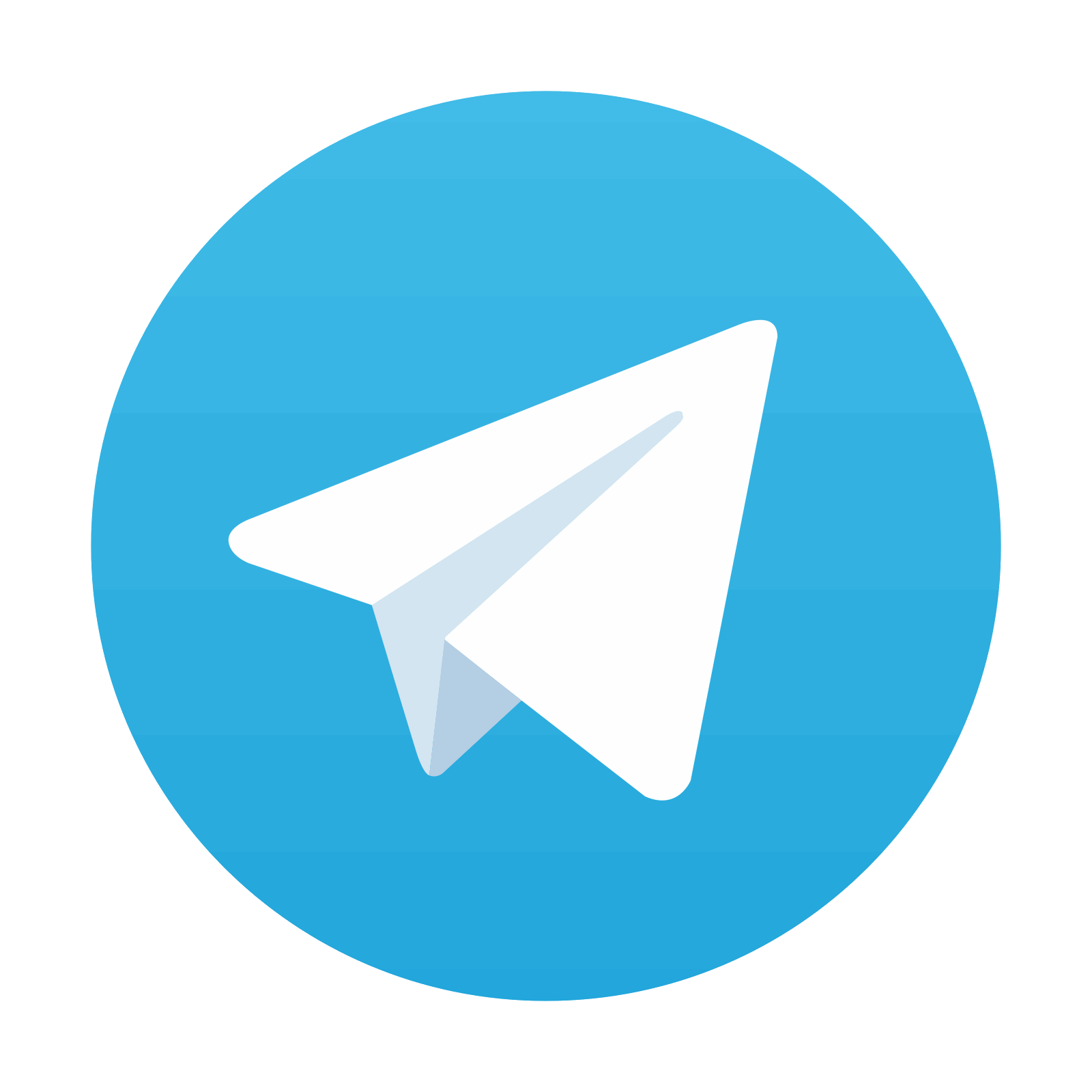
Stay updated, free articles. Join our Telegram channel

Full access? Get Clinical Tree
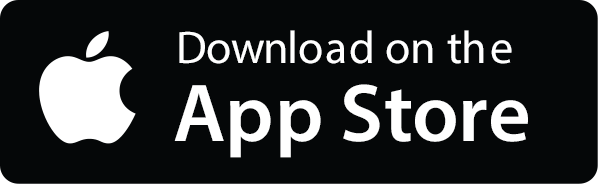
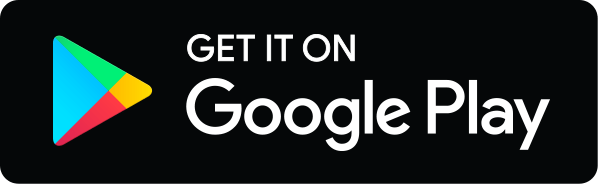