Inpatient
Outpatient
Emergency room
Total
Percentage (%)
Prostate cancer
$264,414,460
$660,791,840
$2,218,220
$927,424,520
0.23
Bladder cancer lower tract transitional cell carcinoma
$446,062,440
$239,802,080
$1,139,600
$687,004,120
0.17
Bladder cancer upper tract transitional cell carcinoma
$24,814,400
$7,625,900
a
$32,440,300
0.008
Kidney cancer
$104,869,840
$24,348,960
a
$129,218,800
0.03
Total Medicare
394,500,000,000
Perianesthetic Management
Preanesthetic Assessment
The preanesthetic assessment consists of a history and physical exam of the patient, a review of the medical record, laboratory tests, and other tests. An anesthetic plan is developed based not only on the patient’s physical status determined by the assessment but on how the patient will tolerate pneumoperitoneum and body position during the surgery. The establishment of a high intra-abdominal pressure (IAP) due to pneumoperitoneum and body position is a major factor for possible cardiopulmonary derangements intraoperatively. Factors such as obesity and the degree of Trendelenburg can intensify the adverse effects of high IAP in the patient and should be considered into the overall anesthetic plan. A search for issues such as a difficult airway, cardiopulmonary status, allergies, medications, and comorbid conditions is essential. A well-thought-out plan based on patient factors, operative factors, and patient position can reduce or eliminate complications.
Choice of Anesthesia
General Anesthesia
Once the patient is determined to be an acceptable candidate to proceed to the operating room the most common anesthetic plan is general anesthesia. There are several recommendations for specifically managing various types of robotic-assisted urologic surgery [14, 15]. General endotracheal anesthesia is chosen to counter the adverse conditions created by the pneumoperitoneum, patient positioning, and surgical time. Pneumoperitoneum and patient positioning impede normal respiratory mechanics. Placement of an endotracheal tube allows the ventilator to supply the work necessary to breathe. An endotracheal tube also protects the airway. Gastric secretions are commonly seen in the oropharynx or on the face of patients at the end of surgery. The increased intra-abdominal pressure and the head-down position increase the risk for a backward movement of gastric effluent. These conditions as well as long surgical times favor the use of a general anesthetic. Surgeons new to robotic surgery have not acquired the efficiency compared to high-volume surgeons, and the operative time is often prolonged. There is a learning curve with decreasing length of surgical time as experience increases. General anesthesia also allows the use of muscle relaxants. Muscle paralysis reduces the increase in intra-abdominal pressure needed for the same degree of abdominal distention [16]. A secure large-bore IV intravenous catheter (at least an 18 gauge) is necessary because of the chance for significant blood loss. The placement of an arterial line may be indicated if the patient’s medical condition warrants closer blood pressure monitoring. Placement of these devices should be accomplished before robotic surgery begins in that access to the patient is severely limited once surgery is underway.
Neuraxial Anesthesia
Although there are reports of the use of spinal anesthesia alone for laparoscopic procedures, [17] it may not be prudent for robotic surgery. The envelopment of the patient by the robot is claustrophobic; the need for spontaneous ventilation and long operative times often lead to patient movement, perhaps causing patient injury and the need to change the anesthetic technique under less than ideal circumstances. The use of epidural anesthesia for short outpatient diagnostic laparoscopic gynecologic procedures has been described [18]. Epidural anesthesia has the same drawbacks as spinal anesthesia for robotic surgery. Combining general anesthesia with epidural analgesia in surgical cases has a wide range of beneficial effects. Pain scores improve, less narcotics are required, and the incidences of ileus, nausea and vomiting, sedation, and cardiac and pulmonary morbidity are decreased [19–22]. The majority of these benefits were analyzed in traditional surgical cases but are assumed to translate to robotic surgical cases. A greater benefit is noted when using thoracic epidural analgesia versus lumbar epidural analgesia. In order to provide the benefits of neuraxial anesthesia and meet the requirements of general anesthesia for robotic surgery, combining a single-shot spinal anesthetic with general anesthesia has been described [15]. The addition of epidural anesthesia for pain control in prostate cancer patients undergoing open radical prostatectomy was associated with a reduced risk of clinical cancer progression [23]. Additional research is needed before this practice can become a recommendation.
Local Anesthesia
In addition to the use of local infiltration of local anesthetic at the port sites, a regional technique is available for use in urologic robotic surgery; the transversus abdominis plane block. The transversus abdominis plane (TAP) block is well suited for robotic-assisted laparoscopic radical prostatectomy. It is a simple block that can be performed quickly and in the supine position. The TAP block can be performed either by landmarks or with ultrasound guidance. It was initially a landmark-guided technique [24] eventually followed by an ultrasound-guided technique [25]. Recently a variation of the original TAP block, a subcostal TAP block, has been described [26]. Injection of local anesthetics into the intermuscular plane can provide anesthesia to the skin, muscles, and parietal peritoneum of the anterior abdominal wall. These blocks have been shown to provide good postoperative analgesia for a variety of surgical procedures [27, 28]. A bilateral TAP block can cover the lower abdominal surgical field involved in robotic prostatectomy. Care must be taken to not exceed recommended local anesthetic doses and cause toxicity. There have been several reported complications with the landmark technique, including intraperitoneal injection, femoral nerve palsy, bowel hematoma, and intrahepatic injection [29]. Using ultrasound guidance allows more exact placement of the local anesthetic to the transversus abdominis plane and helps avoid inadvertent injections into the wrong tissues.
Monitoring
The standard American Society of Anesthesiologists (ASA) monitors must be used in minimally invasive surgical cases. These include noninvasive blood pressure, electrocardiogram, pulse oximetry, capnography, and thermometer. Regional and national requirements for preoperative antibiotics, venous thromboprophylaxis, patient identification, and verification of correct surgery and side are required before the start of surgery. The norm is to accomplish these tasks in the peri-induction period. Placement of invasive monitors such as an arterial line and/or a central line (see above) depends upon patient and surgical factors. Though not mandatory, the arterial pressure monitor is useful to monitor the unpredictable hemodynamic responses (severe bradycardia, hypertension, hypotension, tachycardia) during the procedure and allows real-time pressure readings in patients with comorbidities. This invasive monitor is relatively low risk and allows the anesthesiologist to more quickly respond to hemodynamic changes. Access to the upper extremity is limited once the robot is docked so the placement of an arterial line should be performed prior to docking.
Nasogastric tube decompression of the stomach and Foley catheter drainage of the bladder is basic procedures for most urologic laparoscopic surgeries. At our institution, in collaboration with our surgeons, we no longer routinely place nasogastric tubes prior to robotic prostatectomy because it did not improve the surgical conditions. Deviation from the practice of stomach decompression can be done if both the surgeon and anesthesiologist agree upon it.
Hypothermia is common beginning with the disruption of thermal regulation due to anesthesia. Using a sterile preparation solution at room temperature in a cool room adds to the heat loss. Continuous heat loss can occur due to the insufflation of large amounts of cold carbon dioxide gas into the peritoneum throughout the case. Warm fluids can be infused and the room temperature can be increased to mitigate hypothermia. Forced-air warmers can be used but the difficulty comes in finding a body surface to cover with the warming blanket. At the very least, an upper body warmer can cover the chest and head. However, there have been recent studies proposing that forced-air warming disrupts the intended operating room air flow which clears the contaminants from the site of surgery, leading to an increased chance of infection [30, 31]. New technology such as the Hot Dog® warmer uses a conductive fabric to warm patients.
Anesthesia Complications
Avoidance and/or minimization of anesthesia complications starts with knowing the patient and understanding the physiologic burdens placed upon the cardiopulmonary system due to pneumoperitoneum and patient positioning. Anesthetic complications are addressed through that prism: anesthetic strategies to minimize hemodynamic changes due to pneumoperitoneum and patient position. An animal study examined three different therapeutic approaches: one was to increase the intrathoracic blood volume with colloids, the second was to reduce sympathetic activity with esmolol, and the third was to decrease mean arterial pressure using sodium nitroprusside [32]. The authors found that increasing the intrathoracic blood volume improved hemodynamic function in all body positions with pneumoperitoneum. Esmolol reduced cardiac output and myocardial contractility. Sodium nitroprusside did not improve hemodynamic function. Other than stating that fluid management is the most important element for minimizing pneumoperitoneum side effects, no recommendations can be made as to the type of fluid to use, how much to give, what are the fluid end points, and how to monitor the patient.
Surgical Complications
Complications are part of robotic surgery just as they are following open procedures. The majority of reported complications in MIS are surgical in nature. They are more likely to occur in the beginning phases of a robotic program. In a multi-institutional study of 185 patients, complications occurred in 16% of patients. Of these complications, 71% occurred during the initial 20 cases at each institution [33]. Thermal injury, instrument trauma, inadvertent dissection, trocar misplacement, and careless robotic instrument changes can all cause perforation and/or bleeding. Disruption of organs or tissue can be repaired. Bleeding can be corrected in a timely and non-emergent fashion with endoscopic clips or staplers, direct pressure, cautery, fibrin sealants, and increase of the insufflation pressure. In order to minimize the risk of a venous air embolus, the insufflation pressure should be set at a level below the venous pressure. Large rapid bleeding must be quickly corrected. In this scenario synchronous, coordinated actions are required by the entire operating room team if the surgeon elects to convert to an open procedure. Undocking the robot and repositioning the patient occur as the anesthesiologist is preparing for a possible rapid and robust resuscitation. Practicing a simulated emergency with undocking of the robot is a valuable experience to prepare for the real situation.
Fluids
Given the above animal study and clinical experience, it is very beneficial to have a normovolemic patient. How to achieve that is difficult. Unfortunately the best advice is to “give the patient as much as they need and not a drop more.” Until goal-directed therapy is common across all cases, the anesthesiologist is given wide latitude in what type of fluid to give, what rate to give it, and to what final amount. The technology that is currently available to guide the anesthesiologist in the optimal use of intravenous fluids using cardiac output includes the traditional pulmonary artery catheter, thoracic bioimpedance, esophageal and transgastric Doppler techniques, endotracheal cardiac output monitor (ECOM), and pulse contour waveform analysis from the axillary or femoral artery. Measuring cardiac output strictly to guide fluid therapy in low- or moderate-risk surgical procedures is labor intensive and not routinely performed with the exception of ECOM. Less-invasive techniques using the arterial wave form and the pulse oximetry wave form are available that assess for fluid responsiveness. Fluid responsiveness is defined as an increase in stroke volume (or cardiac output) in response to a fluid challenge of greater than 15%. The limitations for the use of these dynamic parameters for fluid responsiveness is that patients are receiving general anesthesia, mechanically ventilated, tidal volume > 8mL/Kg, no arrhythmias, and possibly no right ventricle dysfunction. Managing fluid administration via goal-directed therapy is beyond the scope of this chapter. Either end of the volume spectrum is detrimental to the patient. A hypovolemic patient can be an unstable patient hemodynamically. A hypervolemic patient may suffer the increased risk of bowel dysfunction, anastomotic leak, wound infection, and cardiovascular complications [34, 35]. Adding to the complexity a normovolemic patient for robotic surgery may be a hypovolemic patient for an open procedure. Given the decreased blood loss, decreased insensible loss, and decreased urine output due to insufflation pressures, less fluid is needed than in the corresponding open procedure. Blood replacement is less common in the robotic procedure versus an open procedure but still can occur and be occult [36].
Equipment
The Robot and Equipment
Anesthesiologists have a long history of equipment and machine use for patient care. Ventilators, monitors, and ultrasound machines are commonplace. Having a working knowledge of what the equipment does, how to operate it, and more importantly how to troubleshoot it allow us to overcome intraoperative obstacles and machine failures.
Currently the dominant system for robotic surgery is the da Vinci Surgical System, also known as the Endoscopic Instrument Control System. There are no major competitors to date. A robot device is “a powered, computer controlled manipulator with artificial sensing that can be reprogrammed to move and position tools to carry out a wide range of tasks” [37]. It can function independently. Robotic systems used in surgery are computer-assisted devices, more accurately defined as telemanipulators. The surgeon is able to manipulate surgical instruments as if their hands were in the surgical field. The robot performs tasks under the surgeon’s control and enhances his/her manual dexterity. The current configuration of the da Vinci system has three components: the surgeon console (see Fig. 6.1), the patient cart (see Fig. 6.2), and the vision cart (see Fig. 6.3). The surgeon sits at the surgeon console outside of the sterile operative field and controls instruments and a three-dimensional endoscope with hands and feet using two master controllers for the hands and variously configured foot pedals. The system is designed to align the eyes, hands, and instruments in a way that simulates open surgery. The surgical instrument tips operate just as if they were in the surgeons’ hands even though they are being manipulated via the master controllers. Further refinement is provided by motion scaling and tremor reduction. A one unit measure of motion of the surgeons’ hands can be translated to a one-half unit or two unit measure of motion at the surgical site.
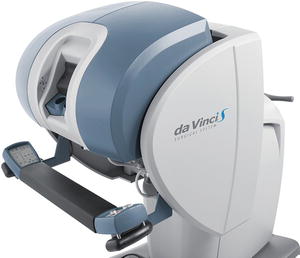
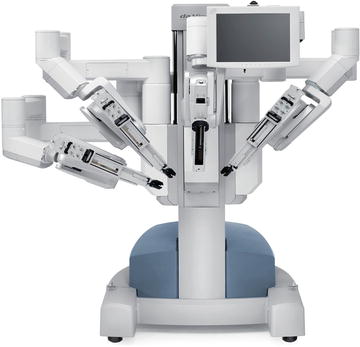
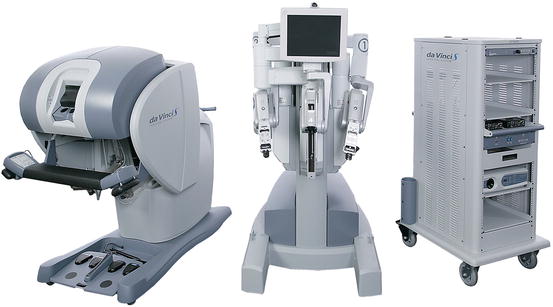
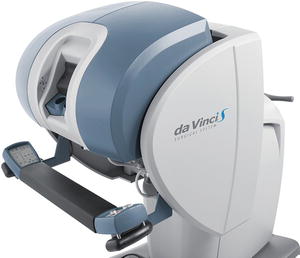
Fig. 6.1
Surgeon console
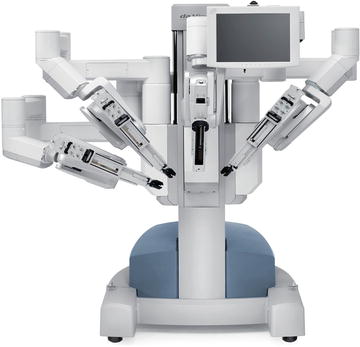
Fig. 6.2
Patient cart
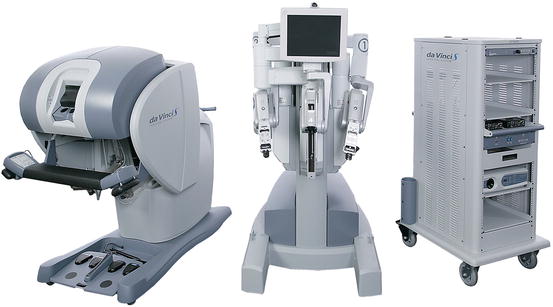
Fig. 6.3
Vision cart (far right)
The patient cart is what most patients refer to as “the robot”. It is the operative component of the system and works in the sterile field. The surgeon has three working arms (see Fig. 6.4) available and a three-dimensional endoscope or camera. While the surgeon sits at the console, a bedside assistant works in the sterile field along with the robot. To aid in patient safety, the actions of the bedside assistant on the robot take precedence over the commands of the surgeon at the console.
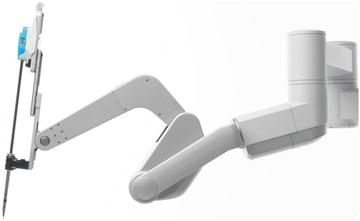
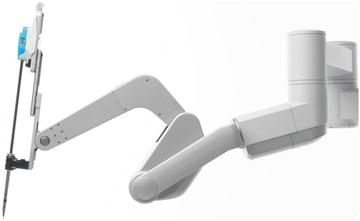
Fig. 6.4
Robotic arm (one of three)
The third part is the vision cart, which is outside of the sterile field and houses the imaging processing equipment. It also can have a monitor allowing the bedside assistant to view the procedure in a two-dimensional mode. There is space for ancillary equipment.
Several other important concepts about the system are the endoscope and the instruments. The endoscope or camera is able to create a three-dimensional view for the surgeon via a fiber optic cable; light traverses the length of the endoscope and illuminates the operative field. The surgical site is then viewed and images captured by a right and left channel on the endoscope. Images from these two separate channels are processed and can be viewed in real time in three dimensions by the surgeon. The number and type of instruments that actually touch and manipulate patient tissue via the robot is increasing constantly. The big step-up evolutionarily was the development of instruments with “wrists.” These instruments provide natural dexterity and a greater range of motion than the human hand. The entire system is designed to allow cutting, dissecting, retracting, and suturing with minimal damage to normal surrounding tissue. What the robot cannot simulate is tactile sensation or haptics, and research is continuing to allow the surgeon to “feel the tissue.”
Robot Reliability
The complexity of the Endoscope Instrument Control System rivals any other operating room equipment. The size and weight alone necessitate operating room logistical considerations. The surgeons’ console weighs ~363 kg (~800 lbs.), the patient cart ~544 kg (~1,200 lbs), and the vision cart ~91 kg (200 lbs). Given the complexity of this system, how reliable is it? The literature does try and answer these questions though studies use different methods and end points. Clearly intraoperative device failure impacts anesthetic care. At our institution, we examined 1,033 robotic-assisted laparoscopic cases across a variety of surgical specialties examining intraoperative robot system malfunction [38]. The case distribution was 55 cases for general surgery, 48 cases for gynecology-oncology, 43 cases for thoracic surgery, and 887 cases for urologic surgery. In no instances was the patient harmed or the surgery aborted. In 16 cases, a serious robotic malfunction occurred. In 10 cases, the malfunctioning robot was exchanged for another robot intraoperatively. The average time to remove and replace the malfunctioning robot was 24.5 min. The robot was shut down and restarted in two cases correcting the malfunction. The final four cases were completed using three functional arms or converting to laparoscopy. The overall serious robotic system malfunction rate was 1.54%. Considering the complexity of the robot, the overall incidence of failure may be considered as low.
Lavery et al. [39] examined robotic equipment malfunction across multiple institutions during robotic-assisted prostatectomy using a questionnaire to high-volume experienced surgeons. The total case volume was 8,240. Critical failure occurred in 34 cases (0.4%). Twenty-four of these critical malfunctions were prior to the procedures and the cases were cancelled. Of the remaining 10 cases, two were completed laparoscopically and eight were converted to open procedures. The authors concluded that in high-volume institutions performing robotic prostatectomies, critical system malfunction is rare and in experienced hands the da Vinci robotic system is reliable. Borden et al. [40] examined their experience with the first 350 robotic-assisted prostatectomies. Nine (2.6%) of the cases could not be completed robotically because of device malfunction. Six of these situations were detected prior to surgery and rescheduled. In three cases, there were intraoperative critical malfunctions; one case was completed laparoscopically and two were converted to open procedures. They also concluded that device malfunction was uncommon.
A review of the FDA’s Manufacturer and User Facility Device Experience Database (MAUDE) reported a device failure rate of 0.038% [41]. This study examined the years from 2000 to 2007 and included the ZEUS robotic system (2001–2003) which is no longer supported and the da Vinci robotic system (2000–2007). A total of 189 adverse events were reported of which 168 malfunctions were with the da Vinci system. This database is under the auspices of the United States Food and Drug Administration and is voluntarily reported.
Regardless of the type of study, the method of data extraction and analysis, single institution or multi-institutional, each study commented that the da Vinci robotic system was reliable. Unrecoverable robotic malfunctions are uncommon.
Nayyar et al. [42] analyzed 340 consecutive robotic-assisted urologic procedures from a single institution using the same robot. An overall device failure rate of 10.9% (37/340) was found. Twenty-eight of the 37 problems (76%) were correctable during the surgery, and 23 of these problems were “instrument related” and 15 occurred in their first 150 cases. It may have been associated with the team’s learning curve. Kaushik et al. [43] surveyed urologists performing robotic-assisted prostatectomies as to the stage of the operation the malfunction occurred, the management of the malfunction, and the most common types of robotic malfunctions. The vast majority of robotic malfunctions occurred or were discovered before the start of surgery. Either the case could be rescheduled or the patient could elect to have non-robotic-assisted surgery. Of the malfunctions that occurred intraoperatively, malfunction of the robotic arm was the most common and could be overcome by changing arms or using the remaining functioning arms. This study supports the notion that the da Vinci robot should be fully checked and operational before the patient is subjected to a general anesthetic as the majority of malfunctions can be captured preoperatively.
Positioning
Positioning is an important component in facilitating minimally invasive procedures by utilizing gravity on the organs to provide optimal surgical exposure. With optimal conditions, one can assume that the operative times are decreased and patient morbidity is minimized. In addition to proper positioning of the patient, the surgical table may also be positioned in a unique formation so that the robot and surgical assistants can be accommodated. Thus, the combination of the position of the patient and the location of the table in relation to the anesthesia machine impact the anesthetic plan. Position for both laparoscopic and robotic procedures is similar. The main difference is that in robotic surgery, the robot adds a bulky physical impediment with four active arms, envelops the patient, and limits patient access when the robot is docked. Therefore, the robot should be docked only after the patient has been optimally positioned for surgery.
Trendelenburg Position
The most common position for laparoscopic and robotic urological procedure is the low dorsal lithotomy with steep (35°) Trendelenburg position (see Fig. 6.5). This position is used for laparoscopic and robotic prostatectomy, cystectomy, retroperitoneal lymph node dissection, and ureteral procedures. The second most common position is the lateral jackknife (hyperextended) decubitus position for procedures involving the kidney and adrenal gland. In our institution after the patient is positioned on the surgical table, the table is arranged in two ways in relation to the anesthesia machine. The main reason for moving the table is to provide the surgeon and surgeon’s assistant more physical space so they feel less confined.
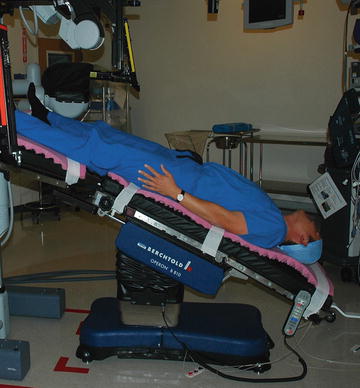
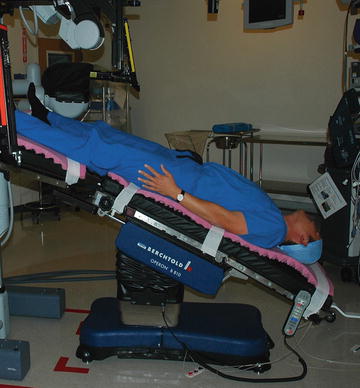
Fig. 6.5
Steep Trendelenburg
In the low dorsal lithotomy and Trendelenburg position, the table is turned 30° in the counterclockwise direction which allows access for the docking of the robot. This maneuver also allows the surgical assistant to be located on the patient’s right side and decreases the likelihood of violating the sterile field.
Lateral Jackknife
For a patient in the left lateral jackknife decubitus position, the table is turned 90° counterclockwise or for a right lateral jackknife decubitus position, 90° clockwise. The primary reason for moving the table to this location is to facilitate docking of the robot, which approaches the patient from posterior at our institution. For conventional laparoscopic procedures in the decubitus position, the table is not turned.
Limited Access to Patient
The steep Trendelenburg position and the size of the robot require attention to three areas that pertain to the anesthesiologist: (1) vascular access, (2) airway access, and (3) physiologic changes. The procedure and the patient’s coexisting condition determine the need to secure certain vascular access devices which range from a second intravenous line, arterial line placement, or rarely a central venous catheter. In order to minimize disruption, enhance efficiency, and maintain sterility, it is preferable to place all of these devices immediately after the induction of anesthesia and the securing of the airway.
Traditionally, a single-lumen endotracheal tube is used for airway management. Once the patient and table are in its final location, the typical access to the airway becomes obstructed by the surgical drapes and distance. In addition, a tracheal intubation may become endobronchial both when the patient is in the lateral position as well as when the patient is placed in the Trendelenburg position. The Trendelenburg position causes a cephalad displacement of the trachea in such a manner that an endotracheal tube immobilized at the lips may migrate into a main-stem bronchus. This slippage may be further exacerbated when the diaphragm is forced cephalad by peritoneal insufflation. It is imperative that constant vigilance is employed to ensure that the endotracheal tube is secured and remains in the desired position.
Physiologic Changes Related to Position
Physiologically, the dorsal lithotomy with Trendelenburg produces cardiovascular, respiratory, and neurologic alterations. Without anesthesia, the body has adaptive mechanisms to maintain fairly normal parameters. With the induction of anesthesia, these reflex mechanisms can be inhibited. In the dorsal lithotomy position, the abdominal organs cause the diaphragm to be displaced cephalad decreasing tidal volume, pulmonary compliance, and functional residual capacity (FRC), while increasing airway ventilatory pressures and increasing the risk of atelectasis. These changes are exacerbated once the pneumoperitoneum is initiated. In the Trendelenburg position, there is a transient increase in cardiac output related to the increase in venous return, the heart rate is slowed, venous pressure is increased, and superior vena cava volume is doubled [44]. The cerebral blood flow and intracranial pressure is increased [45, 46] upon the initiation of the pneumoperitoneum. The lateral position affects the pulmonary mechanics more than the cardiovascular system however; the pulmonary blood flow is increased to the dependent lung thereby causing a ventilation perfusion mismatch. The lung volume is decreased in the anesthetized patient in the lateral decubitus position at the same airway pressure compared to the awake state.
Securing the Patient
Securing the anesthetized patient for minimally invasive procedures is of utmost importance. The main difference in comparison to the open procedure is the requirement for a patient to be placed in the steep Trendelenburg position. At our institution a non-sliding foam egg crate mattress is taped to the table mattress with the convoluted side down. This foam provides a large surface area for skin adherence on a patient’s back without the need for any other method of securing the patient’s torso. A sheet is not placed under the patient because it increases the likelihood of the patient sliding by minimizing the surface area contact. Shoulder braces and/or cross taping the patient to the bed may also be used but may cause brachial plexus injuries and further decrease in lung compliance. Both arms are adducted and a plastic arm sled padded with foam is used to protect the upper extremities. All pressure points are checked and padded (i.e., elbow, wrist, hands, and fingers). The intravenous line, arterial line, identification/allergy bracelets, stopcocks, noninvasive blood pressure cuff, and pulse oximetry must be carefully positioned for full functionality and in a manner that does not put any pressure and/or have a tourniquet effect. The neck and head should be aligned in the neutral position. The anesthesiologist must remember that once the patient is prepped and draped, access will be limited.
In the decubitus position, the head and neck should remain in the neutral position. During the procedure, the table is tilted toward the nondependent side, so the use of a kidney rest prevents the head/neck from hyperextending and thus accidental extubation. The taping of the head to the table as a way to prevent head/neck movement can cause pressure necrosis. An axillary roll should correctly be placed not in the axilla but more caudad to allow the chest wall to bear the weight of the upper body.
Complications of Positioning
The unique positioning requirements of these urological procedures predispose the patient to injuries such as: (1) eye injuries [47], (2) peripheral nerve injury, (3) facial/laryngeal/conjunctiva edema, and (4) increase intraocular pressure [48]. There have been reports that as the duration of the procedure is increased, the potential for injury increases perhaps due to an operator learning curve. There is an increased chance of injury in the surgeon’s first set of patients as compared to subsequent patients [104].
Ocular Injuries
Ocular injuries are caused by either physical contact with the eye or because of the edema caused of the Trendelenburg position. Corneal abrasions in minimally invasive surgery are the most common type of ocular injury with an incidence of 3% [49]. It is caused by direct physical trauma of an instrument, surgeon’s hand, facemask, drapes, etc. The patient presents with postoperative eye pain and a complaint of a foreign body feeling in the eye. The complication is treated with an eye patch and antibiotic ointment. Lavery et al. has recommended the use of occlusive dressing applied to the eyes and eye shield that has resulted in the elimination of corneal abrasion [47]. The occlusive dressing helps to minimize the frequent observation of gastric juice which has regurgitated on to the face in the steep Trendelenburg position. The eye shield is an additional barrier that helps to ensure that inadvertent physical contact to the eyes is not made by instruments, the robot arm, the drapes, and/or a surgeon’s hand.
According to the study by Anad [44], there is a 13 mmHg increase in intraocular pressure in the steep Trendelenburg position which is comparable to patients with glaucoma that have temporarily discontinued their medication. Yet there have been no cases of an increase incidence of ischemic optic neuropathy in patients with glaucoma in patients undergoing surgery in the Trendelenburg position.
Peripheral Nerve Injuries
Robotically assisted surgeries are often lengthier procedures, especially for inexperienced surgeons, thus adequate pressure point padding is essential to avoid tissue and nerve impingement. Careful attention should also be given to the robotic arms to prevent them from contacting the patient. Pressure or crush injuries may inadvertently occur if constant vigilance is not exercised. Peripheral nerve injury can occur in any surgical procedure in the anesthetized patient. According to the ASA Closed Claims analysis, nerve injuries comprise 15–16% of all anesthesia-related malpractice claims [50]. Focusing on perioperative peripheral nerve injuries over a 10-year period in 380,680 consecutive patients undergoing surgery, the incidence was 0.03% [51]. Warner et al. determined the incidence of ulnar neuropathy of 0.5% [52] and lower extremity neuropathies in the lithotomy position to be 1.5% [53]. Furthermore, this study showed that even with careful positioning, ulnar nerve injury could still occur, especially in thin males. Multiple lower extremity nerves are at risk with similar frequencies of injury. Peroneal nerve injury may be due to direct compression of the nerve at the head of the fibula by the stirrups. The sciatic nerve can be stretched by the hyper flexion at the hip and external rotation elongates the nerve, which is fixed at the sciatic notch and the fibula. The other nerves of the lower extremity that can be injured are the obturator and the femoral nerve. As mentioned, brachial plexus injuries can occur with the use of shoulder braces. Because in the dorsal lithotomy position both arms are tucked to the sides, the injury caused by abduction of the upper extremity is minimized. Unnoticed infiltration of the intravenous catheter in the antecubital fossa can lead to median nerve injury. In the lateral decubitus position, the pulse of the dependent arm should be monitored to ensure adequate blood flow. If the axillary roll is misplaced in the axilla, compression of the axillary artery can lead to paresthesia and neurovascular compromise.
Facial Edema/Laryngeal Edema
Clinical swelling of the face, eyelids, conjunctivae, and tongue along with a plethoric color of venous stasis in the head and neck is a common observation. Facial edema is common and laryngeal edema can cause a delay in extubation in up to 5% patients [54, 55]. If significant upper airway edema is suspected, it would be prudent to delay extubation until such time as the edema has subsided to a safe degree. Usually the edema resolves within an hour or two when the head is returned to above the level of the heart. Resolution is usually easily accomplished in the post-anesthesia recovery unit with the patient placed in a semi-upright position. The use of a diuretic has not been necessary unless the patient demonstrated signs of congestive heart failure or intravascular overload.
Though the position is important to provide optimal surgical conditions for the surgeon, the positioning of the patient and the table can have a great impact on the anesthetic plan and also can increase patient morbidity. Positioning is unique to minimally invasive surgery and awareness of the potential consequences of malpositioning enhances patient safety. Though rare, complications from positioning can be devastating in a procedure that is touted as minimally invasive.
Pneumoperitoneum
Trocars
Placement
Establishment of pneumoperitoneum is the first step in the performance of robotic surgery. There are four techniques: percutaneous blind placement of a Veress® needle, open-access technique (Hasson®), optical trocar insertion, and direct trocar insertion with elevation of the abdominal wall. The blind Veress® needle technique and the open Hasson® technique are common methods. The technique and complication rates of the blind method and the open method differ. Using the blind technique, the surgeon percutaneously enters the abdominal cavity with an insufflation needle in a closed technique using a Veress® needle. Once the abdomen is insufflated the camera port is next placed blindly. Finally the instrument trocars are placed under direct vision. The second technique is an open method. A surgical skin incision is made and all layers of the abdominal wall are incised down to the peritoneal cavity. The first trocar is placed under direct vision and haptic perception followed by insufflation [56]. The true incidence of injury with each technique is unknown. One study found a visceral and vascular injury rate of 0.483% and 0.075% (closed) and a 0.048% and 0.0% (open) for establishing pneumoperitoneum and placing the first trocar [57]. The closed method using the Veress® needle is the more common surgical approach but choice depends on surgical preference.
Vascular Injury
Complications associated with trocar placement are rare. When they occur they involve the viscera or great vessels and morbidity is high. More specifically complications comprise bleeding from the abdominal wall, injury to the great vessels of the pelvis, and damage to intraperitoneal viscera including the bowel and urinary tract. Injury can occur on insertion of the port, during the case, or during removal of tissue from the peritoneal cavity.
No method of placement of the trocars is completely risk-free. In obese individuals, adipose tissue can obscure clinical markers of successful and unsuccessful needle placement. At the other extreme, in thin individuals, the great vessels can be as little as 2 cm distance from the abdominal wall, placing them at risk for injury [58]. Hemorrhage may occur if the tip or edge of a trocar or needle injures a vessel. Many vessels are at risk: the aorta, inferior vena cava, and common, internal, and external iliac arteries and veins. Bleeding ranges from rapid to slow and obvious to occult. The incidence of major vascular injuries ranges from 0.04% to 0.5% [59]. The wide range reflects the heterogenicity of surgical cases and surgical volume in each study.
Gastrointestinal Injury
Gastrointestinal injuries occur but are more likely in the face of previous abdominal surgery or peritonitis where adhesions do not allow for insufflation to create an organ-free space inferior to the abdominal wall. The incidence of visceral injury has been estimated at 0.06–0.08% [60]. Many gastrointestinal injuries are not immediately recognized at the time of surgery. Gastrointestinal injuries are usually subtle as partial tears, small perforations, thermal injuries, or hernias, which can take time to become clinically apparent. They present later in the postoperative course with pain, peritonitis, abscess, enterocutaneous fistula, and/or sepsis.
Insufflation
Venous Air Embolism
Venous air embolism (VAE) is a feared complication. Its clinical manifestation can run the spectrum from subclinical to life threatening. Clinically apparent gas embolism is a rare complication (0.0014–0.6%) of laparoscopies, but it is associated with a high mortality rate of 28% [57]. In a study by Hong et al. [61] examining the incidence of venous gas embolism, they found a higher incidence of VAE in radical retropubic prostatectomies (80%) versus robotic-assisted prostatectomies (38%). However, there were no signs of cardiorespiratory instability defined as the appearance of cardiac arrhythmias, sudden decrease in mean arterial pressure >20 mmHg, or an episode of a pulse oxygen saturation of <90%. Non-validated but commonsense recommendations to decrease the incidence of VAE are a careful surgical technique for needle induction of pneumoperitoneum, low intra-abdominal pressure, and low insufflation rates. This diagnosis requires a high index of suspicion and rapid action by the anesthesiologist for successful treatment. If there is a drop in end-tidal carbon dioxide concentration, tachycardia, peaked P waves, and hypertension followed quickly by hypotension during insufflation, VAE is to be considered. The most common time that VAE occurs is during the creation of pneumoperitoneum. The most sensitive method for detecting a gas embolism is transesophageal (TEE) Doppler monitoring [62]. Because VAE is a rare event, routine TEE monitoring is not necessary and an abrupt decrease in end-tidal carbon dioxide is a reliable indicator. Treatment requires release of intra-abdominal pressure and maintenance of a head-down position. Turning the patient to the left lateral decubitus position is practically difficult especially if the patient is docked to the robot.
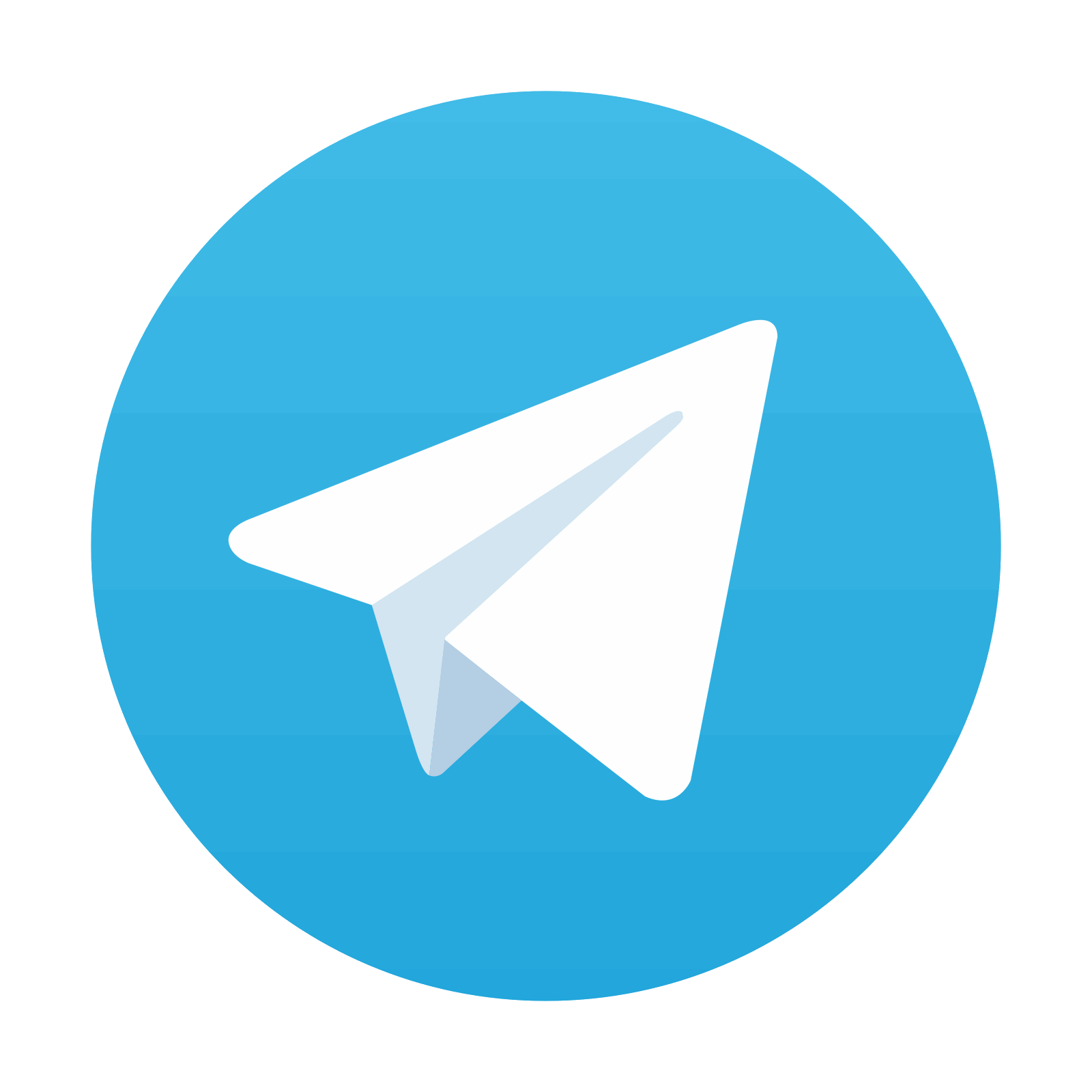
Stay updated, free articles. Join our Telegram channel

Full access? Get Clinical Tree
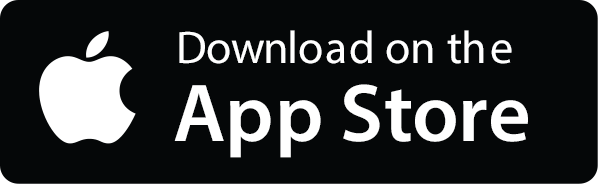
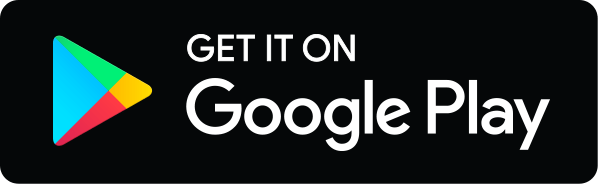