Chapter 15
Anesthesia Equipment
“To a large extent, anesthesia machines are inherently dangerous. A machine that has the power to induce anesthesia necessarily has the power to cause death and serious injury. An anesthesia department may want to institute periodic training to make sure that everyone in the department is familiar with all of the machines in the hospital.”1 It would disturb any of us to read in the news about an adult, without driver’s training, who attempted to drive a car and caused injury or death as a result. Shouldn’t it be equally disturbing to learn that only a minority of anesthetists report that they check their gas machines before use?2,3 Because anesthesia equipment malfunction is so rare, misuse and human error are much more of a threat to patient safety than outright malfunction.4–7 However, when errors in the use of gas delivery equipment occur, the outcome is often mortality or serious morbidity.4 Human error can be most effectively combated by a strong individual, department, and industry commitment “to ensure that no patient is harmed by anesthesia” (the mission of the Anesthesia Patient Safety Foundation).8 The means of accomplishing this goal are educational and motivational. We anesthetize patients with many different kinds of problems, for many different kinds of surgical and diagnostic procedures. But there is one thing that is present in every anesthetic: the anesthesia gas machine. It is the purpose of this chapter to provide timely, accurate, and patient-safety-focused information about this important and ubiquitous patient-safety technology.
Several factors make learning about gas machines difficult. Each model has unique aspects—even those from the same manufacturer. It is not always possible for the anesthetist to be available when department in-service education is conducted. Also, continuing education content may be too limited, or an opportunity to use new equipment soon after an educational session may not occur. New equipment constantly appears in the anesthesia work area. Although anesthesia equipment is designed to meet all legal and technical requirements, the designers of the equipment are not users. Some designs may be recognized as flawed only when they are used clinically. Instructional materials accompanying equipment often are inadequate. For example, no matter how well written, supplying one instruction manual per machine is inferior to supplying one instruction manual per user. The potential lack in equipment competency caused by these factors can be a safety problem.9–11 Users may be legally obligated to know and follow manufacturers’ instructions (checklists, operating manuals) and warnings, because these may contribute to the standard of care. Some courts have defined deviation from manufacturers’ instructions as prima facie negligence.12
To make learning the content easier, this chapter views the machine from a systems approach. For example, all machines have systems to provide (and measure) gas composition, including life-sustaining gases (oxygen), anesthetizing gases, and metabolic by-products (carbon dioxide). When this capability of every gas machine is understood, one need only determine how a particular machine accomplishes this, and how this function is checked before use to operate the machine safely (in this respect). Once all the systems—and their interplay—are understood, learning new equipment is easier. High-fidelity patient simulation is a novel approach that holds promise for both learning anesthesia equipment more efficiently, and studying gas-machine hazards. Simulation has allowed exploration of questions that could not be studied in the past because of risks to patients.13,14
Organization of the Anesthesia Gas Machine
Supply, Processing, Delivery, and Disposal Model
The SPDD model is depicted in Figure 15-1 and Box 15-1. This model is comprehensive in that the path of gases can be followed from their arrival in the operating room to their disposal from it. Most anesthesia gas-machine components can be located easily within the overall scheme. Gas flows in the diagram proceed generally from left to right. The vertical bar separates components within the machine and proximal to the common gas outlet (left side), from external components downstream from the common gas outlet (right). The fact that nitrous oxide and air, unlike oxygen, have only one task in the machine is easy to appreciate. The five tasks of oxygen are easy to follow. Understanding the similarities and differences between the fail-safe and hypoxic guard systems is facilitated. The model makes clear that the scavenging system, rather than the patient, is the ultimate destination of gases. Oxygen is central to the figure, because it is the most essential gas delivered. The reader should note that not every component of the SPDD model in Box 15-1 is depicted graphically in Figure 15-1. The reader should make frequent reference to the model while reading this chapter.
The model organizes the information on the basis of how components are used, rather than on the pressures to which they are exposed. From the viewpoint of pressure that components are exposed to, components are classified as part of the high-, intermediate-, or low-pressure systems within (proximal to the common gas outlet) the gas machine (Box 15-2).
Introduction of new gas machines raises questions about the adequacy and safety of older equipment.1,15 One can determine whether current equipment is obsolete by considering how closely it meets current safety standards and practice needs, by the availability of service, and by the presence of essential safety features.16,17 Certain systems or components are required by the anesthesia workstation standard, which is a voluntary industry-group consensus standard (Box 15-3).18
Supply
Pipeline Supply
Oxygen is produced by the fractional distillation of liquid air. It is delivered to facilities and stored as a liquid at a temperature of minus 184° C.19 The liquid oxygen is converted to a gas and supplied to hospital pipelines at a pressure of 50 psi (344 kPa). In the operating room suite, main and partial-area shutoff valves are present to isolate sections with leaks, interrupt supply in case of fire, and allow repair work on subsections. Wall outlets or hoses dropped from the operating room ceiling are finished with quick-connect couplers. These couplers are used so that the connection of gas machine supply hoses to wall outlets does not require tools. However, the springs and rubber gaskets (O-rings) these couplers contain provide a connection that is less secure than a wrench-tightened connection; thus they are a common source of leaks.20–22
Systems processing nitrous oxide are similar. Nitrous oxide is delivered to the hospital in large (size H) cylinders, which are connected to a manifold. Regulators reduce the pressure so that nitrous oxide, like oxygen, is supplied to the pipelines at 50 psi.19 Consequently, 50 psi is the normal working pressure of the anesthesia gas machine. Shutoff valves and wall outlets with quick-connect couplers are similar for nitrous oxide and oxygen. Delivery piping for both nitrous oxide and oxygen uses the diameter index safety system (DISS) to prevent misconnections. In this system, gas-piping connections are sized and threaded differently so that cross-connection is difficult, though not impossible (Figure 15-2).20,23
Supply hoses connect the pipeline inlets on the back of the machine to the wall outlets. At the pipeline inlet, a filter, check valve, and pressure gauge are present. The check valve ensures unidirectional forward flow, so that a machine running on cylinder supplies, with the hoses disconnected at the wall outlet, does not leak (Figure 15-3). The filter is required by the current anesthesia workstation standard (ASTM-F1850) because it may help prevent damage to the anesthesia gas machine from particulate matter present in the pipeline gas supply.18,24
Problems with Pipeline Supply
Some of the problems associated with pipeline use can be particularly dangerous because they are occult. Pressure loss, excess pressure, cross-connection of gas delivery pipelines, contamination, leaks, and theft of nitrous oxide (for recreational use) have been reported.25 These problems continue to be reported, and they have consequences for patient safety. There were 45 deaths related to pipeline problems in the United States from 1972 to 1993, and this number is probably an underestimate.19 Two patients became hypoxemic during anesthesia due to delivery to a hospital of nitrogen tanks with oxygen fittings in 1996.23 Seven deaths related to piped medical gases were reported from 1997 to 2001.26 Two patients became hypoxemic due to purging of oxygen lines with nitrogen in 2000.27 A serious failure of the bulk liquid oxygen supply was noted in 2004, but no patients were harmed.28 Complications can arise if oxygen analyzers fail or are misused.2,11,29 Always trust an oxygen analyzer reporting a low inspired oxygen, until you can prove it wrong.
Pipeline supplies of gas have been reported to contain particulate, gaseous, and bacterial matter; other contaminants; and water.24,25,30,31 In 1993 the National Fire Protection Association (NFPA) adopted more stringent testing standards. The Joint Commission has established standards that allow site visitors to randomly approach and question operating room staff to ensure they are aware of the location and function of shutoff valves, and alarms related to gas supplies in their area.30
Loss of Oxygen Pipeline Pressure
Loss of oxygen pipeline pressure is indicated by the pipeline pressure gauge. In addition, if pressure loss is profound, the oxygen low-pressure alarm sounds, and the fail-safe valves halt the delivery of all other gases. Some newer machines are designed to switch to air to drive the ventilator bellows with loss of oxygen pipeline pressure. With the new electronic alarms, operator response may be delayed because the electronic alarms lack the distinctive and familiar “whistle” of a pneumatic oxygen low-pressure alarm.32
Two recent simulation studies point to the need for change in the way we teach and respond to loss of oxygen pipeline pressure.33,34 In a simulated loss of pipeline oxygen, most residents recognized the oxygen-loss alarms. But less than half knew how to change an empty oxygen cylinder, attempted to change it (even after prompting), or recognized that bag-valve-mask (Ambu) ventilation would lead to patient awakening.33 A group of volunteer specialist anesthetists (completed residency and in practice) managed a simulated oxygen pipeline failure equally poorly.34 In their pre-use check, 70% failed to identify an empty oxygen cylinder, and only 25% checked for backup ventilation equipment (a bag-valve-mask) before induction. Most failed to conserve cylinder oxygen (by using low fresh gas flows, and turning off the mechanical ventilator), and all used untested pipeline supplies of oxygen when informed that pressure had been restored.34 These responses could be categorized as unwise at least, or unsafe at worst. In a third recent report, more than 700 anesthetics were given over a 3-week period without any checks that the emergency oxygen cylinder was full.35
Optimal management of loss of oxygen pipeline pressure has the following goals: maintenance of oxygenation, ventilation, and depth of anesthesia; and ensuring the safety of the oxygen supply.34 Box 15-4 gives a suggested guideline for management.28,33,34,36–39 With complete loss of oxygen pipeline pressure, the anesthetist should fully open the E cylinder of oxygen, disconnect the pipeline, and consider the use of low fresh gas flows and manual ventilation to conserve the emergency cylinder supply of oxygen. If the E cylinder of oxygen is not opened fully, flow from it may end before the cylinder is empty. The author recommends disconnecting the hose at the quick-connect fitting at the wall during an oxygen pressure-loss event, when doing so is not strictly necessary, for two reasons. First, it must be disconnected in the case of a cross-connection (which is more fully described later in the chapter), otherwise the contents of the oxygen cylinder will not flow. Remembering one strategy that is effective for two reasonably similar problems is easier than remembering two different strategies. Second, if the loss of pipeline pressure is followed by the flow of contaminated contents from the oxygen pipeline, disconnecting when pipeline pressure is lost protects the patient from exposure to these contaminants.
Cross-Connection of Gases
Cross-connection of gases can occur anywhere from the liquid oxygen supply and piping, to the wall outlets, hoses, and internal circuitry of the anesthesia gas machine. Incidents of cross-connection continue to be reported. Mishaps in which cross-connection was a factor have been reported as recently as 2009.40–43 Fatalities (as recently as 2002) have been associated with the shipment in error to the hospital of liquid nitrogen instead of oxygen, liquid carbon dioxide rather than nitrous oxide, the unintentional cross-connection of oxygen and nitrous oxide pipelines during renovation of an operating room, and alteration of an oxygen flowmeter so that it would fit a nitrous oxide outlet in a cardiac catheterization laboratory. A common factor associated with patient injury has been failure to use an oxygen analyzer.
Although not all of the above incidents involved patients connected to anesthesia gas machines, cross-connections also continue to be reported in anesthetized patients. In 1997 a case was described in which the nitrogen hose from the gas machine was discovered to be fitted with a quick-connect coupler for air (at the end that would be plugged into the wall outlet).23 This did not result in patient injury, but would have allowed the delivery of 100% nitrogen had the machine been set to deliver air only. The consequences of this type of error in the oxygen wall-outlet hose could be disastrous. In 1995 it was reported in the United States that two patients became hypoxemic as a result of delivery of liquid nitrogen to the hospital in a tank with oxygen fittings.44 In 2009 a wall fitting for carbon dioxide in a lithotripsy area allowed connection of a nitrous oxide hose from the gas machine, resulting in end-tidal carbon dioxide over 105 mmHg.40 These incidents underscore our susceptibility to errors of ancillary personnel who install and test gas delivery apparatus. But they also highlight many patient-safety aspects, which anesthetists do control: properly checked oxygen analysis monitors, correctly checking the gas machine before use, and proper response to oxygen-analyzer alarms.
In the event of a suspected crossover, one would see declining inspired oxygen concentration. The anesthetist must open the emergency cylinder oxygen supply, disconnect the pipeline, and use low fresh gas flows and manual ventilation. If the pipeline is not disconnected, the pipeline gas will continue to flow, rather than the cylinder oxygen supply. This is because the pressure distal to the cylinder regulator is set at 45 psi (310 kPa), compared with the typical pipeline pressure of 50 psi (344 kPa). Lower pressure is intentionally set on the cylinder regulator so that flow proceeds from the higher-pressure pipeline source if a cylinder is inadvertently left open after the machine has been checked.45 This is analogous to the situation with an intravenous main line and a piggyback line: whichever is held higher is the one that will flow (greater hydrostatic pressure). In the case of cross-connection between oxygen and nitrous oxide pipelines, the contents of the oxygen pipeline (now nitrous oxide) continue to flow (because the pipeline pressure is 50 psi), whether or not the oxygen cylinder is open. Thus, regardless of the problem with the pipeline supply (lack of pressure or cross-connection), the cylinder must be opened; the author advocates disconnecting the pipeline in any instance of problems with the pipeline. If the pipeline is not disconnected and has pressure within it, the emergency supply of oxygen may not flow from the cylinder.
Cylinder Supply
Cylinders are present on the anesthesia machine as reserves for emergency use. Thus they should be open only when they are checked, or when the pipeline supply is unavailable.46 A fresh oxygen cylinder need not be obtained if the cylinder on the machine has an adequate pressure, the amount of which would depend on the availability of pipeline supplies and additional backup oxygen cylinders (see later in chapter under PreAnesthesia Checklist). Cylinders are labeled, marked, and color coded (Table 15-1).18,47 Anesthetists who practice outside the United States must be aware that the color scheme may differ from country to country. Service pressure and cylinder contents are reported slightly differently in various sources.48
TABLE 15-1
∗Note that slightly different values may be found in different sources.
Data from Dorsch JA, Dorsch SE. A Practical Approach to Anesthesia Equipment. Philadelphia: Lippincott Williams & Wilkins; 2011; Standard Specification for Particular Requirements for Anesthesia Workstations and Their Components [F1850-00]. Philadelphia: American Society for Testing and Materials; 2005; NFPA 99: Health Care Facilities [Table 5.1.11]. Quincy, Mass: National Fire Protection Association; 2012:49.
Pin position refers to the pin index safety system (PISS) illustrated in Figures 15-4 and 15-5. In this system, each cylinder valve has a unique arrangement of holes that corresponds to its intended contents. The holes mate with pins in the yoke, which is the point where cylinders are attached to the gas machine. The PISS is thus another means of preventing misconnections. The system can be defeated if the pins are missing, are removed, or if more than one washer is used. Anesthetists should check both pins and washers whenever cylinders are replaced. Furthermore, they should be aware that not all E cylinders are of precisely the right size to fit properly on the machine. Installation of a longer aluminum cylinder has interfered with the casters and prevented an anesthesia gas machine from being moved.49
The cylinder valve is the most fragile part of the cylinder, so it must be protected during transport. The cylinder valve consists of a body, the port where gas exits, a conical depression (opposite the port) for the securing screw, the holes where the pins on the yoke fit, and safety relief devices. If a fire causes the temperature and pressure within the cylinder to increase, safety relief devices release cylinder contents in a controlled fashion, rather than explosively. Manufacturers use one or more of the following on cylinder valves: a frangible disk that bursts under pressure, a valve that opens at extreme pressure, or a fusible plug made of Wood’s metal (which melts at elevated temperatures).
The hanger yoke serves three functions: it orients cylinders, provides a gas-tight seal, and ensures unidirectional flow into the machine. It also contains a filter that is required by standard.18 The unidirectional valve within the hanger yoke minimizes the likelihood of transfilling, or of leakage to the atmosphere (if a yoke is empty). It also allows cylinders to be replaced during use. If two cylinders are open, transfilling occurs when gas flows from the cylinder with higher pressure into the cylinder with lower pressure, rather than proceeding toward the flowmeters. Transfilling is a potential fire hazard because filling a cylinder generates heat. The cylinder pressure gauge is a Bourdon-type gauge (Figure 15-6).
Immediately distal to the hanger yoke for each gas is a regulator (Figure 15-7). Two diaphragms move together, connected by a rod. The smaller of the two diaphragms opens or closes the high-pressure inlet (from the cylinder). Gas entering the regulator exerts pressure on the larger diaphragm, whose movement tends to close the inlet. Thus gas can enter the regulator only at a rate controlled by a feedback loop. The outlet pressure is adjustable by a screw and spring that bear on the inlet diaphragm. Thus the regulator converts the high (but variable) cylinder pressure to a constant downstream pressure (45 psi [310 kPa]), which is intentionally slightly less than pipeline pressure in order to prevent silent depletion of cylinder contents.18,30,32 Pipeline pressure varies, depending on the load that is placed on it throughout the facility. If a cylinder is left open, and pipeline pressure drops below 45 psi, gas will flow from the cylinder. No alarm will sound to warn the user of this condition.25 Further, if the cylinder is left open after checking and the pipeline fails, the operator will not be alerted to the failure at the time it occurs, because gas will simply begin to flow from the cylinder, without alarms. If the mechanical ventilator is in use, a full E cylinder of oxygen may be depleted in as little as 1 hour, because the ventilator-driving gas in a bellows ventilator is usually oxygen.36,50 The low oxygen supply failure alarm that rings subsequently announces the end of the emergency supply, instead of its beginning. This is the rationale for keeping cylinders closed after their pressure has been checked.
The U.S. Department of Transportation issues regulations for the manufacture, handling, transport, storage, and disposal of cylinders. These regulations have binding legal force. Industry advisory groups such as the Compressed Gas Association (CGA) and the National Fire Protection Association also have a role in setting cylinder standards. Cylinders are constructed of steel approximately ¼ inch in thickness. Only nonferrous (aluminum) cylinders may be used in the magnetic resonance imaging (MRI) environment.48 Fatalities have occurred when steel cylinders have impacted patients in the MRI scanner.51
Anesthetists must be aware of the rules for the safe handling and use of cylinders.25,52 Gas under pressure in cylinders has an enormous potential energy, which may be lethal if it is released in a rapid, uncontrolled fashion following damage to the cylinder valve. Selected rules for safe handling of cylinders are presented in Box 15-5.
When installing a cylinder, check the labels, crack the valve, check that both PISS pins are present, check that only one washer is present, place the cylinder in the hanger yoke, observe for the absence of an audible leak, and check for proper gauge pressure. The valve is “cracked” to remove dirt from the port. This is done by opening the valve briefly and carefully before the cylinder is placed on the machine. While cracking the valve, hold the cylinder securely, and do not point the port toward oneself or other personnel.
Remember to consider the oxygen flow rate set on the flowmeter when deciding how long the available liters will last. As an example, if the oxygen flow is 2 L/min, and the cylinder’s oxygen gauge pressure is 500 psi, how long will the cylinder last? From Table 15-1, we know that the service pressure is 1900 psi and that the capacity is 660 L. Substituting these values into the previous relationship, we obtain the following:
Because 2 L of oxygen flow each minute, the cylinder will last approximately 87 minutes (174 L ÷ 2 L/min). This type of calculation is not applicable to compressed gases stored as liquids (nitrous oxide or carbon dioxide). It should be remembered that this calculation refers only to requirements at the flowmeters, and assumes manual ventilation. Use of a mechanical ventilator consumes approximately a minute volume of driving gas each minute, and thus should be avoided in situations in which oxygen supply is limited to cylinders only.50
Nitrous oxide is stored as a liquid; therefore the cylinder pressure of 745 psi (5136 kPa) represents the vapor pressure of liquid nitrous oxide at room temperature. The nitrous oxide cylinder pressure gauge remains at 745 psi until the liquid is gone; at this point, the cylinder is more than three-quarters empty. After this point, the nitrous oxide cylinder pressure swiftly declines with further use. Thus nitrous oxide cylinders should be changed if their pressure is less than 745 psi. Rapid removal (more than 4 L/min) from a cylinder may cause the formation of frost on its wall or freezing of the valve, owing to the loss of the latent heat of vaporization from the liquid nitrous oxide. Nitrous oxide is nonflammable, but it does support combustion.25,48 Anesthesia personnel must be alert to the possibility of nitrous oxide abuse.53
Electrical Power Supply
Electrical power is supplied to the gas machine through a single power cord, which can become dislodged. Because of this possibility, as well as the possibility of loss of main electrical power, new gas machines must be equipped with battery backup sufficient for at least 30 minutes of limited operation.18 Which systems remain powered during this period is specific to each model, thus users must read the operator’s manuals. For example, if you disconnect electrical power from the ADU (anesthesia delivery unit [GE Healthcare]) it loses patient monitors (electrocardiogram, noninvasive blood pressure, gas analysis, pulse oximetry, and other monitors displayed on the right screen), but fresh gas flow, volatile-agent delivery, and ventilation continue during the period that battery backup is used. Once battery power is lost, nitrous oxide and agent delivery are cut off.54
Convenience receptacles are usually found on the back of the machine so that monitors or other equipment can be plugged in. These convenience receptacles are protected by circuit breakers or fuses. It is a mistake to plug devices that convert electrical power into heat into these receptacles (air or water warming blankets, intravenous fluid warmers) for two reasons.55 First, these devices draw a lot of amperage (relative to other electrical devices), so they are more likely to cause a circuit breaker to open. Second, the circuit breakers are in nonstandard locations (so check for their location before your first case). If a circuit breaker opens, all devices that receive power there (such as monitors and, in some configurations, the mechanical ventilator) may cease to function. If one is not familiar with the circuit-breaker location, valuable time may be lost while a search is conducted.
Loss of Main Electrical Power
Generally, hospitals have emergency generators that will supply operating-room electrical outlets in the event power is lost. But these backup generators are not completely reliable. A 90-minute interruption in power during cardiopulmonary bypass, complicated by almost immediate failure of the hospital generators, has been described.56 Injury to personnel as they hurried in the dark to fetch lights and equipment was an unanticipated hazard.
With power failure in older gas machines, the principal problems were loss of room illumination and failure of mechanical ventilators and electronic patient monitoring. In general, new gas machines have battery backup sufficient for 30 minutes of operation—however, typically without patient monitors (e.g., electrocardiogram, pulse oximetry, gas analysis). Mechanical ventilation may or may not be powered by the backup battery (depending on the model). New flowmeters that are entirely electronic (Aisys and Avance [GE Healthcare]) require a backup pneumatic/mechanical (needle-valve and flowtube) flowmeter.15,57–59 Mechanical flowmeters with digital display of flows have a backup glass flow tube that indicates total fresh gas flow (ADU54 [GE Healthcare]; Fabius GS60 and Apollo [Dräger Medical]).
New gas machines with mechanical needle valve flowmeters and variable bypass vaporizers (e.g., Fabius GS, Apollo, or Aestiva [GE Healthcare]) have an advantage during electrical power failure in that delivery of gases and agent can continue indefinitely.46,60 However, in the event of generator failure, anesthesia would be limited to flashlight illumination and monitoring by the five senses. The Apollo (Dräger Medical) provides gas and vapor delivery, mechanical ventilation, and integrated monitoring (e.g., oxygen, breathing circuit volume and pressure, gas analysis) for 30 minutes or more if main electrical power is lost.61,62 Patient monitors will not function on battery power. Pneumatic functions remain even after the battery is exhausted: vaporizers, S-ORC (fail-safe and hypoxic guard), adjustable pressure-limiting (APL) valve, flowmeters, breathing pressure gauge, cylinder and pipeline pressure gauges, and total fresh gas flowmeter.
Processing
Manufacturers and Models
Fabius GS
The Dräger Fabius GS (Figure 15-8) includes volume, pressure, flow, and inspired oxygen monitoring; but not physiologic monitors or gas analysis. The thermal anemometry (“hot wire”) flow sensor in the breathing circuit is unique to this machine and a few others.60 The screen displays tidal and minute volume, respiratory rate, and a respiratory pressure waveform.
The ventilator is piston driven; corrects tidal volume for compliance and leaks; and features manual, spontaneous, volume-controlled ventilation (VCV); pressure-controlled ventilation (PCV); pressure-support ventilation (PSV); and synchronized intermittent mandatory ventilation (SIMV) with PSV.60 Like the Apollo, the mechanical ventilator is activated in two steps (the mode is chosen, and then confirmed by a second key press). The machine uses a manual checklist with several electronic self-tests (e.g., system, leaks and compliance, flow sensor, oxygen sensor). With the Fabius GS (as with all the new models), users must review the operator’s manual to check the machine correctly. Sample preanesthesia checkout procedures are available.63 The flowmeters are needle valves with electronic capture and display, with a common gas outlet glass flowmeter as backup. Variable-bypass vaporizers are used, and these may be removed without tools.
The Fabius GS breathing circuit is lower volume than older gas machines (2.8 L plus bag; of which 1.2 L is absorbent volume).64,65 The absorber head is not warmed. Loose carbon dioxide absorbent granules or prefilled canisters may be used. There is an open scavenger interface. Fresh gas decoupling causes the manual breathing bag to fluctuate during the mechanical ventilator cycle, which serves as a further disconnect alarm.
Narkomed 6000/6400
The Dräger Narkomed 6000 (Figure 15-9) includes volume, pressure, flow, and inspired oxygen monitoring. It also includes gas monitoring (infrared agent and carbon dioxide) and an ultrasonic flow sensor in the breathing circuit (unique to this machine). An integrated patient monitoring module is available as an option, so all parameters are displayed on the single-touch screen.66
From a cold startup, there is a 1-minute power-on self-test, then a 5-minute ventilator self-test.66 One can bypass the ventilator test for 10 days (or 10 times) only, after which the ventilator is unavailable until its self-test is performed. The machine checkout, as with most newer machines, is unique is some respects. For example, the manufacturer recommends breathing through each circuit limb to test unidirectional valves and disconnecting the oxygen wall hose to check the oxygen pipeline pressure-failure device. Sample checkout protocols are available.63
Flowmeters are composed of needle valves and glass flowtubes, but electronic capture of fresh gas flows has been added in the 6400. Variable-bypass vaporizers are used, and these may be removed without tools. The breathing circuit is lower volume (1.5 L absorbent volume), and the absorber head is warmed.66 Only loose carbon dioxide–absorbent granules may be used. An open scavenger interface or passive evacuation may be used. In case of power failure, there is a 30-minute battery reserve with fresh gas, vaporizers, monitors, and ventilator operational.
Apollo
The Dräger Apollo (Figure 15-10) includes volume, pressure, flow, and inspired oxygen monitoring. It also includes gas monitoring (agent and carbon dioxide). Spirometry is optional.15,61,62 The piston ventilator corrects tidal volume for leaks, compliance, and fresh gas flow (by fresh gas decoupling). Like the Fabius, the mechanical ventilator is activated in two steps (the mode is chosen, and then confirmed by a second key press). The ventilator is capable of manual, spontaneous, VCV, PCV, PSV, and SIMV modes. SIMV may be used in either volume or pressure modes. It is accurate to very low tidal volume (range 20 to 1400 mL).62,67
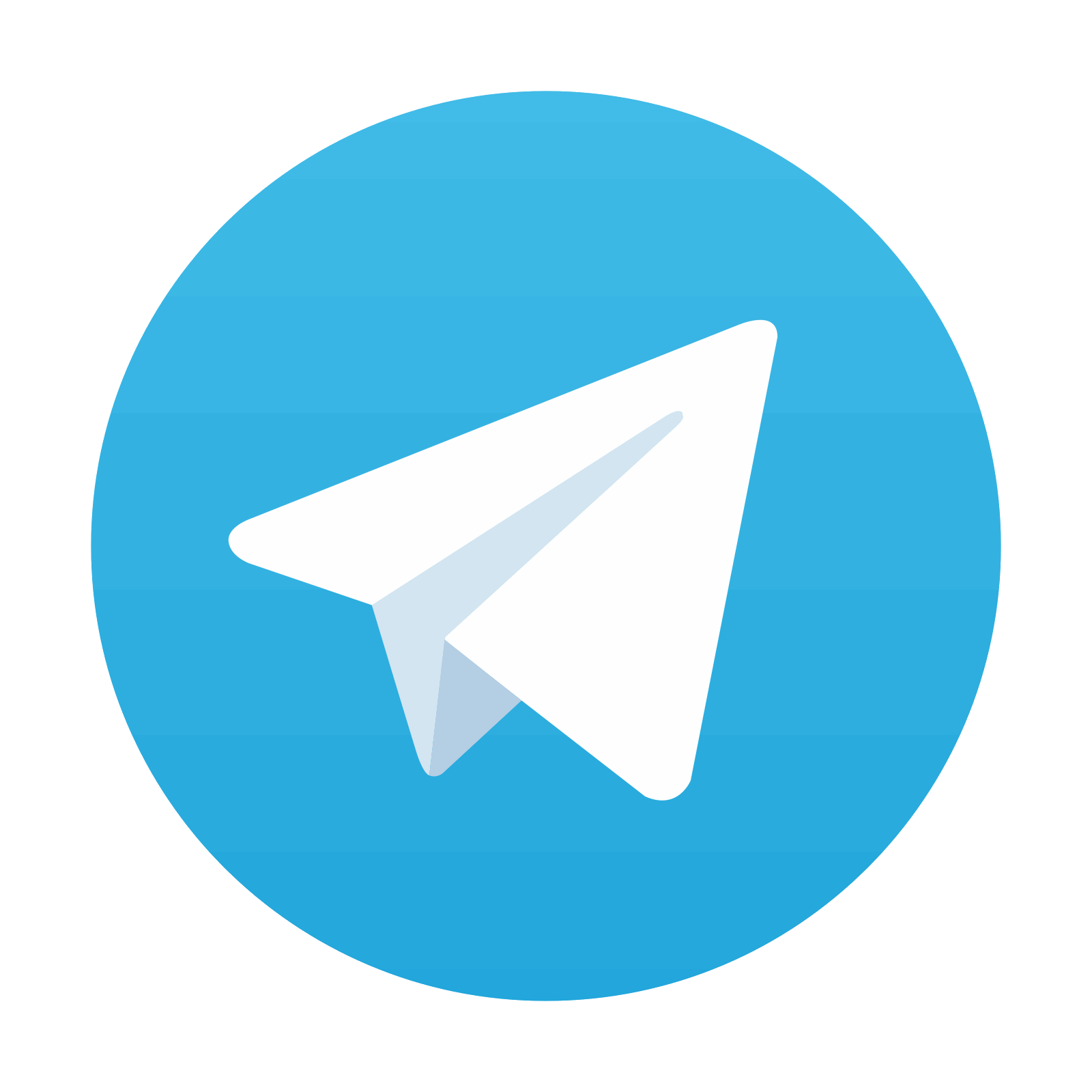
Stay updated, free articles. Join our Telegram channel

Full access? Get Clinical Tree
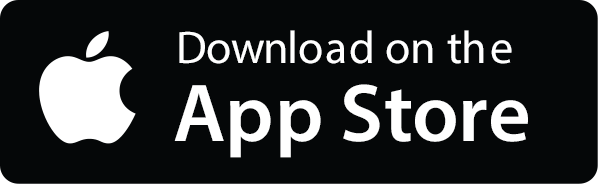
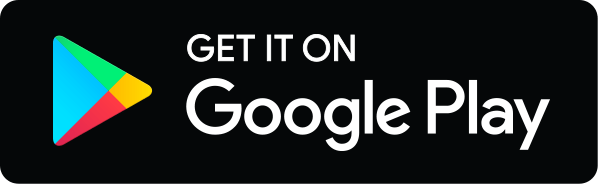