Introduction
As surgical and anesthetic techniques have evolved, perioperative mortality in complex cancer surgical interventions has decreased significantly. However, complications and associated morbidity remain a challenge not only for surgical recovery, but also for functional restoration, completion of care for the patient, and long-term cancer outcomes. As such, optimization and preparation for major cancer surgery (prehabilitation) should be a high priority safety initiative and a fundamental strategy in perioperative medicine. Appropriate preparative management or optimization of functional capacity prior to an anticipated stressor can mitigate adverse outcomes, which carry substantial clinical and economic implications. Pivotal strategies with substantial impact include hematological optimization (including the correction of iron deficiency and anemia), appropriate use of blood products, and prevention of thromboembolic complications.
Blood loss is an inherent risk with more complicated cancer surgeries. Moreover, even prior to surgery, anemia and iron deficiency are common findings and remain important contributors to adverse postoperative outcomes and mortality. Equally, perioperative allogeneic red cell transfusions, which remain the most common strategy to correct anemia, are an independent predictor of postoperative complications and have been linked with an increased risk of cancer recurrence and reduced overall survival (OS).
Prevention of thromboembolism (TE) has been a high priority safety initiative for over a decade. TE is a common complication among patients with cancer, particularly in the postoperative period, and a leading cause of preventable morbidity and mortality. Yet systematic real-time approaches to TE prevention, particularly postsurgery, remain suboptimal.
Here we review the burden of these important contributors to outcomes in patients with cancer in association with the perioperative period. In addition, we propose pragmatic, expert, and evidence-based strategies that can be easily implemented and scaled in real-time to patient care.
Anemia
Anemia is a symptom of erythropoietic failure, reflecting inadequate substrate (particularly iron) or red cell production that is exceeded by loss. These processes frequently coexist in cancer patients and represent a continuum; even in the nonanemic patient, iron deficiency may still be present, impacting on skeletal muscle and respiratory chain function. Patients with cancer about to undergo major surgery require assessment of both hemoglobin and iron status and consideration of appropriate preoperative optimization prior to proceeding.
Burden of Anemia and Iron Deficiency in Cancer Patients
Iron deficiency and anemia contribute to cancer-associated morbidity. These reflect blood loss (particularly in tumors of the gastrointestinal tract) and the inflammatory response to the tumor and neoadjuvant therapy. The European Cancer Survey highlighted the prevalence of anemia in different cancer types; 39% of patients were anemic at time of diagnosis, with 68% becoming anemic within 6 months of starting treatment, a reflection of the additive effects of multiple rounds of chemotherapy and/or surgery.
Emerging evidence suggests that iron deficiency, even in the absence of anemia, should be considered an actionable pathology in its own right. Data on the prevalence of iron deficiency as an independent laboratory abnormality in the oncological population are highest in the colorectal cancer population, ranging from 50%–60% in some series, as a reflection of chronic blood loss. , However, even in patients where ongoing bleeding is not a hallmark of the malignancy, iron deficiency is also found in up to 46% of cases.
Pathophysiology of Anemia in Cancer
Causes of anemia can be divided into three categories:
- 1.
Blood loss;
- 2.
Increased red cell destruction;
- 3.
Decreased red cell production.
Although certain tumors enable chronic, ongoing blood loss, most cancer-associated anemia is caused by diminished red cell production and is often due to iron deficiency ( Fig. 8.1 ). Broadly speaking, iron deficiency can be defined as:
a lack of stored iron due to chronic losses (absolute iron deficiency), and
inability to access stored iron (functional iron deficiency).

Functional iron deficiency is a consequence of the inflammatory response. A key contributor to the associated “anemia of inflammation” is dysregulation of the cytokine interleukin-6 (IL-6). Overexpression of IL-6 has been found in almost all types of tumor, promoting tumorigenesis, suppressing erythropoietin, and increasing expression of the iron regulatory hormone hepcidin. Hepcidin downregulates ferroportin-1, a transmembrane protein that enables iron to be transported across cell membranes. Consequently, iron cannot be absorbed from the gut, and stored iron cannot be accessed by bone marrow. This restricts erythropoiesis and limits the efficacy of oral iron supplementation. Additionally, overexpression of tumor necrosis factor-α (TNF-α) suppresses the hemoglobinization of erythroid progenitor cells, cytotoxic therapies impair hematopoiesis, and nephrotoxic therapeutics impact the production of erythropoietin. Consequently, perioperative risk is increased in patients who have undergone neoadjuvant treatment.
Influence of Anemia and Iron Deficiency on Outcome in Cancer Patients
Separate to the perioperative period, anemia is associated with poor outcome in cancer patients, both due to worsening of local tumor control and increased requirement for allogeneic blood transfusion. While some authors maintain that anemia is a marker of increased concomitant comorbidity, rather than an independent risk factor in its own right, major randomized controlled trial data are lacking. Most guidelines promote hemoglobin concentration as a therapeutic target prior to proceeding to major surgery.
Iron deficiency, independent of anemia, is gaining attention in the perioperative sphere. While clinicians often consider the two to be synonymous, physiological effects of iron deficiency (particularly fatigue and exercise intolerance) may be noted before hemoglobin drops below the anemic threshold. This is because iron is a key contributor to basic cellular function, with roles in energy metabolism, cell signaling, gene expression, and regulation of cell growth. Most iron is inserted into protoporphyrin IX as the final step in the production of heme to allow oxygen binding to hemoglobin. Additionally, iron serves as a prosthetic group for other oxygen-carrying molecules, notably myoglobin, cytochromes, and nitric oxide synthase, and is integral to the function of enzyme systems responsible for DNA synthesis and repair, energy metabolism via the function of the respiratory chain, and production of ADP. Hence iron deficiency can impact respiratory chain and skeletal muscle function adversely, affecting fatigability and recovery from exercise. Early retrospective data from colorectal cancer populations have highlighted a possible association between iron deficiency and poor postoperative outcomes independent of anemia. , Consequently, some consensus statements recommend correction of iron deficiency in patients presenting to major surgery regardless of the starting hemoglobin concentration. Prospective evidence for this practice is limited.
Diagnosing Iron Deficiency in the Preoperative Cancer Patient
Differentiation and identification of iron deficiency as part of a program of preoperative optimization can be challenging, as ferritin (an acute phase reactant) may be elevated for a variety of reasons, possibly reflecting cellular damage and leakage of ferritin from storage sites into the bloodstream. Indeed, serum ferritin in the normal reference range in the setting of a C-reactive protein greater than 5 mg/L will only be 39% sensitive for iron deficiency. An alternative metric is transferrin saturation (TSAT). Taken as the quotient of serum iron divided by transferrin concentration and expressed as a percentage, TSAT is a more direct measure of the physiological demand for iron (although it may still be affected by inflammation independent of iron status). A value of less than 20% is interpreted as being reflective of iron deficiency ( Table 8.1 ). Therefore a patient presenting for surgery with serum ferritin less than 100 µg/L regardless of TSAT, or a TSAT of less than 20% in the setting of serum ferritin at 100–300 µg/L, should be considered iron depleted.
Test | Normal Values | Comment |
Serum iron | >14 µmol/L | Little use for diagnosing iron deficiencySpecificity lost in inflammation |
Serum ferritin | 10–300 µg/L | Allows safe storage of iron in Fe 3+ formAppears in serum due to ICF leakageAcute phase reactant—cannot be used in isolation to determine iron status |
Transferrin | 2.0–3.5 g/L | Rarely used in isolation, but commonly combined with serum iron to determine transferrin saturation (TSAT) |
Transferrin saturation | ≥20% | Measure of binding capacity for ironObtained by dividing serum transferrin by serum iron |
More sensitive markers of iron deficiency have been described. These include soluble transferrin receptor, serum hepcidin, reticulocyte hemoglobin concentration, and percentage hypochromic red cells. Although these are increasingly being cited in best practice guidelines, they have not yet entered widespread use. ,
Management of Iron Deficiency and Anemia in the Perioperative Period
All patients undergoing cancer treatment should be managed for reversible causes of anemia. While the most recent iteration of some medical oncology guidelines advocates for treating a hemoglobin concentration of less than 110 g/L, guidelines pertaining to major surgery frequently target a hemoglobin concentration of greater than 120 g/L for women and greater than 130 g/L for men. Indeed, emerging evidence and opinion suggest that 130 g/L be targeted in all patients, regardless of sex.
Initial management of patients with preoperative anemia should involve a determination of iron status. Some sources recommend assessment of vitamin B 12 and folate, but the incidence of actual deficiency of these substrates in the preoperative patient is rare. Formal testing should not be undertaken without clinical suspicion (elevated mean corpuscular volume, megaloblastic changes on blood film, or evidence of malnutrition).
If an iron deficient state has been identified (TSAT of less than 20%), or the patient is felt to have inadequate iron stores to facilitate postoperative erythropoiesis (serum ferritin of less than 100 µg/L), treatment with iron should be pursued. It is important to note that for the reasons outlined previously, patients with an active malignancy may not be able to absorb iron across the bowel wall. Intravenous iron supplementation is preferred, especially as the newer iron preparations are extremely stable, with a low rate of adverse reaction. In order to facilitate incrementation of hemoglobin prior to surgery, current consensus opinion suggests that 3 weeks is required to see a meaningful increase in hemoglobin concentration, although evidence from orthopedic and cardiac surgical populations suggests intervention as little as 24 hours prior to surgery can yield some benefit. , The PREVENTT trial called into practice the routine administration of intravenous iron to patients with preoperative anaemia undergoing major abdominal surgery. In this randomized trial, no short-term (within 30 days postoperatively) benefit was observed in patients who received intravenous iron at a median of 14 days prior to operation, relative to those who received placebo. While a statistically significant reduction in late readmission was seen at 8-month follow up, together with an increase in hemoglobin concentration, this was a secondary outcome that the study was not powered to examine, and that occured in the setting of a negative primary outcome. At the time of writing, the results of this trial have not yet been translated to best practice guidelines, and routine administration of intravenous iron in patients with iron deficiency anemia prior to major surgery remains standard of care. An example nomogram for preoperative iron therapy is shown in Fig. 8.2 .

Exogenous erythropoietin and other erythropoiesis stimulating agents (ESAs) were historically avoided in patients with an active malignancy, as it was thought that erythropoietin promotes tumorigenesis. However, subsequent registry studies have not replicated this signal and recent clinical guidelines, particularly in North America, are beginning to recommend the use of ESAs in patients with chemotherapy-associated anemia and an apparently adequate iron status. , Perioperative guidelines, particularly those from Europe, have been more reluctant to recommend the routine use of these agents to improve preoperative hemoglobin concentration. Although systematic reviews have suggested that there is benefit to combining ESAs with iron therapy relative to iron therapy alone, there is undoubtedly an increased risk of venous TE that may be magnified in the presence of malignancy. Current FDA recommendations advocate for concomitant anticoagulation in patients receiving ESAs in a perioperative context, particularly where the hemoglobin concentration exceeds 120 g/L. A further important caveat in the use of these agents is the time required for effect, and a course of treatment of up to 4 months is frequently required to realize maximum benefit. Finally, there is considerable heterogeneity in the literature regarding the correct dose of ESAs, which has contributed to the uncertainty surrounding their use.
Transfusion- and Cancer-Related Outcomes
Clinical Evidence
Perioperative red blood cell transfusion (RBCT) remains a common strategy to manage acute anemia in patients with cancer. In this population, the rate of perioperative RBCT ranges from 9.4% to 90% depending on the type surgery, patient risk factors, and the lack of clear definitions on when transfusions should be applied perioperatively. Transfusion triggers in patients with cancer remain ill-defined and controversial. Hemoglobin concentrations of 70–100 g/L have been used; however, these do not necessarily reflect the delivery of oxygen to tissues. Therefore perioperative physicians still transfuse using poorly defined hemoglobin thresholds, or demonstrative hemodynamic instability and/or signs of organ hypoperfusion. A recent trial by de Almeida et al. found a negative impact on short-term outcomes when hemoglobin at 70 g/L was used to trigger RBCT, compared with a liberal transfusion (90 g/L) strategy in critically ill cancer patients. , In these patients, a trigger of 80 g/L could therefore be considered to initiate RBCT with a modified threshold in those with objective signs of organ hypoperfusion.
Transfusion-Related Immunomodulation and Cancer Outcomes
Blood products contain high concentrations of interleukins (IL-1β, IL-6, IL-8), chemokines, prostaglandin E, thromboxanes, histamine, leukocytes (particularly in nonleukoreduced units), growth factors, nonpolar lipids, proinflammatory lysophosphatidylcholines, CD40 ligand, and microparticles. The administration of blood products can therefore have particular profound effects on transfusion-related immunomodulation (TRIM) defined as:
- 1.
donor-specific transfusion-related immune suppression,
- 2.
“generalized” transfusion-related immune suppression.
The former primarily suppresses adaptive immunity; in contrast, the generalized form appears to be mediated by macrophages and neutrophils and has a significant suppressive impact on the innate immunity. TRIM alters the function of natural killer cells, reduces proliferation of T and B lymphocytes, increases activity of T regulatory cells, and impairs the maturation and antigen-presenting activity of dendritic cells. It has been proposed that infections and cancer recurrence would be a consequence of the “generalized” transfusion-related immune suppression, rather than the donor-specific form. The timing of transfusion may also have different effects on the inflammatory and immune response. Thus when blood products are administered during or immediately after surgery, the so-called systemic inflammatory response syndrome can be further exaggerated by transfusions delaying inflammatory resolution, which can also participate in the pathogenesis of transfusion-related adverse outcomes.
Age of Stored Blood and Cancer Recurrence
It has been theorized that the age of the blood may influence perioperative outcomes. Although biological impact theoretically exists, studies that have investigated the impact of blood storage duration on the survival of patients with cancer are limited. Cata et al. reported that neither the use of “new” allogeneic nor autologous RBCs had a beneficial effect on recurrence-free survival or OS in patients with nonmetastatic prostate cancer. In large cohort study of 27,591 patients with mixed malignancies, the administration of “old” blood defined as 28 days of storage or older also did not influence OS or cancer recurrence.
Impact of Blood Transfusions on Outcomes of Patients With Cancers
Perioperative RBCT remains common in patients undergoing head and neck cancer surgeries. Azmy et al. reported a transfusion rate of 11.2% in a cohort of 3090 patients undergoing neck dissection; however, this number can be doubled in those undergoing free-flap surgery. Two retrospective studies indicated that RBCT given perioperatively in patients with head and neck cancers were linked to reduced OS and increased tumor recurrence. , However, this negative association was recently disputed by Goubran et al. who studied 354 patients.
The rate of perioperative RBCT among patients with lung cancer is approximately 10% and an independent predictor of short-term postoperative complications. Luan et al. conducted a meta-analysis of retrospective studies that included 3588 patients undergoing surgery for primary lung malignancies. They assessed the impact on cancer-related outcomes and reported a negative association between RBCT, recurrence-free survival, and OS. A more recent observational study included 5709 patients and demonstrated a dose-dependent relationship between the number of units transfused and a negative effect on cancer progression and OS.
Current evidence on whether the administration of RBCs is an independent risk factor for esophageal and gastric cancer recurrence is also controversial. Boshier et al. conducted a meta-analysis and concluded that the administration of autologous RBCs, and a low transfusion volume (<1–2 RBC units), is associated with better survival rates than with allogeneic transfusion or >3 RBC units transfused. However, a more recent retrospective study has demonstrated (after using propensity score matching) that perioperative blood transfusions are not an independent risk factor of reduced OS.
Evidence from two meta-analyses by Amato et al. that included randomized controlled trials and observational studies demonstrates that RBCT in patients with colorectal cancers had a negative impact on survival regardless of timing (pre-, intra-, or postoperative), type (allogeneic or autologous), leukodepletion status, and number of RBCT units. , In patients with pancreatic and hepatocellular carcinoma, RBCT is also associated with negative perioperative outcomes, including increased risk of death, recurrence, and perioperative complications. Tai et al. reported a rate of RBCT after liver resections for hepatocellular carcinomas of 42%, and the risk of dying from any cause, including cancer, was almost doubled compared to nontransfused subjects. Shiba et al. reported the impact of the transfusion of fresh frozen plasma (FFP) after surgery for pancreatic ductal adenocarcinoma. Patients receiving FFP had a significantly shorter OS.
A negative impact of autologous blood transfusions on cancer progression was not observed after colorectal metastatic liver resections ; however, this was observed with FFP. Nakaseko et al. reviewed the outcomes of 127 patients who had colorectal metastatic liver resections; those who received FFP (24%) had a twofold higher risk of dying of cancer or any cause than those who were not transfused.
Regarding cholangiocarcinoma, a meta-analysis by Wang et al. reported that the administration of RBCTs negatively affected the OS, while a more recent retrospective study using a propensity score matching method did not demonstrate negative survival outcomes.
Multiple studies have assessed perioperative RBCTs in patients with urological malignancies and demonstrated increased cancer recurrence and decreased survival. In patients with bladder cancer who underwent radical cystectomy, two meta-analyses suggested an association. Furrer et al. investigated the effect of RBCTs alone or in combination with FFP on oncological outcomes in a cohort of 885 patients who underwent radical cystectomy. While the risk of dying from any cause was similar between patients who received RBCTs alone or in combination with FFP, the odds of dying from cancer were higher in those receiving the combination therapy. The findings in patients with renal cancers are mixed, although it appears that RBCT had a negative impact in prostate cancer patients and those with adrenocortical carcinomas. ,
There is no definitive evidence of the impact of RBCT in cancer recurrence or survival in patients undergoing radical hysterectomy for cervical or ovarian cancer. Although De Oliveira et al. found an increased risk of recurrence in patients with advanced ovarian cancer, subsequent investigations by Altman et al., Warner et al., and Manning-Geist et al. showed no association. More recently, Hunsicker et al. also reviewed the impact of allogeneic blood transfusions in 529 women with stage I–IV ovarian cancer. After adequate propensity score matching, the authors did not find any association between blood transfusion and shorter progression-free and OS.
Consequently, evidence of a deleterious effect of perioperative blood transfusion on cancer progression remains controversial for most malignancies. Although the association with RBCT and poor oncological outcomes has been demonstrated for colorectal cancer, there is low level of evidence to recommend or contraindicate perioperative RBCT in other malignancies. Nevertheless, the presence of anemia and the requirement for RBCT are associated with more adverse surgical outcomes in patients with cancer.
Thrombosis
Hemostatic Dysfunction and Cancer
Patients with cancer are at high risk of both thromboembolic and hemorrhagic events during the course of their disease. The interplay between cancer and the hemostatic system is well recognized, but complex, and involves a diverse range of factors and pathophysiological mechanisms related to the cancer itself, patient factors, and “anticancer therapies,” including surgical stressors. There is a wide spectrum of clinical manifestations from macrovascular complications such as pulmonary embolus (PE), deep vein thrombosis (DVT), and arterial thrombotic events, through to microvascular pathology such as disseminated intravascular coagulopathy and thrombotic thrombocytopenic purpura. All of these are relevant throughout the perioperative period and confer significant clinical consequences for patients and their surgical outcomes.
The hemostatic system is pivotal to maintain vascular integrity and the surrounding tissue microenvironment during surgery, and incorporates the most fundamental immediate responses to vascular injury and termination of blood loss through to the complex maintenance of the vascular microenvironment and biological continuum of cell–cell interaction, inflammatory responses, stromal cell recruitment, and cellular repair.
The key physiological events required during surgery—when the vasculature and tissue microenvironment are breached—include:
- •
vasoconstriction of blood vessels,
- •
platelet aggregation and adhesion,
- •
generation of the thrombin burst to accelerate conversion of soluble fibrinogen to fibrin polymers to stabilize the platelet plug to the endothelium,
- •
activation of protease inhibitors to contain coagulation and clot formation only to sites of damage, and
- •
activation of fibrinolysis to remove excess blood clot once the structural integrity of the vasculature has been restored.
Many of these processes are perpetually disturbed in patients with cancer and during anticancer therapy, even prior to the event of major cancer surgery. , Importantly, the coagulation system appears to be a systematic target of oncogenic dysregulation and cellular signaling, as a consequence of both the tumor cells and the applied therapies. , Moreover, the surgical stress as a systemic response involving cytokine release, ischemia reperfusion, sympathetic activation, inflammatory response, and hemodynamic changes may further exaggerate hemostatic dysregulation, as well as tumor growth and metastases. Efforts to understand this pathophysiology may have the capacity to prevent important TE and bleeding events, but also impact perisurgical “wound” restoration, patient recovery, and overall cancer outcomes. ,
Importance of Prevention of Thromboembolism in Patients Undergoing Cancer Surgery
The hemostatic system is particularly challenged during the perioperative period, with potential for adverse clinical and economic consequences. Postoperative TE among patients with cancer accounts for more than a third of TE-associated deaths. Nonfatal events remain common (up to 40% in some cohorts) with attributable morbidity, impaired quality of life, increased length of hospital stay, increased rates of recurrent events (2-3 fold), increased rates of bleeding complications on anticoagulant therapy, and negative predictors of OS. Importantly, an index TE event represents a significant clinical hurdle for patients following cancer surgery, not only related to the morbidity and mortality of the TE and the requirement for high-dose anticoagulant therapy, but also the impact on both the recovery and potential interruption to ongoing requirement for therapy. Moreover, TE rates remain high despite the availability of safe, efficacious, and cost-effective thromboprophylaxis (TP) strategies, and more than a third of the events occur postdischarge (within 30 days postoperatively) when routine TP is not universally applied.
Furthermore, in terms of clinical impact this burden is significantly underestimated, with the focus on clinically apparent macrovascular events such as DVT and PE, overlooking the hemostatic and endothelial dysfunction at a microvascular, surgical bed, and tumor biology level, which contributes to morbidity, postsurgical recovery, cancer progression, metastatic potential, and mortality. This interplay between hemostatic dysfunction and tumor cell survival, proliferation, and metastatic spread, as well as the antiangiogenic and antitumor properties of anticoagulants, , highlights additional opportunity for therapeutic impact. The key is identifying for whom and when this impact is maximal.
Risk-Directed Approach to Prevention Thromboembolism Associated With Cancer Surgery
While all patients with cancer, particularly in the perioperative period, should be considered at risk of TE, the risk is heterogeneous and dynamic, with the absolute magnitude and duration not equal for all patient cohorts, or within cohorts. The net risk varies according to patient factors (e.g., age, body mass index, comorbid disease), cancer type and stage, and treatment-related factors (e.g., chemoradiotherapy, biological therapy, hospitalization, presence of vascular access devices). This risk heterogeneity—for both TE and bleeding—is exaggerated during the course of the disease and different intervention phases, most notably the perioperative period, emphasizing the importance of some degree of risk stratification, rather than broad application of preventative strategies.
In retrospective and prospective studies, the TE risk for patients with cancer undergoing surgery increases over the age of 40 years, with a 2–3-fold increased risk for those aged 60 years or older. Moreover, irrespective of cancer, increasing age is associated with other risk factors, such as comorbidities, decreased exercise and functional status, and greater immobility, which all play a role in the TE risk postprocedurally. ,
Cancer-related factors are important, including histological subtype, grade, site, stage, and therapy. The highest TE rates occur in mucin-producing adenocarcinomas and the primary site tumors of the pancreas, gastrointestinal system, lung, brain, uterus, and kidney. However, the frequency of cancer and surgical requirements equally dictate this landscape, with TE-related complications reported in breast, gynecological, and lung cancers in women and prostate, colorectal, and lung cancers in men. Patients with more advanced stage are at substantially greater TE risk with both regional and distant metastases compared with local disease undergoing resection. The highest risk period is immediately following the diagnosis or at relapse, during the first 3–6 months, and particularly during chemotherapy/chemoradiotherapy, which is a common strategy prior to surgical resection. The awareness of this escalated TE risk prior to surgical resection is imperative, given that surgery alone confers a 2–3-fold TE risk compared with similar procedures in patients without cancer. ,
Efforts to apply risk stratification have focused largely on primary tumor site, stage, and comorbid disease, with some consideration to anesthetic and surgical techniques. Such factors lack potency, and real-time clinical application of risk stratification strategies has been challenging. Nevertheless, a systematic approach via prehabilitation programs allows preemptive assessment and identification of TE risk, with integration and the ultimate decision regarding application of pharmacologic prophylaxis (P-TP) in conjunction with neuroaxial blockade, real-time intraoperative assessment of hemostasis, and bleeding risk.
Application of Prophylaxis in Cancer Surgery
P-TP is a proven preventative and safe strategy, with up to 80% reduction in TE in cancer cohorts. However, the heterogeneity in TE risk and concerns regarding cumulative bleeding risk has restricted “routine” P-TP utilization and caused uncertainty regarding real-time clinical application. Meta-analysis demonstrated a 14-fold variation in surgical TE risk in a broad population of patients who did not receive postoperative P-TP using the Caprini risk assessment model. Moreover, Pannucci et al. demonstrated that although P-TP significantly reduced postoperative TE (odds ratio [OR], 0.66; 95% confidence interval [CI], 0.52–0.85; P = 0.001), this was associated with an increased incidence of clinically significant bleeding (OR, 1.69; 95% CI, 1.16–2.45; P = 0.006). Importantly the maximal benefit of P-TP was identified in patients determined to be at high surgical TE risk (Caprini score ≥7), without an increased risk of bleeding.
All major expert clinical guidelines highlight the clinical and economic importance of postoperative TE and as a consequence are largely explicit that all patients undergoing major surgical intervention should be offered P-TP (generally recommending low-molecular-weight heparin), unless contraindicated. It should either commence preoperation or 6 hours postoperation and continue for 7–10 days, with consideration of extension (28 days) for major abdominopelvic surgery. Mechanical methods for TP can be added to, but not replace, P-TP as a single strategy except if P-TP is contraindicated, with the focus being on active or high risk of bleeding. A combined strategy may be considered in high TE risk patients, targeting the potential for improved efficacy.
Despite consensus on the effectiveness of primary TE prevention strategies and presence of international clinical practice guidelines, surgical (and medical) patients with identifiable risk factors continue to be admitted to acute care hospitals without receiving appropriate TP. , The reasons are multifactorial, but largely reflect poor (or highly variable) implementation into clinical practice due to the complexity and relevance of the feasibility of real-time risk assessment of patients and the decision-making provision of appropriate prophylaxis in accordance with that assessment. , , Guidelines often do not allow for flexibility and adaptability to acknowledge the patient and procedural factors that alter risk or the real-time nuances of surgical clinical interaction. Equally there is a perception that TE is less problematic due to improved surgical and anesthetic techniques and earlier discharge. It is therefore not surprising that reported rates of compliance with TE preventative guidelines are as low as 6% at baseline and 36% postintervention. This suggests that the availability of evidence-based recommendations is insufficient to ensure consistent adoption.
Appropriate, but simple and practical, TE risk stratification is essential to guide appropriate TP for maximal clinical benefit. This precision medicine approach, while widely advocated, has found little practical application in TE prevention, and to date there have been few prospective studies supporting its use. Decision-support tools that allow real-time surgical application incorporating competing thrombotic and bleeding risks are increasingly being explored. One such application, the Surgical Thromboembolism Prevention protocol (STEP), is a smartphone application that uses a risk assessment model that considers both procedural and patient risk to generate an overall TE risk. It also integrates surgical hemostasis at the time of surgical “time out” to generate a decision-making algorithm for the appropriate application of TP strategies, including application of both P-TP (choice of agent, time to commence, duration, dose, cautions, and contraindications) and M-TP (strategy, duration, and cautions). Implementation of this protocol has demonstrated sustained, routine TE prevention postsurgery (99% adherence across 24,953 surgical admissions) with a substantial reduction in both TE (relative risk reduction [RRR], 79%; 95% CI, 39%–93%; P = 0.02) and bleeding events (RRR, 37%; 95% CI, 6.2%–57.5%; P = 0.02—which included major bleeding RRR of 31% and nonmajor bleeding RRR of 50%). This program was led by anesthetic perioperative physicians at Peter MacCallum Cancer Centre and is scalable across health institutions and all cancer surgery. This strategy has the capacity to overcome the most important lingering challenge in this clinical area: appropriate, real-time utilization of TP strategies among all patients with cancer undergoing surgical interventions.
Conclusion
The clinical and economic value of systematic identification and management of anemia and the appropriate use (even avoidance) of blood products and TE risk in all patients undergoing surgery for cancer are profound. Despite advances in surgical and anesthetic techniques, these pathologies remain major, potentially preventable, contributors to morbidity and mortality postsurgery. Consequently, routine patient blood management and TP are rightly considered to be the standard of care for the cancer patient.
Among patients with cancer, both anemia and the delivery of blood products in the perioperative period are associated with reduced postoperative survival. Anemia remains the most common hematological abnormality among cancer populations, with prevalence and severity varying by cancer type, stage, and therapy. Retrospective and prospective studies have reported anemia in 30%–90% of oncological patients at diagnosis. Importantly, anemia is a consequence of potentially avoidable and/or reversible drivers. , Early identification can allow optimization and reduction in, or avoidance of, unnecessary allogeneic transfusion in the perioperative period.
Hemostatic dysfunction, in particular the hypercoagulable state, with macro- and microvascular events, remains an important phenomenon, further exaggerated in the postoperative setting. Simplistically, TE is a common and preventable complication of major cancer surgery, with negative implications for survival. Predictive models and decision-making algorithms can identify high TE risk patients, drive systematic preventative strategies, and positively impact postsurgical recovery, morbidity, and mortality among patients with cancer.
References
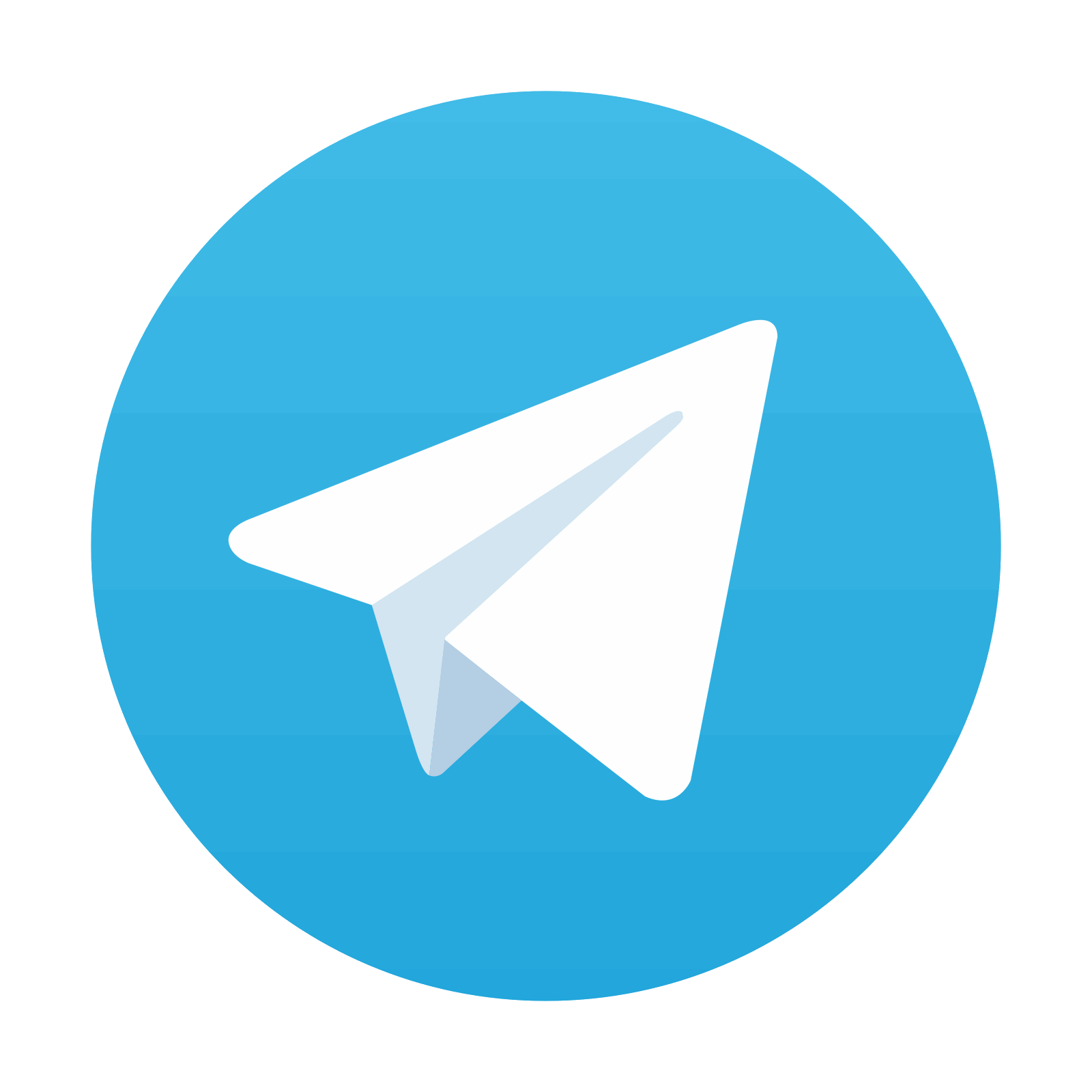
Stay updated, free articles. Join our Telegram channel

Full access? Get Clinical Tree
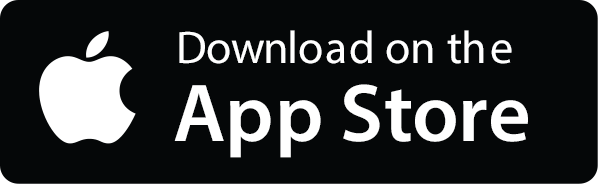
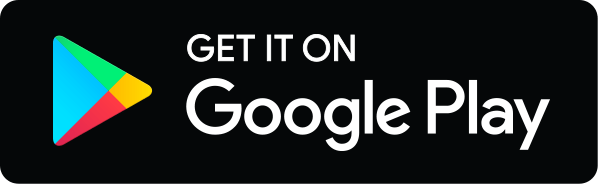
