B. Sitting or semi-sitting craniotomy is the classic procedure associated with VAE. This is the result of having not only an elevated position of surgery, but also due to the fact that there are noncompressible structures such as dural sinuses that may enhance air entrainment. Jadik et al. [3], have described three potential pressure situations which may affect the likelihood of development of VAE during semi-sitting craniotomy:
1. Low risk. Pressure proximal and distal to the injured vessel is under constant pressure and bleeding site is relatively easy to find.
2. Intermediate risk. Pressure is constantly negative, bleeding of injured vessel and source of entrainment are absent or difficult to find (moderate jugular compression may assist in identification).
3. High risk. Positive pressure in the injured sinus of vein during the inspiration of mechanical ventilation and negative pressure during expiration leads to large entrainment.
C. During particular procedures such as kyphoplasty/vertebroplasty, the prolonged uncapping of the needle can lead to entrainment which is similarly seen in central-line insertions [4]. It is important to note that the standard prone position of the procedure results in a position higher than the heart, and may lead to venous pooling and a decrease in venous pressure within vertebral elements. This may ultimately increase the risk of air entrainment.
D. There are some smaller risk procedures such as cesarean section, hypophysectomy, and upright shoulder surgery, which have reportedly been associated with VAE [5].
CLINICAL PEARL
The major risk factor associated with VAE is the position of the surgical/procedural site in relation to the heart. Though the sitting or semi-sitting craniotomy is the classic procedure associated with VAE, a VAE can occur in any procedure where venous disruption is above the level of the heart.
III. Incidence
A. The overall incidence of VAE is unclear as a result of a variety of detection techniques that make the observation of emboli relatively easy. These specific techniques will be discussed later in this chapter, but clinically significant VAE events appear to be a relatively rare event.
B. O’Quin and Lakshminarayan [6] reported an observed rate for VAE in the operative setting to be approximately 21% to 40% in sitting neurosurgical or otolaryngologic procedures (namely, neck dissections) with a subsequent study revealing an overall incidence of 25% to 60% in procedures where the surgical site was higher than the level of the heart [7]. A recent investigation by Jadik et al. [3] revealed a rate of 50% in semi-sitting position craniotomies.
C. Syymons et al. [8] also reported a rate which was more widespread, from a low of 1.6% to a high of 93% with subsequent mortality rates of 0% to 73%.
D. It appears that the sensitivity of the detection device is the key feature in identifying the presence or absence of venous air.
CLINICAL PEARL
The overall incidence of VAE is unclear as the sensitivity of the detection device is the key feature in identifying the presence or absence of venous air. While clinically significant VAEs are rare, they can be associated with significant hemodynamic changes and potential morbidity.
IV. Grading scale
A. There are numerous ways in which VAEs are classified, but most are a variation of one another, many based on the initial work of Matjasko et al. [9], whose team described four major grades based on changes in precordial Doppler sounds, changes in end-tidal carbon dioxide (EtCO2), ability to aspirate air, and hemodynamic instability (Table 22.2). Perhaps the most extensive description scale comes from Girard et al. [10] that incorporates detection devices such as precordial Doppler, EtCO2, and blood pressure measurements (Table 22.3).
Table 22.2 Four grades of intensity of venous air embolus (VAE)
Table 22.3 Girard scale for venous air embolism
B. The utilization of transesophageal echocardiography (TEE) has also increased the degree of identification of venous air, thus increasing the reported incidence rate. However, clinically significant VAE remains low [11] (Table 22.4).
Table 22.4 Scale for venous air embolism utilizing TEE
V. Dose required for significant events
A. It is important to note that there is a dose requirement associated with VAE to create a hemodynamically significant event. Determination of this number has been under constant investigation with many initial studies utilizing dog or swine models to determine lethal doses (LDs). Early investigations revealed that the LDs associated with air was 40 to 50 mL in a small dog and 100 to 200 mL in a larger animal. Early determinations noted that the LD amount required was 7.5 mL/kg with a human range of 200 to 300 mL [12], while others determined the mean cumulative dose for VAE associated with circulatory collapse in the swine model was 4.5 mL/kg [13].
B. One of the very few human case reports has come from Toung et al. [12], whose team described a patient with a history of heart failure who underwent pneumopericardiography in which 200 mL of air was injected over 3 to 5 seconds. This led to restlessness, apnea, ST depression on electrocardiography (ECG), and a varying degree of atrioventricular (AV) block. Overall, the consensus is that the adult lethal dose is approximately 200 to 300 mL or 3 to 5 mL/kg.
1
C. It is also important to note that it is not just the volume involved, but also the speed of injection that plays a vital role. It has been mentioned that the LD of 200 to 300 mL in humans can be exceeded if the air is entrained slowly, allowing for hemodynamic compensation [8,14].
D. The more rapid speed of injection of air results in a lower dose of air required for a lethal event. A bolus of air tends to lead to an increase in central venous pressure (CVP), a decrease in pulmonary artery pressure (PAP), ST segment depression, and shock that is thought to be related to an air lock in the right ventricle. On the contrary, a slow infusion of air results in an increase in CVP/PAP and with a decrease in systemic vascular resistance (SVR) with a compensatory increase in cardiac output [15].
E. As the volume of entrainment increases, a variety of clinical effects can be seen. These effects vary from changes in oxygen saturation, decrease in EtCO2, electrocardiographic effects, and cardiovascular collapse (Table 22.5).
Table 22.5 Entrainment dose of air embolism and clinical effects
Table 22.6 Complications associated with VAE
CLINICAL PEARL
The volume associated with VAE to create a hemodynamically significant event is related to both the volume and rate of air entrainment. However, the threshold of a lethal adult dose is in the range of 200 to 300 mL or 3 to 5 mL/kg.
VI. Complications associated with VAE
A. Hypoxia/sympathetic reflex vasoconstriction. The development of hypoxia and increase in pulmonary vascular resistance (PVR) are some of the key complications associated with VAE. Essentially, the air embolus lodges into the pulmonary vasculature and results in a rapid increase in sympathetic reflex vasoconstriction leading to ventilation–perfusion abnormalities with increase in the physiologic dead space. These events result in decreased EtCO2, increased partial pressure of carbon dioxide (PaCO2), and hypoxemia [16,17].
B. Role of microbubbles and humoral/neurogenic factors. A significant number of effects of VAE are a result of the interaction of microvascular air bubbles with elements within the circulation and are known to activate the release of endothelial mediators that result in an excessive complement production, cytokine release, and production of reactive oxygen species (ROS) [18]. Microbubbles also lead to an obstruction of blood flow with an increase in PAP as well as a resultant trigger in platelet and neutrophil activation. The neutrophils can also release superoxide anions leading to pulmonary microvascular damage [16].
1. Humoral mediators. A thorough review of the subject has revealed that multiple mediators play a role in the induction of damage associated with the VAE. These include serotonin (which results in intense vasoconstrictor of pulmonary vasculature), adenosine diphosphate (ADP), thrombin, catecholamines, leukotrienes, bacterial toxins, cytokines, ROS, angiotension II (AT-II), endothelin-1 (ET-1), platelet-derived growth factor (PDGF), platelet activating factor (PAF) [19]. Ultimately these mediators also lead to a reduction in lung compliance due to increased permeability, interstitial edema, and airway resistance.
2. Neurogenic mediators. It is also known that embolism will trigger a serotonin-induced von Bezold–Jarisch reflex possibly via J-receptors, pulmonary irritant receptors, and pulmonary c-fibers. This stimulation results in the apnea, bradycardia, and hypotension routinely seen with massive embolism [19].
C. Right ventricle failure/air lock. In the case of massive VAE the generation of an air lock may occur. This air lock is essentially a complete outflow obstruction leading to no forward blood flow, increased wall tension in the right ventricle (RV), increased myocardial oxygen consumption of the RV, RV ischemia, and ultimately cardiovascular collapse. More modest volumes may still result in significant right ventricular outflow obstruction [2] that can also trigger the humoral agents’ release of inflammatory mediators, bronchoconstriction, increase in ventilation/perfusion mismatch, and reflex vasoconstriction previously mentioned [20].
D. Thrombocytopenia
1. The mechanism for VAE-induced thrombocytopenia is unknown, but may be related to the direct binding and activation of platelets when they encounter microbubbles. This activation will lead to a formation of air–platelet conglomerates that ultimately liberate more mediators leading to further platelet aggregation [21]. It has been suggested that the formation of platelet–air conglomerates adversely affects air reabsorption, and may lead to a prolonged increase in right ventricular afterload and aggravation of the cardiopulmonary complications associated with VAE [22].
2. Platelet activation results in the release of humoral factors (both procoagulant and vasoconstrictor in nature) that contribute to the pathophysiology of VAE. The release of thromboxane-A2 leads to pulmonary vasoconstriction and a proaggregatory effect on platelets that results in even further release of thromboxane-A2, as well as serotonin, a substance that also has pulmonary vasoconstrictive properties [19,23].
E. Cerebral air (cerebral edema and capillary leak as well as ischemia). Another catastrophic complication associated with VAE is the entrainment of venous air into the arterial circulation with a resultant embolic event, notably cerebral in nature [24]. Notably, this situation occurs when air transverses a patent foramen ovale (PFO) which is a defect that can be found in approximately 25% of the adult population [25]. Cerebral air embolism results in local ischemia and cerebral edema that results in a capillary leak syndrome induced by the ischemic event. Manipulation of central venous catheters, utilization of extracorporeal circulation, arteriography, and hemodialysis are the procedures most at risk. Though there is no conclusive consensus regarding patients with pre-existing PFOs and subsequent neurosurgical procedures, an extensive review by Fathi et al. [26] suggests that screening for the presence of PFO may be warranted. The presence of the structural anomaly would potentially alter the neurosurgical approach to the procedure (i.e., changing from sitting to horizontal) or allow for a surgical repair of the defect prior to the definitive procedure.
CLINICAL PEARL
There are numerous physiologic responses associated with a VAE. An air embolus lodges into the pulmonary vasculature and results in a rapid increase in sympathetic reflex vasoconstriction leading to ventilation–perfusion abnormalities. If a larger air embolus occurs, an air lock in the right ventricle can cause a complete outflow obstruction leading to cardiovascular collapse.
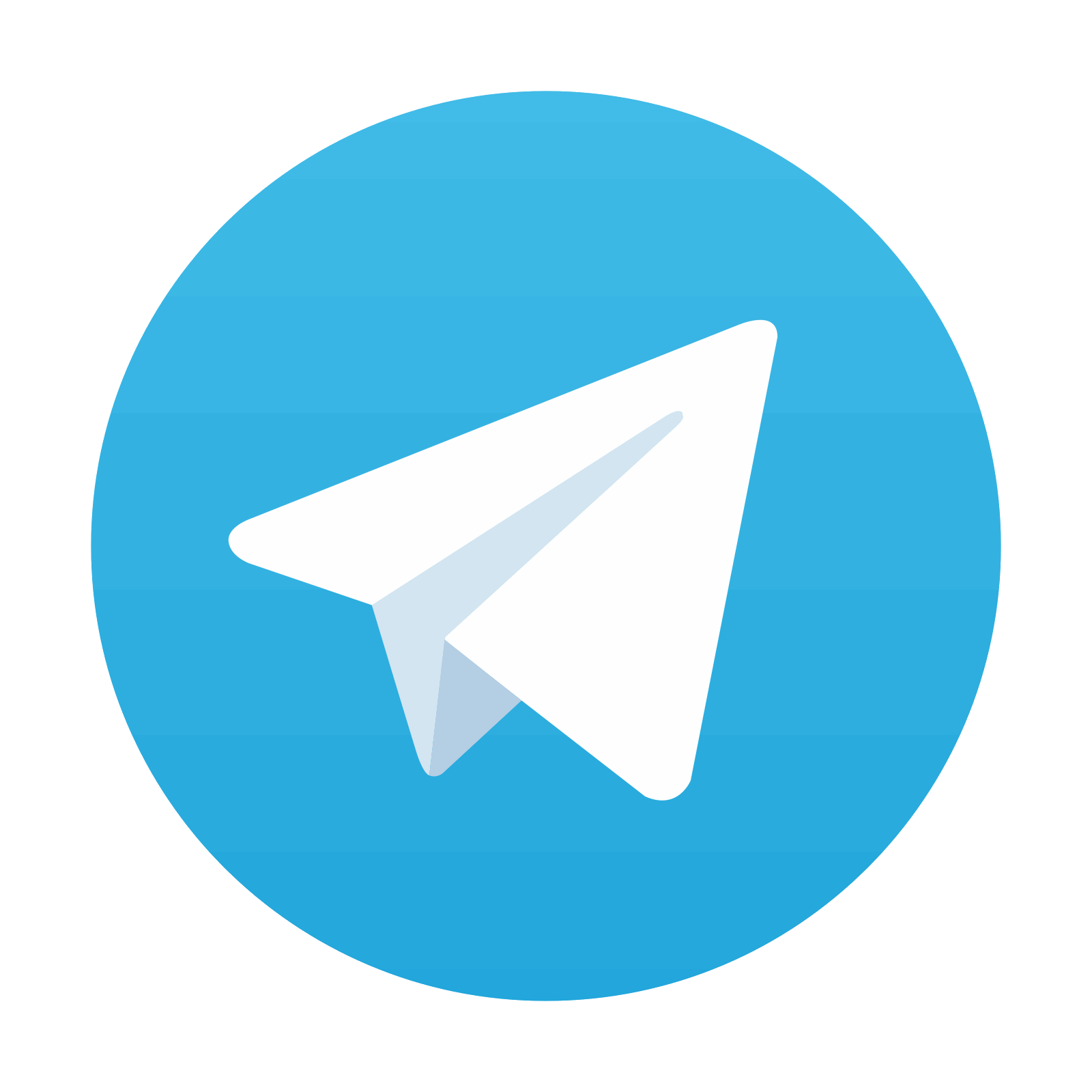
Stay updated, free articles. Join our Telegram channel

Full access? Get Clinical Tree
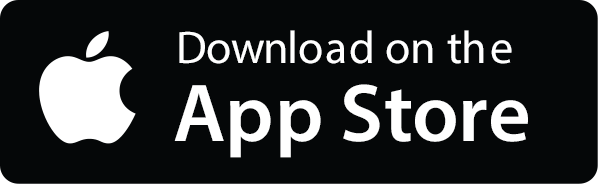
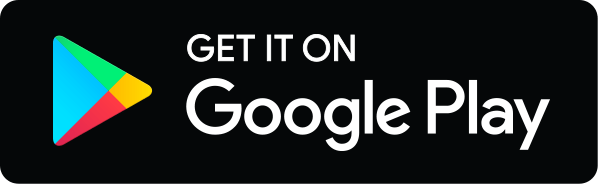