c. The basic frequency bands are thought to be rhythmic activity resulting from neural connections with the brainstem and thalamus (sometimes referred to as EEG pacemakers).
d. Simple methods of computer processing include the average frequency and the average power (voltage squared) contained in the entire EEG or in each of the frequency bands.
e. EEG spike activity and burst suppression are important features of the raw EEG.
(1) EEG “spikes” or “sharp waves” occur when massive synchronized activity occurs with a seizure. Repetitive spikes are used in the diagnosis of epilepsy.
(2) Burst suppression (intermittent electrical activity interspersed with periods of little or no electrical activity) indicates a reduction in synaptic activity associated with reduced cerebral metabolic activity. This can occur from several mechanisms (e.g., trauma, drugs, hypothermia, etc.). The Burst Suppression Ratio (BSR) is used to characterize this and is the fraction of time where the EEG is suppressed.
2. Frequency domain. Since the frequency content of the EEG changes with anesthesia and various brain states, computer processing methods to better quantify the EEG frequency content can be used.
a. One of the most frequently used methods is a mathematical technique called Fourier series analysis developed by Baron Jean Baptiste Joseph Fourier. In this method, the EEG is assumed to be composed of a large number of individual waves of specific frequencies much like light is composed of a mix of different colors. The results of this analysis is termed frequency domain and allows a plot of power at each frequency.
(1) There are two types of common frequency domain displays. The Compressed Spectral Array (CSA) presents the plot of power versus frequency with plots stacked in a third dimension showing changes over time. The Density Spectral Array (DSA) is similar except the amplitude of the power is shown in color or gray scale.
(2) In the frequency domain a commonly derived parameter is the spectral edge frequency (SEF) (above which there is very little power; e.g., SEF95 is the frequency where 95% of the power is below).
E. Other mathematical processing techniques. A variety of other mathematical processing techniques have been employed. These include analyzing the relationship of different frequency components within the EEG with each other. [2] The bispectrum measures the correlation of phase between different frequency components. This analysis may describe the synchrony of different generators of the EEG. The degree of asynchrony or variability in the EEG has also been quantitated.
F. Anesthesia effects
2
1. EEG abnormalities appear rapidly when cerebral blood flow is reduced making the EEG useful to detect cerebral ischemia; generally an asymmetric drop in amplitude or reduction in frequency occurs with ischemia.
a. The EEG becomes abnormal below 22 cc/min/100 g and absent below 15 cc/min/100 g (ischemic threshold). The EEG usually fails in 20 seconds following complete cessation of blood flow. This ischemia can eventually lead to cellular death; cell death is also a function of the duration of hypoperfusion. For example, cell death may take 3 to 4 hours when the cerebral blood flow is just below the blood flow where the EEG becomes abnormal. Infarction occurs after progressively shorter periods of time as the blood flow is reduced below this level.
b. Regions that have the highest metabolic rate or are farthest from the major supply arteries (i.e., boundary regions) are most sensitive to hypoperfusion, making them among the best electrode locations to detect regional ischemia.
c. EEG monitoring is used during surgery where there is a risk of stroke due to reduced blood flow such as during intracranial and extracranial vascular surgery (e.g., carotid endarterectomy, [CEA], intracranial aneurysm surgery).
d. With vascular surgery the time to change in the EEG following cross-clamping has also been used to estimate the allowable ischemic time before irreversible neuronal injury occurs or to prompt the consideration for the use of a shunt. Of note with CEA, the EEG only monitors the surface of the brain and may miss ischemic insults deep in the brain. In addition, it may not detect the two-thirds of postoperative strokes in CEA that are thought to result from embolic events.
e. The EEG can also be used during intracranial vascular surgery to determine the tolerance of the cortex for temporary cross-clamping. Rapid EEG loss indicates poor collateral flow and suggests that medical interventions such as induced hypertension should be considered to improve collateral flow.
2. Since approximately 40% to 50% of the resting energy utilization of the neurons is utilized by normal neuronal activity reflected in the EEG, the EEG has been used to monitor intentional metabolic suppression of the brain to improve the supply–demand relationship during the periods of reduced supply.
CLINICAL PEARL
The EEG is a reflection of the 40% to 50% of the metabolic activity in the cortical neurons related to synaptic function.
a. During surgical procedures
(1) The reduction of EEG activity during metabolic suppression by anesthetics has allowed the EEG to be used to insure or guide suppression during procedures where cerebral blood flow may be compromised (e.g., repair of an aortic arch, CEA, intracranial vascular surgery).
(2) This technique is used to monitor metabolic suppression in the intensive care unit (e.g., “barbiturate coma”).
(3) The EEG can also be used with hypothermia, which can reduce the entire cellular metabolism.
(4) Burst suppression is used as the endpoint of drug-induced suppression as it represents near-maximal suppression of neuronal activity.
CLINICAL PEARL
Burst suppression shows near-maximal neuronal depression.
(5) The EEG can also detect the increased metabolism associated with seizure activity to prompt treatment which will reduce the excessive metabolic activity.
3. The ability to detect the electrical activity of seizures is a unique aspect of the EEG which is useful during surgery and in the intensive care unit.
a. In the operating room this allows the EEG to be recorded directly from the cortex of the brain (electrocorticography or ECoG) to identify a seizure focus for tissue resection. When the abnormal foci is located near the eloquent cortex (near the speech or motor cortex), an awake craniotomy allows the identification of the cortex critical for speech or motor function so as to preserve these functions while resecting the seizure foci with a reasonable possibility of permanent seizure resolution.
b. An awake craniotomy may not be essential when motor areas are involved, as nowadays motor evoked potential (MEP) monitoring as well as cortical and subcortical mapping represent safe and reliable methods to protect these functions in patients under general anesthesia. Yet, awake craniotomies remain the gold standard when language and other cognitive functions are at risk during surgery because there are no techniques yet that can assess the functional integrity of these functions in an asleep patient.
c. EEG, in the form of ECoG, can also be used to detect evoked seizures (the so-called afterdischarges) during electrical stimulation of the brain when it is used to detect the speech centers by interruption of clinical function during stimulation. It can also detect seizure activity when stimulation of the cortex is used to map the motor cortex (about 25% of cases have induced seizures when a 50- to 60-Hz monopolar stimulator device [Penfield technique] is used for the stimulation).
d. If a clinical seizure is induced intraoperatively by cortical stimulation, before the administration of any antiepileptic or neuroprotective drug that may interfere with the result of mapping, it is advisable to irrigate the cortex with cold (4°C) Ringer’s solution. This suffices, most of the time, to arrest the seizure without compromising further neurophysiologic testing; if needed, a small dose of propofol will terminate the seizure.
e. EEG is useful for seizure detection in the intensive care unit, particularly when patients are pharmacologically paralyzed such that the motor component is not observable. It will also detect seizures that are not associated with motor activity (this occurs in 4% to 14% of traumatic brain-injured patients).
f. In ICU patients, EEG monitoring has also been used to determine brain death (cessation of EEG activity in the absence of hypothermia or medications known to depress the EEG).
4
4. Anesthesia effects on the EEG appear to be mediated by drug effects on synaptic function. Differences between anesthetic agents relate to the spectrum of synaptic receptors altered and the effect on a multitude of receptor subtypes throughout the nervous system. [3,4]
a. A general pattern of drug effects is common among many anesthetics; however, not all drugs follow the same pattern.
b. The general changes in the EEG when anesthetics are added to the awake state include the following: (1) An initial excitatory stage with increased power in the faster alpha and beta frequencies that is prominent in the frontal regions, (2) prominent activity in the alpha frequencies which develops in the more posterior regions, (3) the faster frontal region activity moves posterior while the posterior alpha activity moves forward, (4) a reduction in the variability across the brain with a marked increase in the global cortical synchrony and an uncoupling or disruption of the interaction between right and left frontal and parietal regions, (5) a general reduction in high-frequency activity with increases in slower frequencies (theta and delta), (6) a general reduction in EEG amplitude after an initial increase in amplitude, and (7) some agents will stop all activity at higher doses (metabolic suppression).
CLINICAL PEARL
A decrease in EEG amplitude and frequency usually occurs with deeper anesthesia.
(1) Currently used volatile anesthetic agents (sevoflurane, desflurane, and isoflurane) cause a progressive and profound depression of the EEG at higher doses. At deep surgical anesthetic concentrations, burst suppression occurs at approximately 1.5 times the minimal alveolar concentration (MAC). Higher concentrations produce electrocerebral silence.
(2) Intravenous anesthetic drugs (propofol, barbiturates, etomidate, benzodiazepines) at higher doses produce the typical EEG anesthetic depression pattern described above.
(3) Nitrous oxide, as well as the intravenous agent ketamine, can produce EEG excitation with the increase of higher frequencies. Ketamine has been reported to provoke seizure activity in patients with epilepsy but not in normal subjects.
(4) The opioids produce a dose-related decrease in frequency of the EEG until it is in the delta range while maintaining amplitude. Since the opioids do not produce marked suppression of the EEG, they are frequently used during ECoG in surgery for seizure focus identification and ablation.
(5) Dexmedetomidine does not produce burst suppression and produces an EEG which is very similar to slow-wave sleep.
(6) Some anesthetics can produce both depression and enhancement of synaptic activity. With excitement, the EEG has increased amplitude and frequency that can become epileptiform. In small doses these agents (methohexital and etomidate) can be used to enhance epileptic foci during ECoG to assist in identifying native seizure foci. Only enflurane and sevoflurane of the volatile anesthetics can, in high concentrations with hyperventilation, cause epileptiform spikes or electrographic seizures.
CLINICAL PEARL
Methohexital and etomidate can be used to enhance recording of a native seizure focus.
c. Monitoring anesthetic agent effect can be done by visual inspection of the EEG as well as using a variety of processed EEG devices. In each case, mathematical tools are used to derive a parameter associated with the degree of anesthetic drug effect.
III. Electromyography is the recording of the electrical activity of muscles and has been known since Francesco Redi’s observations in 1666. Clinical diagnostic uses evolved between the 1920s and 1950s with its major intraoperative use starting in the 1960s to preserve facial nerve function during brainstem surgery for acoustic neuroma (vestibular schwannoma). Additional interest emerged to assess nerve roots during spinal surgery in the 1990s [5,6].
A. Overview. Similar to the EEG, the EMG is the observation of spontaneous activity. When a person is awake, the muscle activity of the EMG has characteristic activity; under anesthesia the EMG is normally quiet allowing it to show abnormal activity in the muscles or the nerves which innervate the muscle being monitored (termed “free run” monitoring). In addition, the EMG can be used for the detection of stimulation of the motor nerves (intentional or unintentional).
CLINICAL PEARL
The EMG is normally quiet (inactive) under anesthesia.
B. Recording techniques. EMG recording can be recorded from muscles innervated by both peripheral and cranial nerves
1. Cranial nerve monitoring is of particular interest since they are susceptible to damage intraoperatively due to their small size, limited epineurium, and complicated course. They also may be intertwined in tumors placing them at risk during tumor resection. Damage occurs from either trauma (surgical disruption, manipulation) or ischemia, and this leads to paresis or paralysis (with subsequent disability or deformity) and possibly pain [7].
2. Monitoring can be accomplished from all cranial nerves which have motor components. The most frequently monitored cranial nerve is the facial nerve which is commonly monitored using the orbicularis oculi and orbicularis oris muscles ipsilateral to the side of surgery (Table 26.2).
Table 26.2 Muscles for cranial nerve monitoring
3. EMG techniques have been used with peripheral nerves, most notably to assess nerve roots with spinal corrective surgery (Table 26.3) [8].
Table 26.3 Muscles typically monitored for peripheral nerve monitoring
C. Responses
1. The EMG is recorded by electrode pairs placed in (especially for diagnostic applications) or near (typically for monitoring) the muscles of interest. The difference in electrical activity between the two electrodes is displayed similar to EEG techniques. The activity can also be played in a loudspeaker to give audible feedback.
2. Three types of abnormal spontaneous activities are usually seen during monitoring.
a. Continuous low-amplitude activity seen in multiple muscles is often due to light anesthesia. The audible sound is often continuous noise.
b. Neurotonic bursts are high-frequency intermittent or continuous bursts from nerve irritation such as mechanical stimulation (e.g., nearby dissection, ultrasonic aspiration, or drilling), nerve retraction, thermal irritation (e.g., heating from irrigation, lasers, drilling, or electrocautery), and chemical or metabolic insults. Bursts usually last less than 200 msec with single or multiple motor unit potentials firing at 30 to 100 Hz. The audible sound is often a short “blurp.” Typically, these bursts last only for a short time after the mechanical stimulation of the nerve has stopped, and usually are not of concern unless they are of high amplitude or sustained.
5
c. Long neurotonic trains of activity may be indicative of neural injury and are causes for concern and alarm. They are commonly associated with impending nerve injury (nerve compression, contusion, sustained traction, stretching, or ischemia of the nerve); the more sustained the activity, the greater the likelihood of nerve damage. Their audible sounds have a more musical quality and have been likened to the sound of an outboard motor boat engine, swarming bees, popping corn (“popcorn”), or an aircraft engine (“bomber”) potentials.
d. Recently, a specific pattern of neurotonic discharges, called A train, have been identified in the spontaneous activity of the facial nerve during surgery for vestibular schwannomas. Unlike other patterns of EMG activity, these trains and their duration seem to represent a reliable prognostic indicator of postoperative facial nerve palsy [9].
e. Often multiple muscles are monitored so as to identify activity in different branches of a nerve or so that the responses from one nerve and muscle are not confused with the response of a different nerve and a nearby muscle. For example, recordings from the orbicularis oris or orbicularis oculi might show EMG thought to be from stimulation of the facial nerve, but may actually be recordings of activity from the nearby masseter (CN V) or temporalis muscles (CN VII) when nearby cranial nerves are stimulated.
f. Multiple peripheral muscles may be monitored because they are often innervated through multiple nerve roots.
g. Cutting a nerve may not result in an EMG response.
3. EMG as a result of stimulation of a cranial or peripheral nerve can be used to locate the nerve or identify its integrity during surgery (this evoked EMG response is often termed a compound muscle action potential [CMAP]).
a. Searching with high-intensity stimulation can find the general vicinity of a nerve. Lower-intensity stimulation can locate the nerve. In this way, it is possible to identify regions of a tumor where there is no motor component of a cranial nerve present so that large portions of the tumor can be quickly resected with a low risk of causing permanent neurologic injury.
b. Continual stimulation with electrified instruments during surgery can assist a surgeon in operating with warning when they may be encroaching on the nerve.
c. The amplitude of the CMAP response of the facial nerve has been correlated with immediate and long-term muscle function.
d. Overall, the recording of free-running EMG activity remains a valuable and very common method to monitor cranial nerve function. Yet, one of the limitations of this methodology is due to the variety of stimuli that can influence the pattern of discharge. With the exception of facial nerve monitoring in cerebellopontine surgery, the reliability of EMG activity to monitor the function of other cranial motor nerves is still debated due to the existence of false-negative and especially false-positive responses. Paradoxically, a cranial nerve can be cut with no changes in the EMG activity. Vice versa, sustained activity may not necessarily result in postoperative deficits.
e. For these reasons, other techniques to assess the functional integrity of cranial motor nerves have been explored. Corticobulbar techniques are less commonly used methods to continuously assess the facial nerve by stimulation of the brain similar to the MEP technique described below. This allows a continual evoked assessment of the neural pathway rather than intermittent assessment using stimulation mapping techniques during brainstem surgery (see below).
D. Applications of EMG monitoring. Applications of EMG monitoring provide useful information in a large array of surgical situations including posterior fossa, head and neck, spine, and major joint surgery.
1. EMG is frequently utilized during brainstem surgery to monitor cranial nerve integrity.
a. EMG monitoring of the facial nerve is particularly helpful with vestibular schwannoma (acoustic neuroma) where monitoring increases the likelihood that the anatomic integrity of the nerve will be maintained during surgery. In these cases, over 60% of patients with intact nerves at the conclusion of surgery will regain at least partial function several months postoperatively. An NIH consensus panel has established this use as a standard of care [10].
b. Some authors suggest that a very low threshold (<0.05 mA) for stimulation of the facial nerve at the brainstem, after the removal of a vestibular schwannoma, indicates good motor outcome. Yet, this has been recently questioned due to the low negative and positive predictive values of such a test.
c. Monitoring of the lower cranial nerve nuclei (IX to XII) can help prevent complications of dyspnea, severe dysphagia, and aspiration requiring tracheostomy.
d. EMG monitoring is used in surgery with hyperactive cranial nerves and cranial nerve compression syndromes including trigeminal neuralgia (V), hemifacial spasm (VII), and vagoglossalpharyngeal neuralgia (IX, X).
6
2. EMG has been frequently utilized during spinal surgery where it is considered more sensitive for detecting radiculopathy than the somatosensory evoked potential (SSEP); the SSEP pathway traverses multiple nerve roots such that the SSEP cortical responses may be unaffected if only one nerve root is injured.
a. Spontaneous (“free run”) EMG is used to identify unfavorable stretch or impending injury to nerve roots during approaches to the spine (e.g., lateral approach to the lumbar spine which traverses the lumbar plexus).
b. EMG monitoring has been used to reduce the risk of nerve injury secondary to misplaced pedicle screws (as frequent as 15% to 25%). In this case the pilot hole or screw is stimulated and the current necessary to activate the EMG from the nerve root or spinal cord is measured. If the current is low, then the screw may need to be repositioned as it may have broached the pedicle wall and may be too near to the neural elements so as to cause irritation.
c. EMG during surgery on the cauda equina has proven essential to differentiate neural tissue from nonfunctioning tissue structures tethering the spinal cord which would be cut. It is especially useful to identify nerve roots to the anal and urethral sphincters to maintain bowel or bladder control (S2–S4) [9,11].
d. EMG is useful to identify nerve rootlets during dorsal rhizotomy for sacrifice to reduce incapacitating spasticity (often seen with cerebral palsy). Here, EMG recording is used to identify the most hyperactive rootlets by observing the spread of activity to adjacent myotomes when the rootlets are stimulated (usually between L2 and S2). As with surgery in the cauda equina, EMG recording is also the key preserving rootlets to the anal sphincter and urogenital system.
3. EMG Monitoring has been found to be useful in a variety of other surgeries.
a. Recording of vagal nerve EMG activity is typically done using electrodes in the vocalis muscle or with an endotracheal tube with attached electrodes which are able to make contact with the vocal cords. This is used in surgery on the anterior neck (e.g., thyroidectomy, neck dissection, anterior spine surgery) to identify and prevent damage to the recurrent laryngeal nerve.
b. EMG recordings from the facial musculature can also be used during facial surgery (such as surgery on the parotid gland) to reduce the risk of facial nerve injury.
c. EMG responses have been used to detect unfavorable stretch of peripheral nerves in orthopedic joint surgery. For example, hip replacement or hip arthroscopy can cause nerve stretch where the EMG would signal caution.
d. Identification of the motor cortex can be done by stimulation of the cortex and recording EMG of muscles activated by the stimulation. This can be helpful to preserve motor function during resection of nearby cerebral pathology.
E. Anesthesia effects. Anesthesia considerations concern the use of muscle relaxation. If relaxation must be used, the amplitude of the EMG response will be reduced which may make spontaneous activity undetectable. For stimulated activity, CMAP activity can generally be detected when train-of-four (TOF) testing produces only one twitch or with 75% suppression of the single twitch. However, having more than one twitch is desirable, since the amplitude of the CMAP is reduced by muscle relaxants and small CMAPs may be missed. Pedicle screw thresholds may be falsely elevated with greater than 75% blockade.
CLINICAL PEARL
Muscle relaxants should be avoided when electromyographic (EMG) recording is being done.
IV. Somatosensory evoked potentials are a method to assess a normally quiet sensory pathway by stimulating the pathway and recording the evoked response (much like stimulated EMG). Because of the small size of the cortical response to peripheral nerve stimulation, the development of the SSEP required signal averaging and the development of digital computers in the 1970s and 1980s. Applications in IOM became common when Nash and Brown in the 1970s found an application in reducing the risk of paralysis in scoliosis correction.
A. Overview. The SSEP response has wide clinical utility because of the length of its neural pathway and the various neural risks associated with surgery along that pathway.
B. Anatomy. The SSEP is a sensory-evoked response which involves stimulation of a peripheral sensory nerve and recording the response of the sensory cortex.
1. Although any sensory nerve could be used, large, mixed motor and sensory nerves are usually stimulated electrically. The nerves usually utilized for IOM tend to be the median (component roots C6–T1), ulnar (C8–T1), posterior tibial (L4–S2), and occasionally the common peroneal nerve (L4–S1).
2. Peripheral nerve stimulation activates the large diameter, fast conducting Ia muscle afferent and group II cutaneous nerve fibers producing both sensory transmission giving rise to the monitored response and transmission through the motor fibers giving rise to muscle contraction.
FIGURE 26.1 Pathway of the somatosensory evoked potential. The SSEP is produced by stimulation of a peripheral nerve (arrow). The electrical activity enters via the dorsal nerve root and ascends the spinal cord via the dorsal column pathway of proprioception and vibration. It synapses at the cervicomedullary junction and crosses the midline ascending in the medial lemniscus to the ventromedial nucleus of the thalamus where it has a second synapse. From there it ascends to the sensory cerebral cortex for the limb stimulated. SSEP recordings can be made along the pathway; shown are the responses from the peripheral nerve, spinal cord, cervical spine, and cerebral cortex. (Reproduced from Jameson LC, Janik DJ, Sloan TB. Electrophysiologic monitoring in neurosurgery. Anesthesiol Clin. 2007;25:605–630, with permission.) [12]
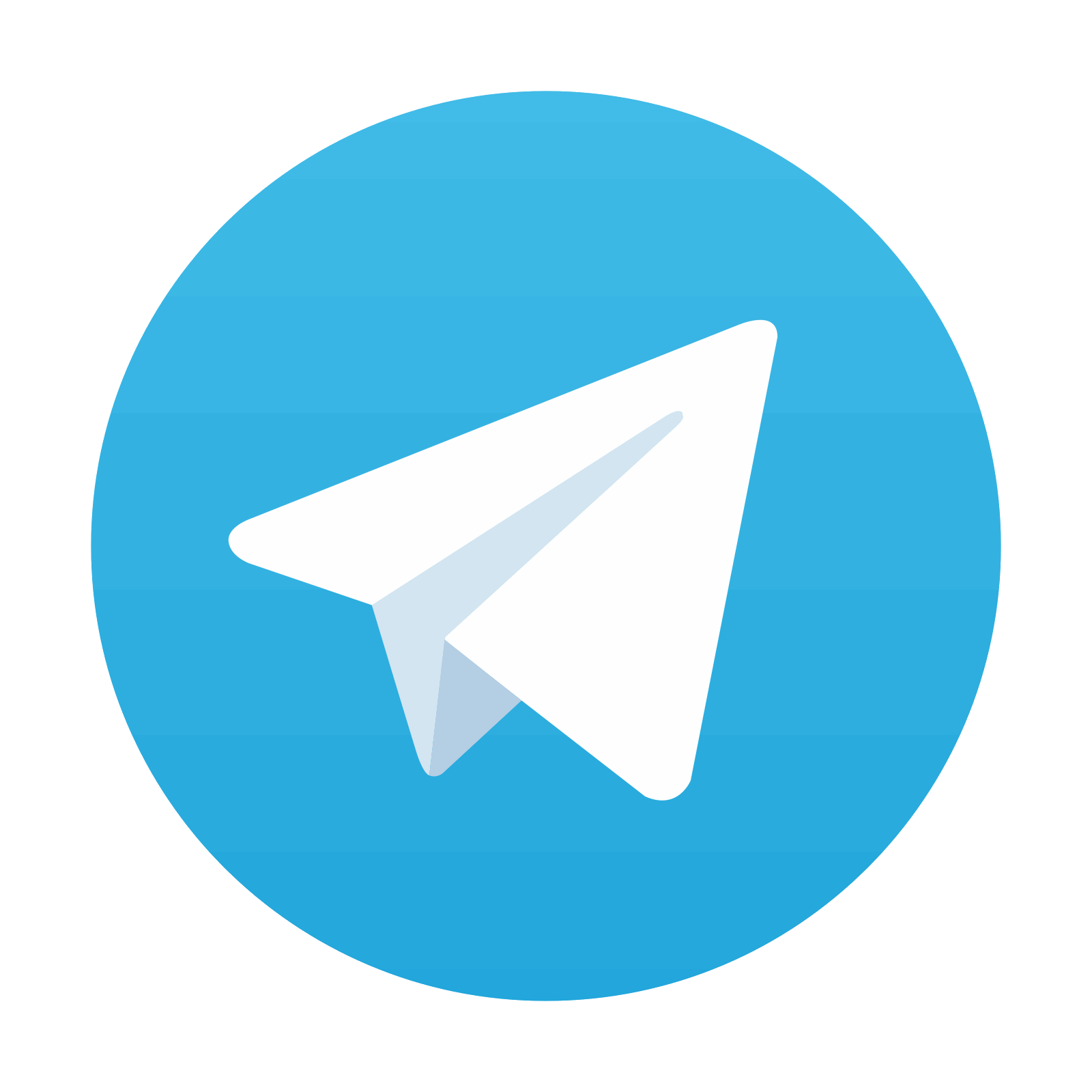
Stay updated, free articles. Join our Telegram channel

Full access? Get Clinical Tree
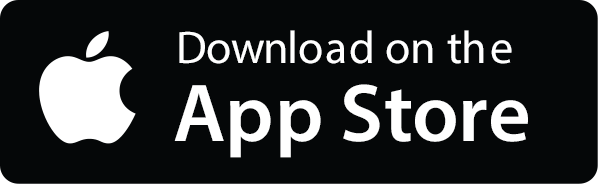
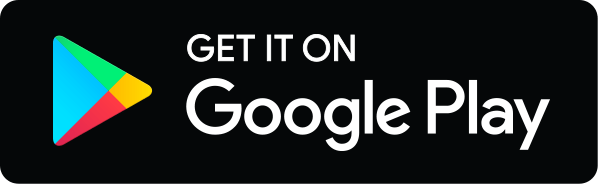