Chapter 73
Acute Lung Injury and Acute Respiratory Distress Syndrome
Since 1994, both acute lung injury (ALI) and ARDS were defined by criteria of the American European Consensus Conference (AECC) (Table 73.1A) with ARDS defined as a more severe subset of ALI. However, in 2012, a panel of experts meeting in Berlin published the “Berlin definition” to address ambiguities of the prior AECC criteria and to improve the accuracy and usefulness by applying various criteria stepwise to existing databases of patients with ALI and ARDS (Table 73.1B). According the Berlin definition, ARDS encompasses the same Pao2/Fio2 range of the old ALI, which would now be an obsolete term. The new definition of ARDS also details mild, moderate, and severe forms of the syndrome based on the Pao2/Fio2 ratio. Patients who previously were diagnosed with ALI but not ARDS (Pao2/Fio2 ≤ 300, but > 200) are now referred to as patients with mild ARDS. Because this modification to the definition of ARDS was published in 2012, virtually all of the literature published from 1994 to 2012 has utilized the AECC definition of ALI and ARDS.
Table 73-1A
A Recommended Criteria for Acute Lung Injury (ALI) and Acute Respiratory Distress Syndrome (ARDS)
Modified from Bernard GR, Artigas A, Brigham KL, et al: The American-European Consensus Conference on ARDS. Am J Respir Crit Care Med 149:818–824, 1994.
TABLE 73.1B
Criteria | Acute Respiratory Distress Syndrome (ARDS) |
Acute onset | Within 1 week of known clinical insult or new or worsening respiratory symptoms |
Radiographic studies | Bilateral opacities—not fully explained by effusions, lobar/lung collapse, or nodules |
Etiology of edema | Respiratory failure not fully explained by cardiac failure or fluid overload |
Hypoxemia | Mild ARDS: 200 < Pao2/Fio2 ≤ 300 with PEEP or CPAP ≥ 5 cm H2O Moderate ARDS: 100 < Pao2/Fio2 ≤ 200 with PEEP ≥ 5 cm H2O Severe ARDS: Pao2/Fio2 ≤ 100 with PEEP ≥ 5 H2O |
Modified from The ARDS Definition Task Force: Acute Respiratory Distress Syndrome: The Berlin Definition. JAMA 307:2526-2533, 2012.
Synonyms for ARDS include noncardiogenic pulmonary edema, shock lung, permeability pulmonary edema, and pulmonary capillary leak syndrome. The latter two terms arise from the concept that pulmonary edema in patients with ARDS is due primarily to increased permeability of the alveolar-capillary membrane at normal or modestly elevated pulmonary capillary pressures. In contrast, pulmonary edema in left-sided congestive heart failure (CHF) (i.e., elevated left atrial pressure) results from excessive filtration of plasma across the alveolar-capillary membrane as a result of the hydrostatic forces created by high pulmonary capillary pressure (see Chapter 52).
Pathogenesis and Precipitating Causes
ARDS results from injury to the alveolar-capillary membrane that is caused by exogenous agents or by endogenous inflammatory mediators. This injury results in leakage of plasma into the lung’s interstitial and alveolar spaces, with the end result being alveolar flooding and respiratory failure. It is a final common pathway in response to various initial systemic insults, including sepsis, pneumonia, and severe trauma (Box 73.1). For most of these predisposing conditions, only a minority of patients at risk actually go on to have full-blown ARDS. Although it is unclear why ARDS develops in some at-risk patients and not others, the risk of ARDS increases several-fold if the patient has multiple predisposing conditions. To date, no pharmacologic intervention has been effective in preventing ARDS in at-risk patients.
Clinical Considerations
Clinical Features
Patients with ARDS typically present with acute respiratory distress at the same time as, or shortly after, one or more of the associated precipitating causes (see Box 73.1). Their physical examination is notable for signs of respiratory distress, rapid shallow respirations with or without scattered inspiratory crackles. They often have orthopnea but no other signs of CHF. Chest radiographs often show characteristic diffuse bilateral infiltrates without cardiac enlargement. Initially, the infiltrates may be interstitial and then progress to widespread and confluent alveolar densities.
Arterial blood gas (ABG) results in very early ARDS are notable for hypoxemia, but often, there is hypocapnia with a primary respiratory alkalosis. Pao2 typically remains low, despite supplemental oxygen, because of the pulmonary shunt created by flooded alveoli (see Chapter 1). A high respiratory rate and an increased work of breathing rapidly lead to respiratory muscle fatigue, hypercapnia, and need for intubation and mechanical ventilation. Because many patients with ARDS have associated life-threatening conditions, such as hemorrhagic or septic shock, their ARDS may become evident only after initial stabilization and volume resuscitation.
Differential Diagnosis
Unlike AECC definitions of ARDS, the current Berlin definition does allow for concomitant ARDS and cardiogenic pulmonary edema. However, it is still important to differentiate ARDS from pulmonary edema resulting primarily from cardiac failure or fluid overload. Evidence in favor of CHF includes a cardiac history, an enlarged heart on chest radiograph, and a third heart sound. Rapid improvement after diuresis strongly suggests CHF without concomitant ARDS. If a pulmonary artery (PA) catheter is present, a pulmonary artery wedge pressure (PAWP) of ≤ 18 mm Hg supports the diagnosis of ARDS. However, PAWPs of 19 to 22 mm Hg are not uncommon after fluid resuscitation in patients previously diagnosed on clinical grounds prior to insertion of the PA catheter. Conversely, PAWPs in the 19 to 22 mm Hg range or even ≤ 18 mm Hg may be present at the time of measurement in some patients with CHF. One example of the latter is the patient who undergoes diuresis in the interval between the occurrence of pulmonary edema and PAWP determination. Another example is the patient who has “flash pulmonary edema,” in which transient ischemia-induced left ventricular dysfunction or papillary muscle dysfunction resolves before PAWP measurement. In general, cardiogenic pulmonary edema to the degree of confluent alveolar flooding and respiratory failure is associated with PAWP > ~28 to 30 mm Hg in patients with normal oncotic pressure and is primarily due to the imbalance of their Starling forces across the alveolar-capillary membrane.
Mechanisms of Lung Injury
Pathophysiology of ARDS
Hypoxemia
In early-phase ARDS, the most life-threatening problem is severe hypoxemia. This arises predominantly from a large right-to-left intrapulmonary shunt through numerous fluid-filled alveoli (see Chapter 1). Its magnitude can be estimated as follows: a 5% shunt is present for every 100 mm Hg decrease in Pao2 below 700 mm Hg while the patient is breathing 100% oxygen. For example, if Pao2 on 100% oxygen is 200 mm Hg, then the shunt is ~25%. (This estimate is accurate only for Pao2 values above 150 mm Hg.) Patients with ARDS who need mechanical ventilation usually have shunts in the range of 20% to 50%. Increased right-to-left shunt is the cause of the difficulty in reversing hypoxemia with supplemental oxygen, even with oxygen concentrations of 100%. For this reason, one goal of ARDS management is to decrease the shunt fraction by reopening (recruiting) alveoli that have no ventilation (i.e., whose ).
Low Compliance
Decreased lung compliance in ARDS is due to widespread interstitial and alveolar edema and atelectatic alveoli (microatelectasis). Decreased surfactant activity leads to the collapse of alveoli at end-expiration and increased hysteresis between inspiratory and expiratory pressure-volume curves. Low lung compliance results in low respiratory system compliance. For example, if a patient is on 10 cm H2O of PEEP and has a normal respiratory system compliance of 100 mL/cm H2O, a ventilator-delivered tidal volume of 500 mL would result in 5 cm H2O end-inspiratory pressure in addition to the PEEP of 10 cm H2O. Taken together, the sum results in a plateau pressure (Pplat) of 15 cm H2O (see Figure 2.3, Chapter 2). In contrast, if the patient has ARDS and a respiratory system compliance of 20 mL/cm H2O (again assuming 10 cm H2O of PEEP), the same 500 mL tidal volume would result in a 25 cm H2O addition to the PEEP of 10 cm H2O, resulting in a Pplat of 35 cm H2O.
Although the chest radiograph shows the infiltrates as diffusely uniform, computed tomographic (CT) scans of the lungs of ARDS patients indicate a more patchy distribution of fluid and atelectasis. CT scans of patients with ARDS performed at varying inspiratory pressures indicate that alveoli can be divided into three compartments: (1) completely filled and nonrecruitable alveoli, (2) atelectatic and recruitable alveoli, and (3) open alveoli (Figure 73.1). Some have regarded the recruitable and open alveoli as a “baby lung,” because they constitute a small fraction of the total lung. Lung protective strategies, such as low tidal volume ventilation, as described later, are based in part on the concept that in patients with ARDS, one is really ventilating a lung for which traditional larger sized tidal volumes (10 to 12 mL/kg predicted body weight) are much too large, resulting in alveolar overdistention and lung injury.
Increased Minute Ventilation
Patients with ARDS have increased minute ventilation. This results from marked increases in alveolar dead space, arising from microscopic level changes in ventilation-perfusion () ratios that increase the number of alveoli with
greater than 1. Overall dead space to tidal volume (Vd/Vt) ratios are commonly in the 0.7 to 0.8 range (compared with a normal Vd/Vt ratio of 0.3). As a result, the minute ventilation must be increased two to three times in order to keep the Paco2 in the normal range (see Appendix B). During mechanical ventilation this requires high inspiratory flow rates to maintain an I:E of < 1. Although high respiratory rates are needed to keep Paco2 in the normal range while simultaneously using low tidal volume ventilation, this approach can be limited by short exhalation times and dynamic overinflation (auto-PEEP; see Chapter 2). Therefore, many clinicians allow Paco2 to rise (permissive hypercapnia), prioritizing lung protective ventilation over maintaining normal levels of Paco2.
Clinical Management: Specific Therapy
Specific therapy for early-phase ARDS is limited except in cases involving treatable infections or ARDS resulting from diffuse pulmonary hemorrhage (see Chapter 78). There remains controversy regarding the role of systemic corticosteroids in late-phase persistent ARDS because of inconsistent effects on mortality and concern for side effects. Several meta-analyses comparing high doses of systemic corticosteroids to placebo in patients with ARDS have drawn conflicting conclusions with the majority failing to find a mortality benefit to steroids. Thus, they are not recommended for routine use. In contrast, a much lower daily dose of corticosteroids has become accepted therapy for patients with pneumocystis pneumonia caused by human immunodeficiency virus. Other anti-inflammatory agents have shown promise in animal studies or in preliminary human studies, but confirmation of their efficacy by large, multicenter, controlled clinical trials is lacking. Various other specific therapies targeting pathogenic mechanisms in ARDS, such as statins, are currently under evaluation in clinical trials. Therefore, the current approach to ARDS management focuses on various supportive therapies aimed at improving oxygenation and ventilation and limiting the deleterious affects of mechanical ventilation.
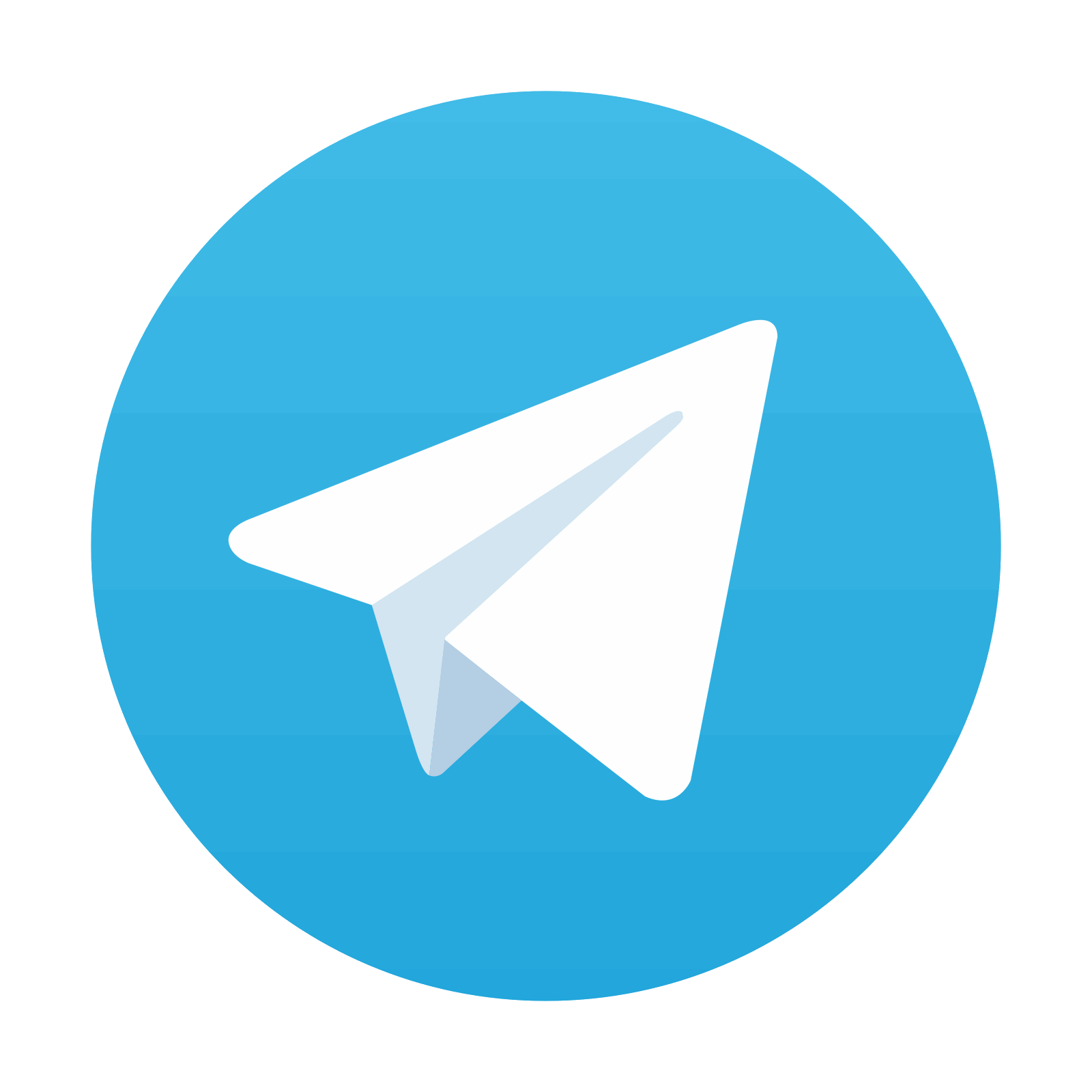