For bedside purposes, it is sufficient to remember that this daunting equation may be functionally represented as [H+] = f (pCO2, [SID], ATOT). This implies that [H+] (and also [HCO3−]) are dependent parameters, which can only be modified by changes in the three following independent parameters:
pCO2, the partial pressure of CO2. It follows from the Stewart equations that if pCO2 increases, [H+] must increase as well. This is not different from other approaches to acid base–physiology.
[SID], the strong ion difference. Strong ions are essentially completely dissociated and thus exist in charged form only. Important examples include Na+, K+, Ca2+, Mg2+, Cl−, lactate, and ketoacids. In contrast, weak ions can exist both in charged and uncharged forms. Examples include HCO3−, albumin, and inorganic phosphate. [SID] is the sum of strong cations minus the sum of strong anions. In plasma, it is mainly determined by [Na+] and [Cl−] and its normal value is about 40 mEq/l. It follows from the Stewart equations, that if [SID] decreases, [H+] must increase and vice versa. Any pathological process that disturbs the balance between strong cations and strong anions will thus directly affect pH. This includes lactic acidosis, ketoacidosis, renal acidosis, vomiting-induced alkalosis, contraction alkalosis, and most importantly iatrogenic fluid administration.
[ATOT], the total amount of weak acids. Weak acids are molecules that exist in incompletely ionized forms. They are grouped as ATOT, the total amount of weak acids, and consist mainly of plasma proteins. From an acid–base perspective, albumin and to a lesser extent phosphate are the most important contributors. It follows from the Stewart equations that if ATOT increases, [H+] must also increase. This implies that hypoalbuminemia of any cause contributes to alkalosis. Similarly, hyperphosphatemia, as seen in renal failure, causes acidosis.
The effect of the different parameters on acidity is summarized in Figure 8.1. It is easily appreciated that a decrease in [SID] exerts the strongest effect.
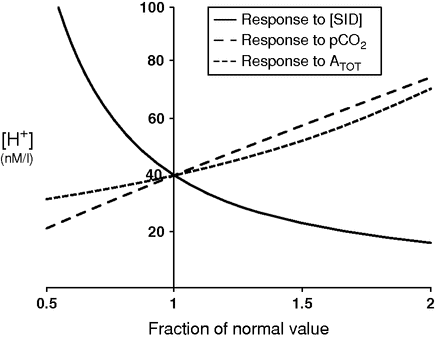
Spider plot showing the dependence of plasma pH on changes in the three independent variables: strong ion difference [SID] (normal value = 40 mEq/l), pCO2 (normal value = 40 mmHg), and total concentration of non-volatile weak acids (ATOT) (normal value = 17.2 mmol/l).
Impact of fluids on acid–base status
The pivotal role of crystalloid [SID]
If a patient is being resuscitated with large amounts of fluids, plasma [SID] will be forced in the direction of the [SID] of the fluid. For example, NaCl 0.9% has a [SID] of 0 mEq/l. Thus, giving large amounts of NaCl 0.9% will lower the normal plasma [SID]. This directly causes [H+] to rise (acidosis). Therefore, it is important to consider [SID] when choosing fluids for resuscitation.
Importantly, it is the [SID] rather than the chloride content per se that causes this effect. Hyperchloremic acidosis is, as such, a poor descriptor. Theoretically it would be perfectly possible to produce a solution that is hyperchloremic as opposed to plasma and yet would (seemingly paradoxically) lead to metabolic alkalosis, provided it contained enough cations to enlarge its [SID]. A fluid containing 120 mEq/l of chloride and 160 mEq/l of sodium in the absence of other strong ions is an example of such a solution. On the other hand, the use of a chloride-free solution will not in itself avoid acidosis. For example, glucose and dextrose 5% do not contain any chloride, but owing to the concomitant absence of strong cations, the [SID] amounts to 0 mEq/l. The acid–base effect of such a solution will be the same as NaCl 0.9%, maybe slightly less important at most owing to an increased distribution of the fluid towards the intracellular compartment.
The only way to avoid fluid-based manipulations of the acid–base equilibrium is by using fluids that respect the human [SID] after being administered. We call these solutions “balanced.” Their common characteristic lies in the fact that they contain more strong cations (mostly sodium) than strong anions (mostly chloride), and the electrical “gap” hereby created is filled by alkalizing agents, such as lactate, acetate, and gluconate, among others. These agents, sometimes called “buffers” (which is not the completely correct term from a chemical point of view) are metabolized after administration, exposing the solution’s in vivo [SID] (versus the [SID] “in the bag,” which is often 0 since lactate acts as strong anion). Classic examples of balanced solutions are Ringer’s acetate and Hartmann’s solution. More recent formulations are Plasma-Lyte® (Baxter, Deerfield Illinois, USA) or Sterofundin ISO® (B. Braun, Melsungen, Germany). An overview of the most common fluids with their respective [SID] is given in Table 8.2. The use of acetate as a buffer solution was treated with some skepticism, especially after it was abandoned in the field of hemodialysis, but nowadays is generally regarded as safe.[2] There is almost no research on the more recently used agents such as gluconate (an ubiquitous food additive) and malate, but they too seem to be without important safety issues. It would seem more straightforward to avoid the need for this extra metabolization step and use bicarbonate as the buffer of choice in balanced solutions. This is not commonly done, since it would be obligatory to use glass bottles in place of the much more flexible plastic bags.
Composition and [SID] of fluids that are frequently used across Europe. Not all solutions are available in every country
Electrolytes | |||||||||||||||||
---|---|---|---|---|---|---|---|---|---|---|---|---|---|---|---|---|---|
Cations (mEq/l) | Anions (mEq/l) | ||||||||||||||||
Nutrients Glucose (g) | Na+ | K+ | Ca2+ | Mg2+ | Cl− | HPO42− | HCO3− | Lactate | Acetate | Gluconate | Malate | SID (mEq/l) | pH | Osmolarity (mosmol/l) | |||
Unbalanced | Hypotonic | Glucose 5% | 50 | 0 | 0 | 0 | 0 | 0 | 0 | 0 | 0 | 0 | 0 | 0 | 0 | 4.20 | 278 |
NaCl 0.45% in glucose 5% | 50 | 77 | 0 | 0 | 0 | 77 | 0 | 0 | 0 | 0 | 0 | 0 | 0 | 4.30 | 432 | ||
GNaK1 | 50 | 51 | 40 | 0 | 0 | 91 | 0 | 0 | 0 | 0 | 0 | 0 | 0 | 4.50 | 460 | ||
Glucidion2/Bionolyte3 5% | 50 | 68.4 | 26.8 | 0 | 0 | 95.2 | 0 | 0 | 0 | 0 | 0 | 0 | 0 | 4.80 | 467 | ||
Isotonic | NaCl 0.9% | 0 | 154 | 0 | 0 | 0 | 154 | 0 | 0 | 0 | 0 | 0 | 0 | 0 | 5.50 | 308 | |
NaCl 0.9% in glucose 5% | 50 | 154 | 0 | 0 | 0 | 154 | 0 | 0 | 0 | 0 | 0 | 0 | 0 | 3.5–6.5 | 585 | ||
Tutofusin1 | 0 | 140 | 5 | 5 | 3 | 153 | 0 | 0 | 0 | 0 | 0 | 0 | 0 | 4.3–6.5 | 300 | ||
Hypertonic | NaCl 3% | 0 | 513 | 0 | 0 | 0 | 513 | 0 | 0 | 0 | 0 | 0 | 0 | 0 | 5.50 | 1,026 | |
Mannitol 15% | 0 | 0 | 0 | 0 | 0 | 0 | 0 | 0 | 0 | 0 | 0 | 0 | 0 | 4.5–7 | 823 | ||
Colloid | Voluven3 | 0 | 154 | 0 | 0 | 0 | 154 | 0 | 0 | 0 | 0 | 0 | 0 | 0 | 4–5.5 | 308 | |
Balanced | Hypotonic | Glucion 5%1 | 50 | 54 | 26 | 0 | 5.2 | 55 | 12.4 | 0 | 25 | 0 | 0 | 0 | 30 | 4.90 | 447 |
Rehydrex3 5% | 50 | 70 | 0 | 0 | 0 | 45 | 0 | 0 | 0 | 25 | 0 | 0 | 25 | 6 | 440 | ||
Hartmann’s/Ringer’s lactatea | 0 | 131 | 5 | 4 | 0 | 111 | 0 | 0 | 29 | 0 | 0 | 0 | 29 | 5–7 | 278 | ||
Ringer’s acetate | 0 | 130 | 5.4 | 1.8 | 2 | 112 | 0 | 0 | 0 | 27 | 0 | 0 | 27 | 6–8 | 276 | ||
Isotonic | Sterofundin ISO/Ringerfundin2 | 0 | 145 | 4 | 5 | 2 | 127 | 0 | 0 | 0 | 24 | 0 | 5 | 29 | 5.1–5.9 | 309 | |
Plasma-Lyte1 | 0 | 140 | 5 | 0 | 3 | 98 | 0 | 0 | 0 | 27 | 23 | 0 | 50 | 7.40 | 295 | ||
Ionolyte3 | 0 | 137 | 4 | 0 | 3 | 110 | 0 | 0 | 0 | 34 | 0 | 0 | 34 | 6.9–7.9 | 286 | ||
Sodium bicarbonate 1.3% | 0 | 154 | 0 | 0 | 0 | 0 | 0 | 154 | 0 | 0 | 0 | 0 | 154 | 7–8.5 | 308 | ||
Hypertonic | Sodium bicarbonate 8.4% | 0 | 1,000 | 0 | 0 | 0 | 0 | 0 | 1,000 | 0 | 0 | 0 | 0 | 1,000 | 7.0–8.5 | 2,000 | |
Colloid | Gelofusine2 | 0 | 154 | 0 | 0 | 0 | 120 | 0 | 0 | 0 | 0 | 0 | 0 | 34b | 7.1–7.7 | 274 | |
Geloplasma3 | 0 | 152 | 5 | 0 | 3 | 100 | 0 | 0 | 30 | 0 | 0 | 0 | 64b | 7.1–7.7 | 287 | ||
Gelaspan/Isogelo2 | 0 | 151 | 4 | 2 | 2 | 103 | 0 | 0 | 0 | 24 | 0 | 0 | 56b | 7.1–7.7 | 284 | ||
Volulyte3 | 0 | 137 | 4 | 0 | 3 | 110 | 0 | 0 | 0 | 34 | 0 | 0 | 34 | 5.7–6.5 | 287 | ||
Tetraspan2 | 0 | 140 | 4 | 5 | 2 | 118 | 0 | 0 | 0 | 24 | 0 | 5 | 33c | 5.6–6.4 | 296 |
1 ® Baxter, Deerfield, Illinois, USA.
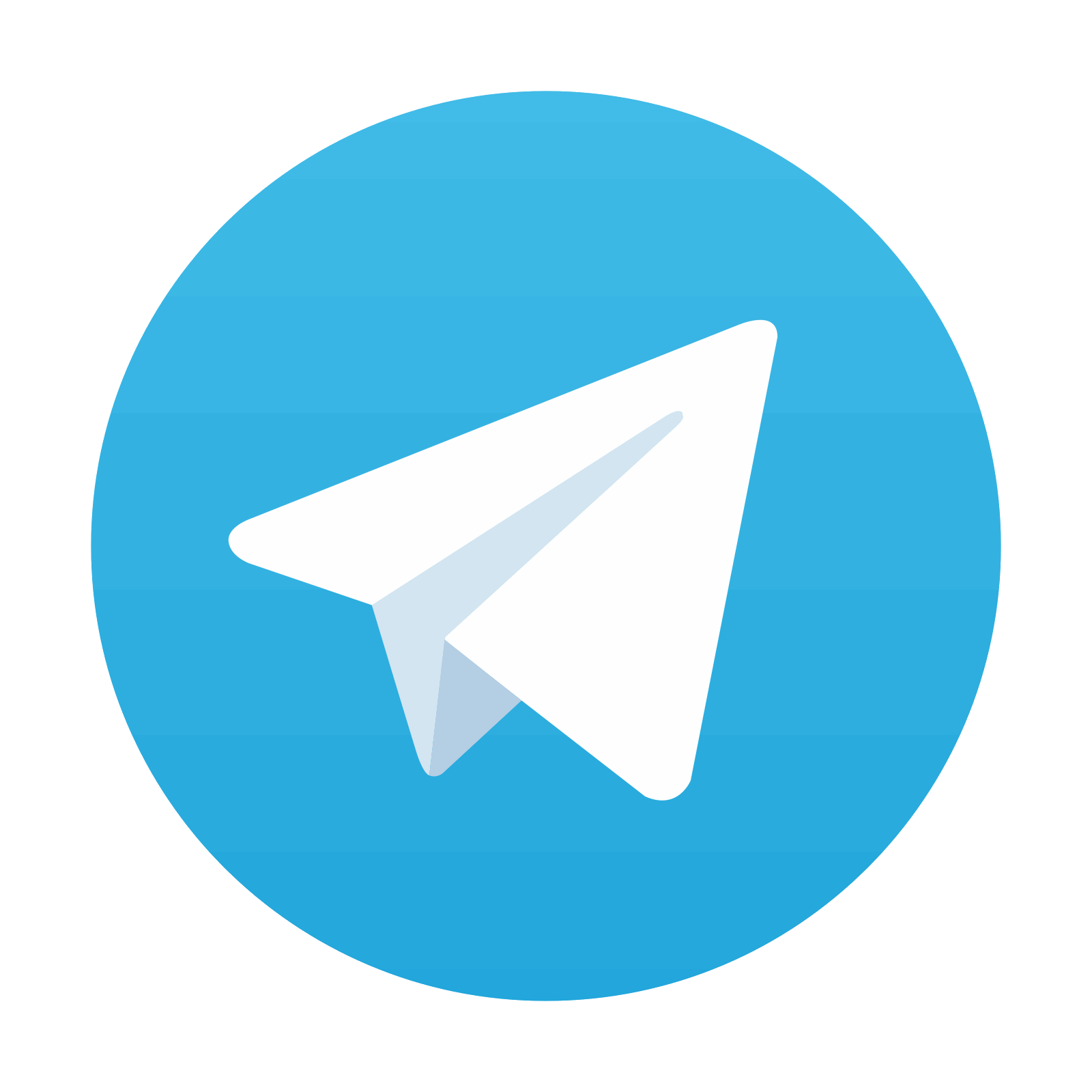
Stay updated, free articles. Join our Telegram channel

Full access? Get Clinical Tree
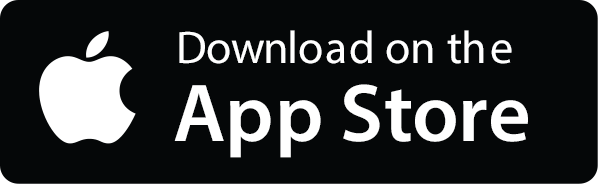
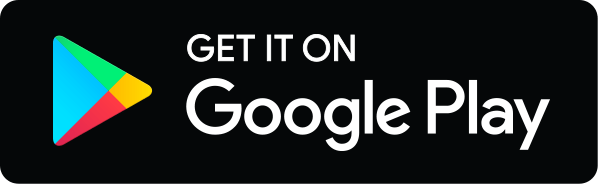
