Fig. 12.1
The shaded parabolas illustrate the transcranial photon paths from a scalp-mounted infrared (IR) light source to adjacent proximal (shallow) and distal (deep) sensors. The photon paths depicted represent those used with spatially resolved spectroscopy. With this technique, photons arriving at the shallow and deep detectors are reflected by distinct regions of the cerebral cortical microcirculation. Ratios developed from the multi-site intracranial measurements enable suppression of both extracranial reflection and interindividual variability in intracranial photon scatter. The commercial alternative of differential spectroscopy locates the shallow detector much closer to the IR source. As a result, incident photons arriving at this detector have been reflected exclusively from extracortical tissues. The differential shallow vs. deep signal suppresses extracranial reflection. Additional wavelengths of infrared light are used in an attempt to suppress intracranial photon scatter variation
Three other cerebral oximeters have received FDA clearance. The CAS Medical (Branford, CT) Fore-Sight® uses four wavelengths of infrared light with single-site extra- and intracranial measurement to produce a differential rSO2 signal [9]. Approximately 90 peer-reviewed articles have described device performance in a variety of clinical settings [10]. In addition, several studies have compared Fore-Sight® and INVOS™ performance [11–15]. In general, interdevice measurement disparities led the investigators to conclude that the rSO2 values were device specific.
Nonin Inc., (Plymouth, MN) manufacturers the EquanOX® 7600 cerebral oximeter. The analytic approach resembles that of the CAS Fore-Sight® (i.e., four infrared wavelengths and single-site extra- and intracranial differential measurement). The optode contains two light sources, so that the resulting rSO2 apparently represents an average from pairwise measurements in adjacent tissue samples [16]. About two dozen peer-reviewed articles describe device performance in various clinical settings [17]. The authors of a four-way comparison of INVOS™, Fore-Sight®, and EquanOX® (3- and 4-wavelength) devices concluded that the resultant rSO2 values were not interchangeable [4]. This situation resembles the discrepant arterial oxygen saturation measurements that have been reported between on-site invasive co-oximetry and laboratory-based complete blood count analysis [18].
The OrNim Inc. (Los Gatos, CA) CerOx® measures brain or peripheral tissue regional oxygen saturation using a combination of multiple wavelength near-infrared light and phase-modulated ultrasound [19]. Currently, there is one peer-reviewed article that characterizes its clinical performance [20]. Comparative studies with competing devices are unavailable.
Additional research instruments without FDA clearance include the (1) NIRO® 100, 200, and 300 (Hamamatsu Photonics KK, Hamamatsu City, Japan) [21], (2) Oxiplex® TSS (ISS, Champaign, IL) [22], and (3) O3® (Masimo, Irvine, CA) [23]. Their clinical application requires an FDA Investigational Device Exemption and/or an Institutional Review Board-approved research protocol with informed patient consent.
Transcranial NIRS Technology
Regional Cerebral Oxygen Saturation Measurement
With the exception of infants, brain mass is too large for transillumination, and as a result, NIRS in adults and children relies on reflectance spectroscopy. An optical electrode or optode, containing both near-infrared and infrared light sources as well as sensors to detect light reflected from the underlying tissue, is fixed on the calvarium. The optode is generally placed on glabrous skin to avoid contamination by environmental infrared light. Light-tight seals around the sensor(s) aid in artifact suppression. In the proximity of intense infrared sources, it may be necessary to drape the cranium with infrared-opaque material. Disposable optodes contain electroconductive material referenced to the instrument. These optodes should be isolated from electrophysiologic electrodes or ultrasound probes to prevent artifactual contamination or signal degradation.
Large, reusable optodes require fixation and mechanical stabilization with a strap or elastic band, whereas small disposable optodes rely on an adhesive patch that also serves as a light shield. Because of variations in anatomy, skull curvature, and the position of sinus cavities and surface blood vessels, accuracy may be compromised if disposable optodes are placed contrary to the manufacturer’s instructions [24, 25]. In particular, placement over the sagittal sinus is potentially problematic due to the large amount of pooled venous blood found there and its confounding effects on photon absorption and scattering [24]. Furthermore, disposable optodes designed for adults should not be used on patients weighing less than 40 kg. Pediatric and neonatal optodes are available from some manufacturers for small patients. Regardless of the optode style, care must be taken to avoid excessive or prolonged compression of the underlying sensitive forehead or scalp tissue. The FDA-cleared optodes have a flat design to minimize tissue compression.
Manufacturers should provide convincing data that the device is actually capable of measuring cerebral as opposed to cranial hemoglobin [7]. Known changes in cerebral perfusion or oxygenation should be manifest by appropriate change in the intracranial oxygenation metric [7]. Evidence should verify that extracranial contamination does not substantially confound interpretation [6]. By measuring optical signals obtained from two detectors placed at different distances greater than 2 cm from the infrared source, oximeter systems utilizing spatially resolved spectroscopy (i.e., INVOS™ and NIRO®) measure the slope of absorption change as a function of photon penetration depth. Their proprietary algorithms partially suppress the confounding influences of interindividual differences in intracranial photon path length and scattering [1].
FDA-cleared cerebral oximeters display rSO2 trends as a function of time. Research devices may additionally provide estimates of change in the concentrations of oxy-, deoxy-, and total hemoglobin as well as parenchymal cytochrome aa3. The trends of some devices represent moving averages, while others are a time series of discrete measures. Because smoothing can dramatically affect the responsiveness of the trends to important physiologic perturbations, the averaging time constant should be clearly described by the manufacturer and understood by the user.
Now it is not possible to directly confirm the accuracy of transcranial measurement of regional cerebral oxygen by an independent analytical method. The closest approximation to this goal has been the comparison with field saturation [5]. As with other neuromonitoring modalities, optimal recording technique dictates that a simple calibration method should be available to confirm that both the optode and recording electronics/software are functioning properly. Although some manufacturers currently provide a calibration device for their recording electronics, none is available for use with individual optodes.
Safety Considerations
Potential users of this technology should ensure that NIRS devices intended for clinical application successfully restrict environmental current flow to patient connections and between optodes. The low-intensity light sources used in these devices are similar to those found in pulse oximeters and do not appear to represent a safety hazard [25]. The higher powered laser light source used in the Ornim oximeter is rated as Class 1, meaning that there is no evidence that its use can lead to potential injury. With any of these devices, care must be taken to avoid undue pressure of the optode against the unprotected forehead or scalp, particularly in infants and young children. Some manufacturers recommend that the skin should be checked for irritation and sensors replaced daily.
Technical Considerations
Cerebral oximeters are relatively immune to motion and electrocautery interference that plague electrophysiologic and ultrasound measurements. The most troublesome artifacts for NIRS are environmental light contamination and light piping. Especially in infants, intense infrared light (i.e., heat source) can readily penetrate the skull opposite the optode and artifactually elevate reflected intracranial light by transillumination. An elevated extracranial signal may occur in adults through the use of a pulse oximeter probe placed on the nasal bridge or forehead, which illuminates the frontal sinuses with infrared light [26]. Contamination may also occur through light piping. Translucent material such as hair, perspiration beads, or moisture condensate in the proximity of the sensors can act as a conduit for environmental infrared light.
Although rSO2 measurement is relatively insensitive to melanin and other normal skin pigments [27], anatomic anomalies or underlying pathology in darkly pigmented patients may have a confounding influence [28]. Systems utilizing two wavelengths cannot distinguish between normal and pathologic hemoglobin moieties . The presence of methemoglobin or other abnormal hemoglobins will result in a functional saturation measurement that can be quite different than the fractional measurement. Non-heme chromophores such as bilirubin and biliverdin [29, 30], as well as some intravascular dyes like indigo carmine and methylene blue [31], absorb photons and reduce signal intensity. In contrast, other dyes such as indocyanine green may falsely elevate rSO2 values [32].
The intracranial presence of extravascular blood (i.e., hematoma or hemorrhage) within the frontal tissue sampled by the oximeter renders the rSO2 estimate ambiguous [33]. A fundamental assumption of the computational algorithms used in several NIRS devices is that the infrared signal reflects exclusively intravascular hemoglobin. Mixture of this signal with that obtained from a stagnant pool of poorly or unoxygenated blood can result in uninterpretable values. This consideration also influences sensor placement. A midline locus should not be used in order to avoid placement over the sagittal sinus. A superior placement on the forehead also helps to avoid the frontal sinuses [26].
Placement directly over a metabolically inactive cortical infarct may result in indeterminate information because of the lack of oxygen extraction from sequestered venous blood. However, several recent studies have observed abnormally low rSO2 values sampled from regions containing cerebral cortical infarction [34, 35]. Optode placement over regions of damaged or absent brain tissue (i.e., hemispherectomy) may result in spurious readings. In addition, a postcraniotomy metal plate implant obviously makes monitoring impossible. Conversely, the absence of frontal bone can result in overscaled reflected signals [36]. Skull defects and abnormal frontal sinus anatomy can often be identified simply through transillumination with a flashlight.
Limitations of Cerebral Oximetry
On the basis of current clinical literature and expert opinion, cerebral oximetry has some limitations as a monitoring tool. First, direct validation of the accuracy of rSO2 currently is not possible because of the absence of true noninvasive measures of brain oxygen saturation and oxy- and deoxyhemoglobin and cytochrome aa3 concentrations. Second, baseline brain oxygen saturation measurement appears to be influenced by sensor position on the forehead, and at present, sensor placement is limited to glabrous skin. Finally, because rSO2 represents focal measurement of prefrontal cortex, regional hypoperfusion affecting large portions of the intracranial anterior or posterior circulations may be invisible to forehead sensors.
Rationale for Cerebral NIRS Monitoring
The generic rSO2 trend measures change in regional cerebral microcirculatory oxygen supply balance. Currently, this information is not otherwise available in the perioperative or critical care settings. This NIRS technology can prove invaluable as an aid in interpreting electrophysiologic or cerebral hemodynamic data. Change in electrophysiologic signals indicates functional alteration in a specific neural pathway or region, but does not indicate the underlying cause or the most appropriate corrective action . Similarly, transcranial Doppler (TCD) ultrasound documents change in blood flow velocity through the large basal cerebral arteries, but provides no information about the underlying functional status of the neural tissue at risk. In either case, concomitant rSO2 monitoring aids in determining the:
- 1.
Development of a potentially injurious oxygen imbalance
- 2.
Nature of the imbalance
- 3.
Potential appropriate corrective action
- 4.
Patient response to intervention.
The INVOS™-derived normative median rSO2 for healthy adults, children, and neonates, respectively, are 65 % [27], 71 % [37], and 78 % [38], whereas lower values have been described for adult (62 %) [39], pediatric (60 %) [40], and neonatal (67 %) [41] patients with cardiac disease. Because rSO2 reflects a composite of arterial and venous blood, normative values are higher than published values for jugular venous oxygen saturation (SjvO2) ranges for both healthy adults [42] and cardiac surgery patients [43]. Although conscious baseline rSO2 values appear to be device specific, currently only limited normative values are available for the other cerebral oximeters [9, 16].
Both noninvasive rSO2 [44] and invasive brain field saturation [5] are associated with hemoglobin concentration and hematocrit. The relationships appear to be complex and nonlinear [45], such that rSO2 is independent of hematocrit at fractions greater than 0.3 [46]. Thus, the apparent relationships between these measures and low hematocrit and [hemoglobin] seem to reflect inherent properties of cerebral oxygen saturation that occur with inadequate oxygen delivery.
Kishi et al. [44] observed in adolescent and adult patients that rSO2 was significantly correlated with patient age. Yet, this apparent age-dependency may be simply a manifestation of underlying pathology (i.e., older patients tend to have more disease and dysfunction). A study by Baikoussis et al. [45] involving adult and geriatric patients supports this interpretation.
This study also documented close interhemispheric agreement in preanesthetic baseline rSO2 measurements. In contrast, marked asymmetry is the rule with SjvO2. Indeed, SjvO2 right-left asymmetry greater than 10% has been reported to occur in two thirds of patients [46].
In a cohort of more than 2097 adult cardiac surgery patients, INVOS™ preoperative rSO2 values less than 60 % were associated with increased risk of morbidity and mortality [47]. A similar study involving 1178 patients found even greater risk with rSO2 baseline below 50 % [39]. Subnormal intraoperative rSO2 is associated with heightened risk of postoperative delirium and neurocognitive dysfunction [48–53].
Despite the establishment of normative absolute rSO2 values, it should be appreciated that the number represents exclusively a measure of highly regional intracranial hemoglobin saturation. Individualized static baseline rSO2 values correlate with cardiac function. However, these static values do not necessarily provide direct information about cerebral well-being [54, 55].
Thus, values within the normative range may be obtained from patients with cerebral infarction or brain death [56]. Cadaveric rSO2 values may also lie within the normative range [57] because postmortem cerebral venous oxygen saturation ranges between 5 and 95 %, depending on the cause of death and body storage conditions [58]. Because both the pulse and cerebral oximeters are functionally spectrophotometers, they may generate normative saturation values from illumination of chromophore-containing inanimate objects (i.e., pumpkins) [59].
Preoperative Factors
Interpretation of the clinical significance of cerebral dysoxygenation during surgery or critical care is heavily influenced by underlying patient pathophysiology. There are a number of preexisting factors that affect both the static rSO2 value and its dynamic response to physiologic change. These factors involve primarily influences on oxygen delivery. Thus, patients with chronic hypertension or diabetes mellitus may have impaired cerebral pressure autoregulation. In such cases, oxygen delivery may be transiently impaired by orthostasis or other positional change. Delivery may also be impaired through vascular stenosis, cardiac dysfunction with low cardiac output, anemia, pulmonary dysfunction, or right heart/pulmonary artery congenital anomalies [60]. These various disorders explain, in part, the wide range of baseline values observed in awake surgical patients prior to preoxygenation.
Systemic Arterial Pressure
The principle of cerebral autoregulation states that cerebral perfusion (i.e., oxygen delivery) is independent of mean systemic arterial pressure over a wide range (i.e., 50–150 mmHg). In healthy individuals, profound hypotension is required to significantly reduce oxygen delivery with a concomitant rSO2 decrease. However, even a small pressure decrease within the normal autoregulatory range may result in rSO2 decline if autoregulation is diminished or entirely absent [8]. Cerebrovascular insufficiency from dysautoregulation may be secondary to a preexisting pathology (e.g., stroke) or be iatrogenically induced during surgery (e.g., volatile anesthetics, nonpulsatile perfusion, hypocapnia, hypothermia) [61]. Recently, reports have described the benefit of cerebral oximetry in the detection of position-related cerebral hypoperfusion while systemic arterial pressure remained within the normally acceptable range [62]. Availability of the arterial pressure trend is essential for interpretation of rSO2 change.
Systemic Arterial Oxygenation
Delivery of oxygen to the brain depends, in part, on the relative concentration of oxygenated hemoglobin in the arterial blood. An inadequate oxygen supply will thus eventually result in decreased arterial oxygen saturation. However, because systemic oxygen reserves far exceed those of the metabolically active healthy brain [63], a venous-dominant rSO2 decrease will often precede systemic arterial oxygen desaturation [64].
Oxygen delivery also depends on the availability of hemoglobin. Blood loss or hemodilution can result in an insufficient supply of oxygen to meet brain demands, even though the cerebral perfusion pressure, flow, and arterial oxygen saturation are within normal limits [8]. It is essential to note any marked change in hemoglobin that may accompany sudden rSO2 changes [65]. Because of the sensitivity of rSO2 as a measure of inadequate hemoglobin concentration, it has been proposed as a factor to be considered in determining transfusion triggers [66].
Systemic Arterial CO2 and pH
Normally, cerebral arteries are exquisitely sensitive to hydrogen ion shifts and thus to CO2 changes. For example, middle cerebral artery flow velocity typically changes approximately 4 % for each 1 mmHg change in the arterial CO2 partial pressure [67]. This vasomotor reactivity (VMR) may be alternatively measured with cerebral oximetry. INVOS™-determined normal VMR has been defined as approximately 1 % rSO2 change for each 1 mmHg CO2 change [68]. Because hyper- and hypocapnea have profound effects on normally reactive cerebral arteries, an end-tidal or arterial carbon dioxide trend may be invaluable in the interpretation of rSO2 changes.
With rising arterial CO2 tension , the absence of a parallel rSO2 increase suggests impairment of both cerebral VMR and autoregulation. Furthermore, an interhemispheric VMR asymmetry warns of a possible vasculopathy (i.e., intracranial stenosis or silent infarct). Anesthesia providers armed with this information may optimize perfusion management to maintain cerebral perfusion in both hemispheres [68].
Cerebral Blood Flow Obstruction
Systemic arterial pressure reflects perfusion at sites distant from the head. Obstruction to one or more vessels carrying blood to the brain may result in a profound decrease in regional cerebral oxygen delivery without altering either systemic pressure or oxygenation. For example, with bilateral carotid disease and an incomplete circle of Willis, head rotation may obstruct flow by vertebral artery compression [69]. Of course, mechanical carotid occlusion for endarterectomy may produce regional ischemia in the absence of a functionally intact collateral perfusion [70]. Alternatively, during cardiopulmonary bypass, a malpositioned aortic cannula or balloon occluder may block flow to a critical head vessel [71]. Continuous delivery of oxygen to the brain is impeded if venous outflow is compromised (i.e., edema or malpositioned caval cannula). Such obstruction will decrease rSO2 [72].
Temperature
Changes in rSO2 cannot be fully understood without knowledge of the cranial temperature fluctuations . Cooling is used for neuroprotection during surgery requiring circulatory arrest because hypothermia, in part, decreases brain oxygen requirement. Thus, during cooling, a nonlinear rSO2 rise indicative of cerebral hyperoxia may occur despite a decline in cerebral blood flow [69]. Because the magnitude of hypothermic reduction in cerebral oxygen demand varies markedly among individuals, monitoring of cerebral oxygen balance helps to determine the optimal temperature for circulatory arrest. With progressive cooling, rSO2 typically reaches a plateau with cortical synaptic quiescence (i.e., flat-line EEG) [69]. During rewarming, cerebral blood flow may fall relative to metabolism due, in part, to a cold-induced vasoparesis [73]. The severity of the potentially injurious flow-metabolism mismatch (i.e., vasoneural uncoupling) is manifested by a proportionate rSO2 decrease [74].
Anesthetic Adequacy
Approximately 60 % of cerebral oxygen consumption is spent on interneuronal communication [75]. Therefore, rSO2 is influenced by the choice of anesthetic agent and resulting magnitude of cerebrocortical synaptic depression. Agents such as the opioids exert much of their action at subcortical sites. With these agents, rSO2 may remain relatively unchanged over a wide dose range. Alternatively, the volatile halogenated agents, propofol and barbiturate hypnotics can virtually eliminate interneuronal signaling (i.e., flat-line EEG). This metabolic suppression typically results in rSO2 increase [76]. Similarly, inadequate anesthetic may lead to increased oxygen consumption in the waking brain accompanied by rSO2 decrease. Therefore, intraoperative interpretation of rSO2 is aided by an objective, quantifiable measure of cerebrocortical synaptic activity (i.e., hypnotic adequacy).
Seizure Activity
With intact vasoneural coupling, increased neural activity produces cerebral blood flow increases that are far larger than associated local metabolic increases [77]. Because this hemodynamic response has variable onset and duration, intermittent activation leads to complex oscillations in hemoglobin saturation, causing a transient rise in the venous-dominant rSO2 measurement [78]. Based on this phenomenon, a rapidly oscillating rSO2 trend has been used to detect suspected seizure activity in chemically paralyzed, ventilated patients [77, 78]. This situation is not uncommon, since subclinical seizures are thought to occur in up to one quarter of neurocritical care patients [79].
Supplemental Cerebral Perfusion
For surgery requiring hypothermic circulatory arrest, supplemental cerebral perfusion (SCP), both antegrade (ACP) and retrograde (RCP), appear to provide added neuroprotection [80]. Despite the widespread use of these techniques, optimal management procedures have not been established and vary widely among institutions. Cerebral oximetry has aided management in several important ways. First, it identifies an SCP requirement. As the hypothermia-elevated rSO2 declines toward a predetermined oxygen debt threshold [81], it warns the surgical team of the impending need to initiate SCP. Second, the rSO2 trend provides direct objective evidence that cerebral perfusion actually has been established and is appropriate to avoid either hypo- or hyperperfusion [82]. Absent this evidence, one is typically forced to rely on an unjustified assumption of SCP flow adequacy. The evidence vs. assumption dichotomy may help explain the wide range of published RCP clinical experiences, since many lack either rSO2 or ultrasonic evidence of retrograde blood flow.
Case reports have documented rapid rSO2 detection of ACP hemispheric flow asymmetry due to perfusion cannula malposition [83], or an incomplete circle of Willis [82]. Neither of these events is rare [84, 85]. The recent study of Senanayake et al. [86] illustrates the potential clinical value of rSO2 guidance for SCP management . They found in a series of 27 adult ACP-supported aortic arch repairs that by maintaining rSO2 at baseline level, postoperative neurodeficit could be completely avoided.
Rationale for Peri-spinal NIRS Monitoring
Recently, a custom-built NIRS optode and an indocyanine green tracer technique were used to validate translaminar peri-spinal oxygen saturation (SsO2). The neuromonitoring technique demonstrated both local blood flow autoregulation and CO2 reactivity [87]. SsO2 monitoring has been achieved by placing FDA-cleared optodes designed for adult transcranial tissue oximetry over the lower thoracic and upper lumbar spine. Badner et al. [88] described the application in two thoracic aortic aneurysm endovascular repairs. In the first, thoracic sensor SsO2 decline was corrected with mean arterial pressure (MAP) increase and cerebral spinal fluid (CSF) drainage. In the second, an uncorrectable SsO2 decline of greater than 50 % followed deployment of a thoracic endograft and presaged postoperative paraplegia. A contemporaneous case report examined the SsO2 vs. MAP relationships before and after deployment of an arch endograft [89]. Postoperatively, SsO2-guided blood pressure management until autoregulation eventually returned. This observation suggests that SsO2 monitoring may offer insight into the genesis and prevention of delayed spinal cord ischemic injury. The SsO2 aortic endograft experience has also been replicated in open thoracoabdominal aneurysm repair [90]. In this clinical population, Etz et al. [91] performed a pilot study using commercial optodes placed bilaterally over lower thoracic and upper lumbar paraspinous vascular collateral networks (CNs) [91]. Declines in lumbar CN oxygenation appeared to be directly linked to aortic perfusion compromise.
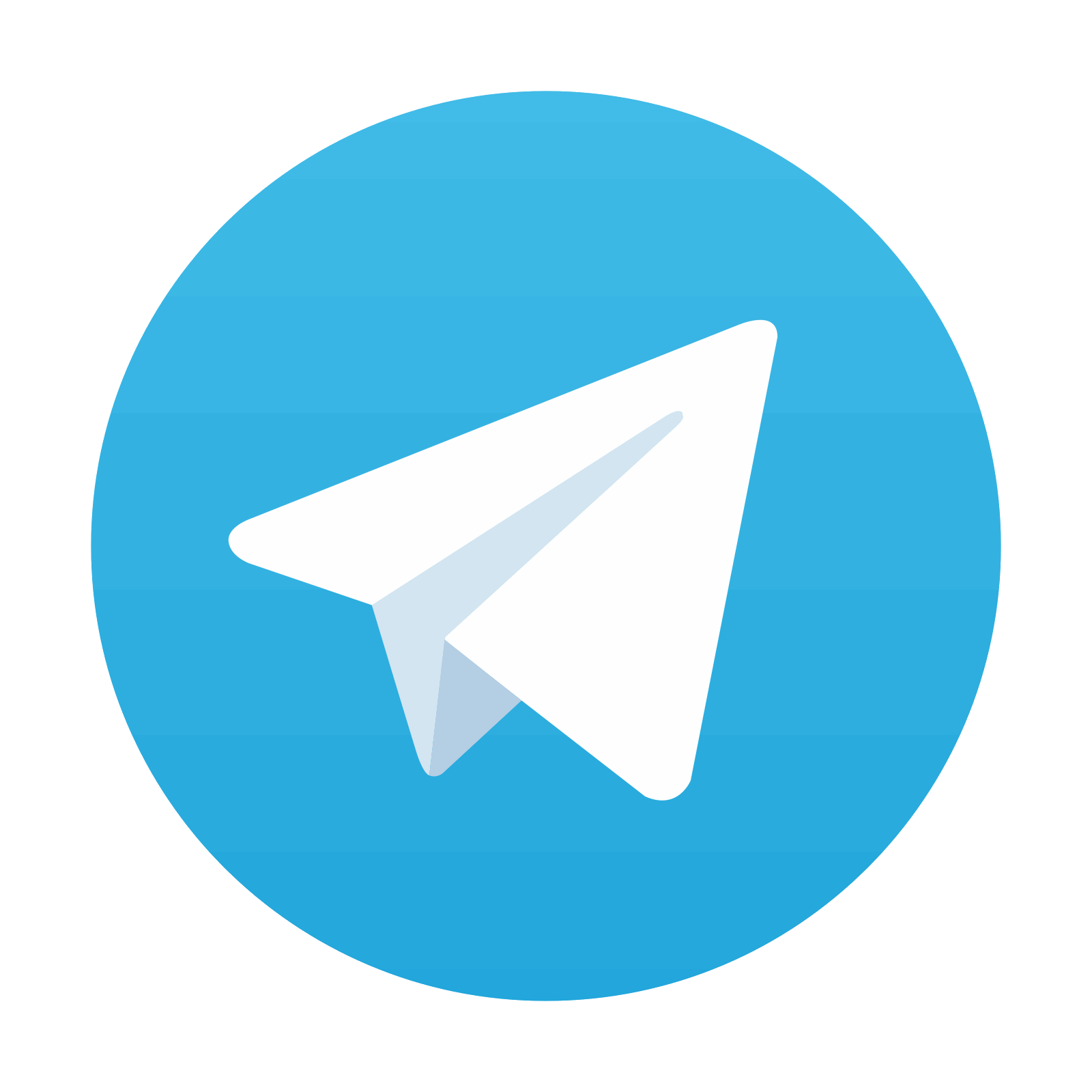
Stay updated, free articles. Join our Telegram channel

Full access? Get Clinical Tree
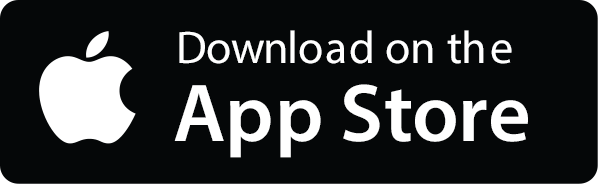
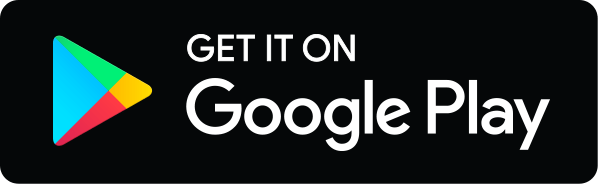