Humidification (air to trachea)
Dry room air is humidified.
The addition of the partial pressure of water reduces the partial pressure of oxygen.
PO2 = FiO2(PATM – PH2O)
Ventilation (trachea to alveoli)
Contribution of alveolar carbon dioxide.
Diffusion (alveoli to pulmonary capillaries)
Diffusion barrier for O2 is negligible in healthy lungs, accounts for a tiny reduction in PO2
Shunt (pulmonary capillaries to arteries)
Shunt equation QS∕QT = (CcO2 – CaO2)∕(CcO2 – CvO2)
Perfusion and oxygen uptake
Along the course of systemic capillaries, oxygen diffuses from blood into cells down its concentration gradient resulting in lower blood PO2 distally
Mitochondria receive oxygen via diffusion from blood across tissues and into cells and thus may have extremely low PO2 depending on oxygen supply and barriers to diffusion
Venous blood
Lower PO2 than arterial blood due to uptake by tissues
Typical oxygen saturation about 75%
Should PO2 be reduced at any point in the cascade, such as pulmonary oedema causing a barrier to diffusion, each subsequent step will also have reduced PO2. The opposite is also true: breathing oxygen-enriched air will increase PO2 at each step.
Haemoglobin (Hb) is the main carrier of oxygen within the body
Each Hb molecule is capable of binding 4 molecules of O2, forming oxyhaemoglobin
Hb has a high affinity for oxygen in well-perfused areas of normal pH, and a lower affinity in acidic environments (including high PCO2, the Bohr effect), promoting the release of O2 in peripheral or under-perfused tissues
Hb concentration is one of the main determinants of blood oxygen carrying capacity
Note: Previously, a multiplier of 10 was used to convert [Hb] measured in g/dl to g/l in order to correspond with cardiac output measurements. Modern measurements are given in g/l meaning this multiplier is no longer required. The equation can also be described using cardiac index rather than cardiac output.
Using the oxygen flux equation, it follows that altering any individual component will affect oxygen delivery to the tissues.
Cardiac output: manipulated by increasing heart rate (sympathetic drive, adrenaline, vagolytics) and stroke volume (volume status to increase preload, increase contractility with inotropes)
Haemoglobin concentration: may be increased by preventing blood loss, increasing Hb production (erythropoietin, iron supplementation) or transfusion
Oxygen saturation: increase by optimising each stage of the oxygen cascade. Increase FiO2 with supplemental oxygen, minimise diffusion gradients and shunt by treating underlying lung pathology and ensuring optimal ventilation (PEEP, adequate tidal volumes, minimising oedema, etc.)
Dissolved oxygen: under normal conditions, dissolved oxygen content is negligible in comparison with that bound to Hb, and manipulation of it is rarely clinically relevant. Increasing FiO2 and atmospheric pressure (in a hyperbaric chamber) will both increase the dissolved content. This follows Henry’s Law, which states that the amount of gas dissolved in a solvent is proportional to its partial pressure above the solvent (at a constant temperature)
Increasing altitude results in a decrease in atmospheric pressure
At sea level the atmospheric pressure is 101.3 kPa giving a PO2 of 21 kPa. Ascending to 5486 m (18 000 ft) drops the atmospheric pressure to half that at sea level, whilst the atmospheric pressure at the summit of Mount Everest at 8848 m (29 029ft) is 34 kPa, a third that of sea level
The percentage of oxygen in the atmosphere, and hence FiO2, remains the same at 21%
The partial pressure of oxygen falls in keeping with the reduction in atmospheric pressure. Thus, the oxygen content of a given volume of atmospheric air is reduced at altitude
With a reduction in inspired PO2, total blood oxygen content falls
The initial flat section of the oxyhaemoglobin dissociation curve explains the ability of Hb to maintain high oxygen saturation despite falling PO2, until an inflection point is reached at which point saturation falls off rapidly on the steep part of the curve
Physiological changes occur at altitude, known as acclimatisation, in an attempt to offset the fall in available oxygen (e.g. hypoxic ventilatory response causing increased minute ventilation, increased production of erythropoietin to increase Hb concentration)
Physiology and Biochemistry
Question 4B
The autonomic nervous system is the part of the nervous system that regulates the involuntary homeostatic functions of the body, often via reflex pathways.
It is divided into two systems:
parasympathetic
sympathetic
Differences exist between the two systems in terms of their anatomy, pharmacology and functions performed.
What are some of the key functions of the parasympathetic (PNS) and sympathetic nervous system (SNS)?
The functions of the parasympathetic system are more involved in the resting state and digestive functions, so termed ‘rest and digest’. By contrast, the sympathetic system involves stress reactions and the ‘fight or flight’ functions. They normally act in opposition to achieve regulation of the organs they innervate; further details are given in the table below.
Key functions of the parasympathetic and sympathetic nervous systems
Can you describe the anatomy of the outflow of the PNS and SNS?
Most organs receive input from both SNS and PNS
◦ Exceptions include:
▪ PNS only: lacrimal glands
▪ SNS only: adipose tissue, piloerector muscles, juxtaglomerular apparatus
Parasympathetic system has craniosacral outflow.
◦ Cranial outflow:
▪ cranial nerves 3 / 7 / 9 / 10 (oculomotor, facial, glossopharyngeal, vagus)
▪ efferents originate in the brainstem from the specific motor nuclei of these nerves
▪ vagus is major cranial efferent supplying parasympathetic fibres to abdominal and thoracic organs
◦ Sacral outflow:
▪ S2–S4
▪ supply pelvic viscera
◦ Long pre-ganglionic fibres
◦ Short post-ganglionic fibres
◦ Ganglia are near effector organs and a distance away from spinal cord
Sympathetic system has thoracolumbar outflow
◦ T1–L2, via ventral nerve roots
◦ Efferents also from hypothalamus and medulla
◦ Pre-ganglionic fibres: short and myelinated. Pass via white rami communicantes and synapse in sympathetic ganglia
◦ Post-ganglionic fibres: long and unmyelinated. Grey rami communicantes leave the ganglia and travel as C-fibres to innervate viscera
◦ Pre-ganglionic fibres may also ascend or descend the sympathetic chain prior to synapsing within ganglia of different levels
◦ The adrenal medulla is innervated directly by pre-ganglionic fibres
Describe the ganglia of the autonomic nervous system
Parasympathetic
Cranial nerves pass through ganglia to deliver their functions
◦ CN 3 → ciliary ganglion → eye
◦ CN 7 → pterygopalatine + submandibular ganglia → salivary + lacrimal glands
◦ CN 9 → otic ganglion → larynx + tracheobronchial tree
Vagus nerve and sacral outflow have less distinct ganglia and form plexuses of nerves close to their target organs supplying parotid gland/heart/proximal gastrointestinal tract and distal gastrointestinal tract/bladder/genitalia respectively
Sympathetic
Sympathetic ganglia fuse to form the sympathetic chain bilaterally, anterolateral to and running in parallel with the spinal cord
Consists of:
◦ 3 cervical ganglia (superior, middle and lower cervical ganglia)
◦ lower cervical and upper thoracic ganglia fuse to form the stellate ganglion
◦ 12 thoracic ganglia
◦ 4 lumbar ganglia
◦ 4 sacral ganglia
Outflow also forms plexuses:
◦ cardiac (+ vagal contribution)
◦ coeliac (largest sympathetic plexus + right vagal branch)
◦ hypogastric
Parasympathetic
Sympathetic
◦ Pre-ganglionic fibres: acetylcholine (ACh) acts at nicotinic receptors
◦ Post-ganglionic fibres: noradrenaline (except sweat glands where ACh acts on muscarinic receptors)
◦ Sympathetic effects depend on the nature of adrenoreceptors activated: α-1 receptors will cause vasoconstriction, whereas β-1 receptors will cause positive inotropy and chronotropy
Parasympathetic:
Sympathetic:
Physiology and Biochemistry
Question 4C
Glucose is absorbed from the small intestine by:
passive diffusion (80%)
carrier-mediated transport (20%): an active process using a Na+/glucose co-transport mechanism
Blood glucose levels are regulated by several hormones acting mainly on the liver, the most important of which are insulin and glucagon
Insulin’s main effect is to reduce blood glucose concentrations by promoting glucose uptake into cells, increasing glycogen synthesis and reducing both glycogenolysis and gluconeogenesis
At low plasma glucose levels, insulin production falls whilst glucagon stimulates a rise in glucose levels
How is insulin produced?
Insulin is a polypeptide anabolic hormone
Produced by β-cells in the pancreatic islets of Langerhans
Secreted by the pancreas into the duodenum in response to high plasma glucose, but there is always a basal level of insulin secretion
Initial rapid phase of secretion due to release of stored insulin, followed by slower phase of secretion of both stored and newly synthesised insulin
Other stimuli for insulin secretion include specific amino acids (from metabolism of food), glucagon, gut hormones released after feeding, and direct neural modulation via the autonomic nervous system
Acts on insulin receptors located on muscle and adipose cells to phosphorylate and activate tyrosine kinase. This triggers a cascade of intracellular proteins and recruits glucose-transport proteins to the cell membrane. Glucose permeability increases allowing glucose flux into the cell, thus reducing plasma glucose
Inhibited by somatostatin and catecholamines acting on α-2 adrenoreceptors
In hepatocytes the cell membrane is already permeable to glucose. Insulin does not increase glucose transport into these cells but alters intracellular metabolic processes, creating a favourable diffusion gradient for glucose uptake
What are the effects of insulin?
Multiple mechanisms of action with the overall effect of lowering plasma glucose concentration
Increased glucose uptake by enhancing facilitated diffusion of glucose into cells
Increased glycogen synthesis, reduced glycogenolysis
Reduced gluconeogenesis
Reduced hepatic release of glucose
Enhances protein formation and storage
Increased fat synthesis and reduced fat breakdown
Reduced ketogenesis
Electrolyte shifts: K+ moves intracellularly (mainly in muscle and liver), thus insulin can be used to treat hyperkalaemia
The fall in blood glucose is sensed by pancreatic β cells, leading to reduced insulin secretion
This results in lesser effects of insulin on liver enzymes, and thus promotes gluconeogenesis and glycogenolysis
Pancreatic α cells also sense the reduced blood glucose level and increase glucagon secretion
The overall effect will be to increase plasma glucose concentration
Insulin and glucagon have opposing effects and thus the overall physiological response to this pair of hormones is determined by their ratio. After a meal, insulin/glucagon ratio is 30:1. This falls to 2:1 after overnight fasting, and 0.5:1 after prolonged fasting
How is glucagon produced?
Glucagon is a polypeptide catabolic hormone
Produced by α-cells in the pancreatic islets of Langerhans
Production is stimulated by hypoglycaemia and inhibited by hyperglycaemia
Again, a variety of amino acids stimulate its release (different to those that stimulate insulin release), as well as cholecystokinin, gastrin and secretin. Inhibited by fatty acids and somatostatin. Physiological stress, hunger and exercise promote its release
Liver is the main site of action
Increases plasma glucose concentration by several mechanisms
Enhances hepatic glycogenolysis and gluconeogenesis
Inhibits glycolysis
Stimulates lipase in adipose tissue leading to the break down of fat to fatty acids and glycerol, which can then be used for glucose production and a source of energy. In the absence of insulin, the oxidation of fatty acids produces ketones, as seen in diabetic ketoacidosis
Stimulates release of insulin, growth hormone and somatostatin
Positive inotropic and chronotropic effects (hence use in beta-blocker OD)
Are there any other substances that affect blood glucose levels?
Yes, they include the following:
Glucocorticoids: reduce cellular glucose uptake and utilisation
Growth hormone: has an anti-insulin effect
Catecholamines: produced in severe hypoglycaemia, enhance glycogenolysis and reduce glucose uptake by cells
Thyroid hormones: cause increased cellular uptake of glucose, as well as increasing glycolysis, gluconeogenesis and glucose absorption from the gut
Glucose is freely filtered at the glomerulus, the amount being directly proportional to its plasma concentration
Glucose is normally completely reabsorbed in the proximal tubule of the kidney. More distal parts of the nephron also contribute should the concentration be very high, as in diabetes mellitus
Glucose reabsorption occurs in two stages
Stage 1: glucose uptake from tubule to epithelial cell. Co-transport with sodium by sodium–glucose linked transporter (SGLT) receptors. Sodium ions are transported down their concentration gradient, but transport is against the glucose gradient
Stage 2: glucose moves from epithelial cell into interstitium. Glucose concentrates within the epithelial cell before crossing the basolateral membrane via facilitative diffusion transporters (GLUTs) and being reabsorbed into interstitial fluid. Meanwhile, sodium moves into the interstitium via the Na+/K+-ATP-ase pump (this process maintains a low Na+ concentration within the cell to allow stage 1 to occur)
Owing to the requirement of co-transport mechanisms, there is a maximum rate of glucose reabsorption, so if the filtered load from the glomerulus exceeds this rate, glucose will be excreted in the urine. This happens at blood glucose levels of approximately 10–12 mmol/l
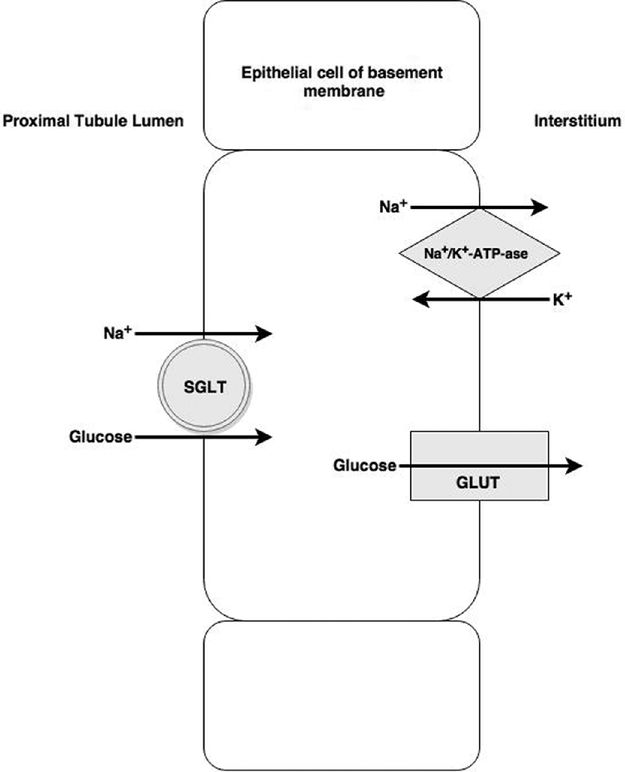
Renal glucose
Because of the requirement of co-transport mechanisms, there is a maximum rate of glucose reabsorption, so if the filtered load from the glomerulus exceeds this rate, glucose will be excreted in the urine. The maximum rate of glucose reabsorption is quoted as a Tm value (Tm means tubular maximum). The Tm for renal uptake of glucose is 380mg/min. When plasma glucose levels exceed 11 mmol/l some proximal nephrons will reach their Tm threshold and glucose will start to be excreted in the urine. As plasma glucose levels rise further, progressively more and more distal nephrons also become reuptake saturated. When plasma levels exceed 22 mmol/l all nephrons are typically operating at Tm and the reabsorption plateaus. All excess glucose is then excreted.
Note: The upstroke of the excreted line and the levelling off of the reabsorption lines are slightly curved rather than sharp inflections – this is because there is some inter-nephron variability in glucose uptake.
Pharmacology
Question 4A
Tell me about the structures of aspirin and paracetamol?
Both drugs are based on an aromatic/benzene ring and are non-specific COX inhibitors
Aspirin is classed as a salicylate. It is an aromatic ester of acetic acid
Paracetamol is a para-aminophenol. It is derived from acetanilide and has a phenol group attached
What are their indications for administration?
Paracetamol is a very commonly administered analgesic. It is also given for its antipyretic actions
In addition to the above two indications, aspirin is also used as an anti-inflammatory agent and for platelet inhibition following myocardial infarction and for cardiac and stroke prevention
Tell me about the contraindications for these two drugs?
Aspirin: should be avoided if known hypersensitivity, active peptic ulceration and haemophilia/bleeding disorders. It should not be given to children under 16 due to risk of Reye’s syndrome (encephalopathic liver failure secondary to mitochondrial damage). Caution should be used in asthmatics (worsens asthma in 10%–20%), those on other anticoagulants and those with renal impairment or dehydration states
Paracetamol: typically very well tolerated. Rare cases of hypersensitivity do occur. Caution to avoid overdosing especially with adult patients <50 kg and in patients with hepatic impairment/failure
What are the mechanisms of action for these two drugs?
Aspirin
Irreversibly inhibits the enzyme cyclo-oxygenase (COX), thus preventing the conversion of arachidonic acid to cyclic endoperoxides
This limits the onward synthesis of thromboxane A2, prostacyclin and prostaglandins (PGE2, PGF2α and PGD2)
Platelets produce thromboxane A2 resulting in local vasoconstriction and platelet aggregation as part of a haemostatic response. Aspirin administration will block thromboxane A2 synthesis via this pathway and result in antiplatelet action
The anti-inflammatory and antipyretic actions are thought to be due to reduced prostaglandin synthesis. At low doses aspirin selectively inhibits the production of thromboxane A2, whilst not affecting prostacyclin. At higher doses, all pathways are inhibited
The mechanism of action of paracetamol is still uncertain
Multiple central pathways are thought to be involved
Prostaglandins: it has been shown that paracetamol has no anti-inflammatory action despite playing a part in the inhibition of COX-mediated prostaglandin production. It is likely that paracetamol indirectly inhibits the activity of the COX enzyme by preventing it entering its oxidised form. This can only occur in areas of low peroxidase levels, such as the brain, and not in areas of inflammation where peroxidase levels are high
Serotonergic pathways: activation of descending central serotonergic pathways plays a key role in paracetamol’s mechanism of action. Its analgesic properties can be partially inhibited by administration of 5-HT3 receptor antagonists (e.g. anti-emetics)
Also thought to have actions modulating cannabinoid, nitric oxide and TNFα systems
Bioavailability describes the fraction of a drug dose that ends up in the systemic circulation when compared to an intravenous dose, which has a bioavailability of 100% as it is injected directly into the circulation. Oral administration of a drug usually gives the lowest bioavailability
Bioavailability can be represented and calculated by the area under a concentration–time curve
Bioavailabilty of an oral drug = AUC Oral∕AUC Intravenous
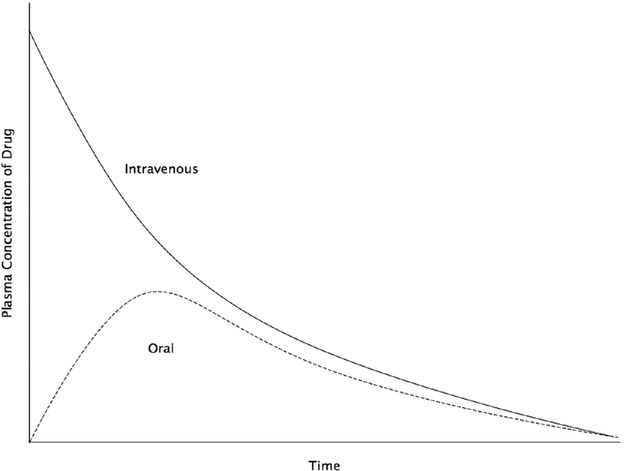
Bioavailability curve
Aspirin
◦ Oral: 70%. Rapid and complete absorption limited by first pass metabolism
◦ Rectal similar but slower absorption
Paracetamol
How are these two drugs metabolised?
Aspirin
Paracetamol
Hepatic metabolism primarily by glucuronidation and sulphation to non-toxic metabolites
A small amount is metabolised by the cytochrome p450 system to form a highly toxic metabolite called N-acetyl-p-benzo-quinone imine (NAPQI)
Normally, NAPQI is metabolised and detoxified by conjugation with glutathione to form mercaptate and cysteine compounds
Both are associated with adverse outcomes in overdose; choose one and tell me about the pathophysiology and management of it in overdose
Overdose can be acute (2% mortality) or chronic (25% mortality)
Toxicity depends on dose ingested, gut absorption may be slowed and peak levels may be delayed. As levels rise the degree of protein binding falls and the usual metabolic pathways in the liver become saturated, leading to zero-order kinetics
Inhibition of thromboxane, prostacyclin and prostaglandins contributes to platelet dysfunction and gastric injury
Stimulation of chemoreceptor trigger zone causes nausea and vomiting
Direct stimulation of the medullary respiratory centre results in an increased minute volume and respiratory alkalosis
Interference with cellular metabolism, particularly oxidative phosphorylation, causes an anion-gap metabolic acidosis largely due to the build up of organic acids such as lactate and ketoacids
Uncoupling of oxidative phosphorylation in mitochondria generates heat causing pyrexia
The acidic environment further exacerbates the process by allowing salicylate to remain unionised, thus able to cross cell membranes and both enter the CNS and be reabsorbed by the kidneys, exacerbating toxicity
Some clinical features of aspirin overdose are given in the following table:
Respiratory |
|
CVS |
|
CNS |
|
Renal |
|
GI |
|
Metabolic |
|
Rarely with severe toxicity |
|
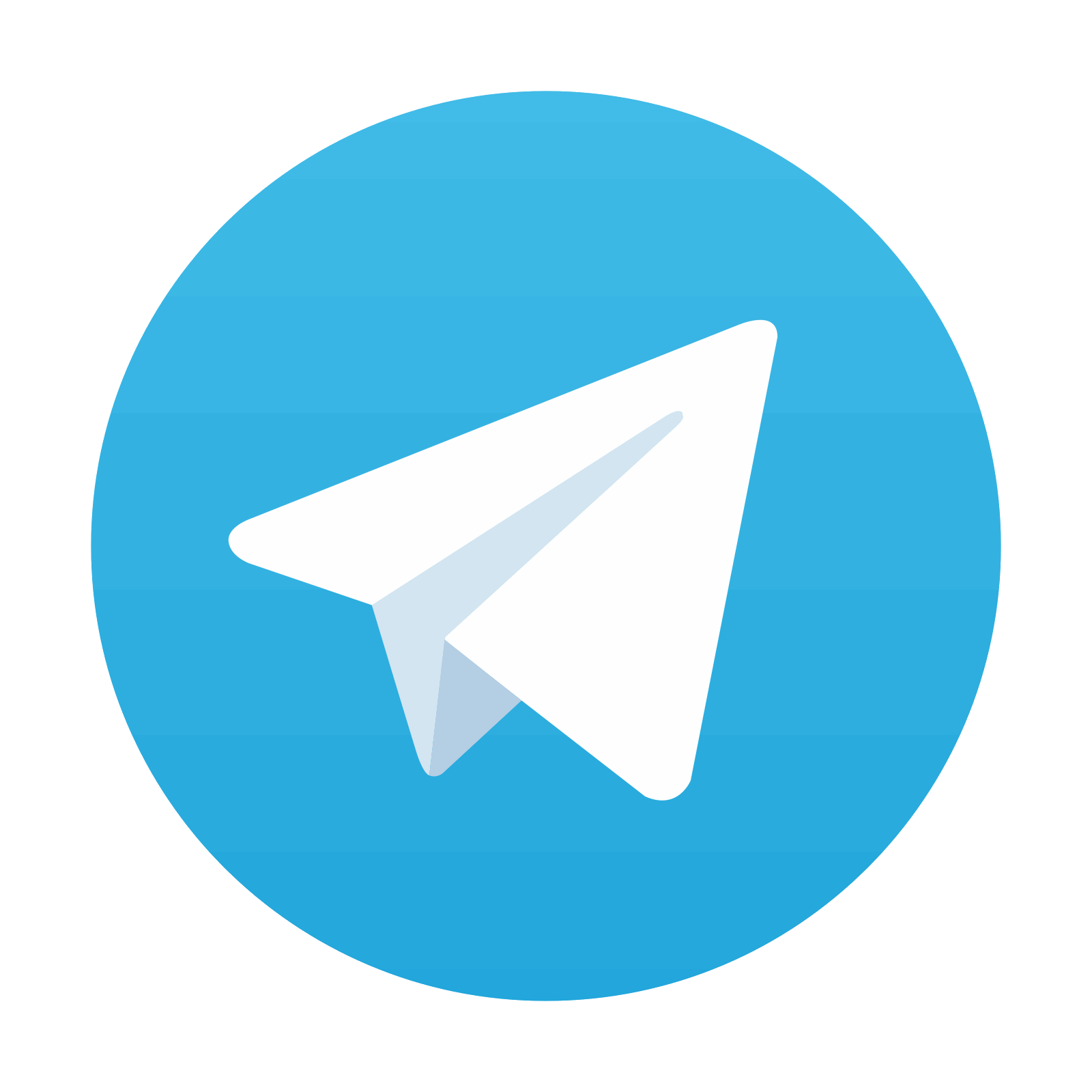
Stay updated, free articles. Join our Telegram channel

Full access? Get Clinical Tree
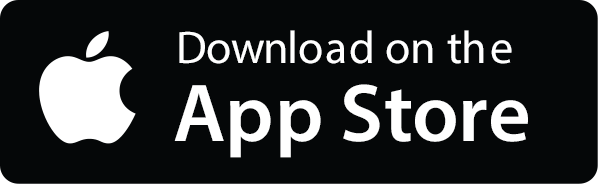
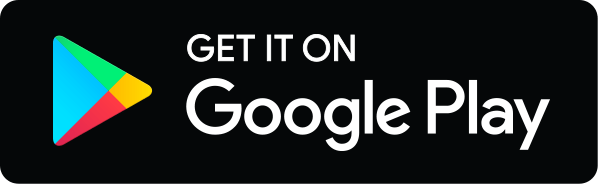