Peripheral nerve blocks provide effective anesthesia and analgesia in a site-specific manner with the potential for long-lasting effects.
Accurate
identification of target nerves and precise and adequate placement of local anesthetic are critical for performing safe and successful peripheral nerve blocks.
Ultrasound imaging has renewed interest in peripheral nerve blocks because it allows visualization of needle movement toward nerve structure(s) in real time, minimizing the risk of needle contact with critical structures and potentially reducing complications. Although highly desirable as an aid to the performance of regional anesthesia, this technology requires considerable training and thorough knowledge of the equipment and cross-sectional regional nerve block anatomy.
Peripheral nerve stimulators are useful tools to facilitate nerve blocks, but they do not eliminate the risk of nerve injury. In the adult patient, maintenance of responsiveness may allow reporting of nerve contact or pain during injection.
Nerve blocks associated with bony or vascular landmarks are more reliable and easy to perform than those dependent on surface landmarks alone.
Larger volumes of local anesthetic may increase the potential success of peripheral nerve blocks, but the total milligram dosage must be limited to avoid systemic toxicity. Higher concentrations of local anesthetics increase the degree of motor block. Ultrasound imaging, through more accurate nerve localization and visualization of local anesthetic spread, may enable successful blocks to be performed with reduced volumes of local anesthetics, but this has yet to be proven.
Multimedia
Neck Anatomy
Brachial Plexus Block
Interscalene Block
Supraclavicular Block
Sensory Innervation of the Hand
TAP Block
Penile Block
Femoral Nerve Block
Ultrasound-Guided Saphenous Nerve Block
Ultrasound-Guided Popliteal Nerve Block
Ankle Block
INTRODUCTION
Regional anesthesia enables site-specific, long-lasting, and effective anesthesia and analgesia. It is suitable for many surgical patients and can improve analgesia,1 reduce morbidity, mortality, and the need for reoperation after major surgical procedures.2 Peripheral nerve blocks (PNB) can be used alone as the sole “surgical” anesthetic; as a supplement to provide analgesia and muscle relaxation together with general anesthesia; or as the initial step in providing prolonged postoperative analgesia, as with brachial plexus blocks or continuous peripheral nerve catheters. Compared to parenteral analgesics, single-shot or continuous PNB can provide superior analgesia and reduce the incidence of side effects.3–5 Optimal pain relief and minimal side effects (e.g., nausea and vomiting) following surgery have a major impact on patient outcome, including patient satisfaction and earlier mobilization, as well as fulfilling the need for streamlined surgical services with lower costs.6 Nevertheless, the safety and success of PNB
techniques are highly dependent on accurate delivery of the correct dose of local anesthetic; even in experienced hands, there is an inherent failure rate associated with regional anesthesia7 with the potential—albeit rare—for systemic toxicity, infection, bleeding, permanent nerve injury, or other physical injury. In addition to the benefits of PNB, advances in knowledge (e.g., physiologic characteristics of solutions during electrical nerve stimulation) and technology (e.g., the introduction of anatomically based ultrasound imaging) have encouraged many anesthesiologists and surgeons to use PNB on a more frequent basis.
Advancements in medical knowledge and techniques are constantly being made and, while new advancements provide an opportunity for improved patient care, they need to be studied and compared to currently accepted techniques to evaluate their safety and utility. In contrast, anatomic structures are static, and an understanding of basic anatomy cannot be replaced by excellent technical skills and knowledge of the technique when performing regional anesthesia. Thus, this chapter provides an in-depth discussion of regional anatomy, while providing an overview of today’s two most up-to-date techniques for nerve localization and block performance: Nerve stimulation (NS) and ultrasound (US) imaging. Specific techniques that are practically useful for the anesthesiologist are described in sections grouped by body location.
GENERAL PRINCIPLES AND EQUIPMENT
Regional anesthesia has long been regarded as an “art” and real success with these techniques was confined to a small number of gifted individuals. The introduction of NS some 30 years ago was the first step toward transforming regional anesthesia into a “science.” This technique relies on physiologic responses of neural structures to electrical impulses. There is considerable interindividual variation in physiologic responses to NS. Furthermore, several other factors influence responses to NS, including injectates, physiologic solutions (e.g., blood), and disease. Despite these limitations, NS was one of the first objective methods available in regional anesthesia to place a needle in close proximity to a target nerve with some reliability. One of the most exciting recent advances in regional anesthesia technology has been the introduction of anatomically based US imaging. This is the first time since the beginning of regional anesthesia practice that the target nerve can actually be visualized. This is a quantum leap in technology for those interested in this pursuit, and the realization of its potential benefits may encourage many anesthesiologists who had previously abandoned these techniques to resume or increase their use of regional anesthesia. However, despite initial excitement over this advancement, US images are subject to individual interpretation depending on experience, training, and where that experience and training was obtained. There is a substantial learning curve associated with US-guided regional anesthesia, and not all practitioners are skilled in interpreting US images. Consequently, in many situations, it is prudent to combine the two technologies of NS and US imaging together in order to achieve the goal of 100% success with all regional blocks. US alone may allow good visualization of the needle and nerve as well as a reasonable estimate of the spread of the full dose of the local anesthetic, yet the correct identity of the nerve may be unknown, especially for novice ultrasonographers.8 By stimulating the nerve, its identity may be objectively determined by observing the motor response to NS.
Patient monitoring and other factors related to optimizing patient care and prevention of complications are similar to those for general anesthesia, with some important differences. Safe and successful performance of PNB requires careful selection of patients and administration of an appropriate type and dose of local anesthetic in the correct location. In addition, the patient must be monitored during the procedure and prior to discharge, and ambulatory patients with home going catheters should be monitored remotely with either telephone follow-up or home health care team visits until the catheter has been removed and the block has completely regressed.
Setup and Monitoring
Setup
Although regional blocks can be performed in the operating room setting just like general anesthesia, it is preferable and desirable to perform these techniques in a designated room or area outside the immediate operating room environment (Fig. 35-1). This is a consequence of what is commonly referred to as “soak time,” which is the time it takes for local anesthetics to cross the cell membrane, block action potentials, and produce either analgesia or surgical anesthesia. The designated area must contain the necessary equipment for safe monitoring and resuscitation but must also contain all of the supplies and equipment to perform common and sophisticated regional block techniques. Some important considerations for this “block room” are described below:
FIGURE 35-1. Designated regional block room with labeled storage cart.
All supplies located in this area must be readily identifiable and accessible to the anesthesiologist.
The area should be of ample size to allow block performance, monitoring, and resuscitation of patients.
There should be equipment for oxygen delivery, emergency airway management and suction, and the area should have sufficient lighting.
A practically organized equipment storage cart (Fig. 35-1) is desirable and should contain all of the necessary equipment (including that required for emergency procedures), supplies, local anesthetics, needles, nerve stimulators, block trays, dressings, and resuscitation drugs. A US machine should also be present.
It is ideal to have a prepared specialty tray that includes items for sterile skin preparation and draping, a marking pen and ruler for landmark identification, needles and syringes for skin infiltration, and specific block needles and catheters.
A selection of sedatives, hypnotics, and intravenous anesthetics should be immediately available to prepare patients for regional anesthesia. These drugs should be titrated to maximize benefits and minimize adverse effects (high therapeutic index); short-acting drugs with a high safety margin are desirable.
Emergency drugs should include atropine, epinephrine, phenylephrine, ephedrine, propofol, midazolam, succinylcholine, and intralipid. In addition, guidelines for resuscitation in the setting of local anesthetic toxicity should be laminated and kept with the intralipid.
Monitoring
When performing regional anesthesia, it is vital to have skilled personnel monitor the patient at all times. At minimum, standard monitoring should include electrocardiogram (ECG), noninvasive blood pressure (NIBP), and pulse oximetry. In addition, the patient’s level of consciousness should be gauged frequently using verbal contact since vasovagal episodes are common during many regional procedures. At present, there are no practical or effective devices that detect rising blood levels of local anesthetic; however, this can be done indirectly by adding pharmacologic markers, such as epinephrine, in appropriate concentrations to the local anesthetics. Close observation for systemic toxicity secondary to rapid intravenous injection (within 2 minutes) as well as delayed (∼20 minutes) absorption is essential. The patient should be monitored for at least 30 minutes following the procedure.
Standard ECG and pulse oximetry are essential monitors when performing regional anesthesia.
Careful monitoring of the patient’s heart rate (along with ECG measurement) is important to detect tachycardia seen with epinephrine when it is included in a test dose. It is also useful as an indicator of systemic toxicity with bupivacaine and other potent local anesthetics.
Before performing blocks with significant sympathetic effects, a baseline blood pressure reading should be obtained. Once the regional anesthesia procedure is complete, the monitors should remain attached. In conscious patients, end-tidal carbon dioxide monitoring is not required; however, there are special nasal prongs available for monitoring patients when this is considered necessary.
At minimum, stable vital signs must be present following regional anesthesia to fulfill discharge criteria from the recovery area. If the block has not begun to regress, appropriate protection for the anesthetized limb and complete instructions should be provided to the patient and their family before discharge. For inpatients, appropriate orders should be written to assure limb protection.
Patients receiving perineural local anesthetic infusions should be visited regularly by a qualified physician postoperatively (i.e., Acute Pain Service) with ongoing documentation of their condition in the medical record.
Common Techniques: Nerve Stimulation and Ultrasound Imaging
Nerve Stimulation
Basics of Technique and Equipment
Electrical stimulation of nerve structures was introduced to regional anesthesia in the middle of the 20th century.9,10 A low-current electrical impulse applied to a peripheral nerve produces stimulation of motor fibers and theoretically identifies proximity to the nerve without actual needle contact or related patient discomfort. When NS techniques are used, it is unnecessary to make actual contact with the nerve (in contrast to the paresthesia method). This theoretically infers that the risk of nerve injury should be less when using NS methods, although this theory has not been proven. Stimulating catheters have recently been introduced and have increased our ability to accurately advance catheters over greater distances along nerve structures.11,12
Using motor responses to NS as a primary nerve localization technique has drawbacks. The main limitations with NS are related to the technique’s inconsistent results13,14 and the variance in electrical properties of different nerve stimulators.15 Many variables affect the ability to stimulate nerves, including conductive area of the electrode (needle or stimulating catheter tip), electrical impedance of the tissues, electrode-to-nerve distance, current flow, and pulse duration.16 Ultimately, the technique relies on the physiologic responses of neural structures to the stimulating current, which is subject to considerable interindividual variation.
Today’s nerve stimulators have features to improve ease-of-use and success, such as maintaining a constant current with adjustable frequency, pulse width, and current intensity (milliamperes; mA). This enables a stable current output (an important safety feature) in the presence of varied resistances from the needle, tissues, and connectors. A clear digital display indicating the actual current delivery is important, as is regular calibration and testing. Some nerve stimulators are equipped with low (up to 6 mA) and high (up to 80 mA) current output ranges. The lower range is primarily for localizing peripheral nerves, while the higher range is mainly used for monitoring neuromuscular blockade. Recently, higher ranges have been utilized for transcutaneous NS techniques17 (2 to 5 mA) including percutaneous electrode guidance18 and surface nerve mapping,11,19 and the epidural stimulation test (1 to 10 mA).20,21 Most nerve stimulators deliver an electrical pulse width of 100 or 200 μs for stimulating motor nerves. Similar to current amplitude, the length of time over which the current is delivered (pulse width) is usually considered important, as shorter duration currents can selectively stimulate motor components of mixed nerves while sparing the discomfort caused by stimulation of sensory components. Some sophisticated devices allow variable pulse widths from 50 μs to 1 ms in an attempt to provide such selective stimulation. The general rule is to use short-duration current of ≤100 μs for peripheral NS, although there is some evidence that duration does not impact patient discomfort22 and that intensity (mA) of the stimulation is perhaps the most important variable.23
Practical Guidelines
During initial advancement of the needle, the nerve stimulator should be set to deliver a current of 1 to 2 mA in order to gauge the approximate distance to the nerve. Depolarization of the nerve can also be improved by using the positive (anode; red) pole of the stimulator as the ground (reference or surface electrode) electrode and the negative (cathode; black) lead as the connection to the needle itself (known as cathodal preference). The actual location of the ground is of little importance with the use of constant-current nerve stimulators.23 Generally, the needle is in close proximity to the nerve when the threshold for motor response is between 0.3 and 0.5 mA; placing the needle to the point where a motor response only requires 0.1 to 0.2 mA may increase the chance of intraneural injection and should be avoided.24 Once a low threshold response is obtained, 2 to 3 mL of local anesthetic is injected and the operator watches for disappearance of the motor twitch, which is a signal to inject the remainder of the proposed dose in divided aliquots. This “Raj test”25 was originally thought to result from physical displacement of the targeted nerve by the injectate, but this response has recently also been attributed to a change in the electrical field at the needle-tissue interface. Electrically conducting solutions (e.g., local anesthetic or saline) reduce the current density at the needle tip, thereby increasing the current threshold for motor response, whereas nonconducting solutions (e.g., dextrose 5% in water; D5W) increase the current density and maintain or augment the twitch response (Fig. 35-2).26
FIGURE 35-2. The current density is localized to the needle tip when using nonconducting solutions (e.g., D5W), thereby maintaining the motor response to the threshold current level during nerve stimulation.
After nerve localization using a stimulating needle, introduction of a stimulating catheter with continuous stimulation of the nerve is suitable to provide continuous analgesia. Similar current thresholds are applicable with the use of stimulating catheters. If an attempt to dilate the perineural space is undertaken, injection of D5W is preferable in order to maintain the motor response to stimulation.27 The reader is referred to the section on Other Related Equipment for optimal features of stimulating catheters.
Ultrasound Imaging
Basics of Technique and Equipment
US imaging is rapidly emerging as a very promising regional anesthesia tool since the size, depth and precise location of many nerves in their surrounding environment can be determined with correct interpretation of the visual image. Visualization of the moving needle, once inserted at an appropriate angle and within the plane of the US probe, as well as the spread of local anesthetic, provides valuable assistance to the anesthesiologist performing regional anesthesia. With US-guided PNB techniques, the operator can adjust needle or catheter placement under direct vision (i.e., US imaging), which may lead to fewer needle attempts and ultimately improved motor and sensory blocks. Furthermore, visibility of vital structures (e.g., blood vessels and pleura) is advantageous in order to avoid complications. Today, technologic advances have led to the development of US systems that can deliver high frequency (10 MHz or higher) sound waves offering the high axial resolution required for visualization of nerves and the ability to distinguish them from the surrounding anatomical structures (e.g., tendons, muscles). The proposed benefits of US guidance, as compared to NS, for upper extremity blocks include improved block success28 and completeness,29 reduced block performance and onset times,28–31 prolonged duration of blocks,30 and reduction in complications.32 While the cumulative evidence may appear convincing, many of the studies show conflicting results for certain parameters, and the large variability in trial methodologies and application of different outcome measures account for many of the discrepancies. Indeed, the various endpoints used during research in regional anesthesia may bias outcomes when comparing multiple regional techniques. Recently, Marhofer et al.33,34 published an excellent review of the current status of US and its use in regional anesthesia. They emphasize that adequate training in US-guided techniques is essential and suggest that education and proper technique can help ensure safe blocks. In addition, current advantages of using US in regional anesthesia, including direct visualization of subcutaneous structures, identification of anatomic variation, ability to use less local anesthetic, and improvement in block quality and patient satisfaction, are also discussed.
US is defined as any sound with a frequency >20 kHz, although medical imaging generally requires between 3 and 15 MHz. Within the body, US scanners emit sound waves that produce an echo when they encounter a tissue interface. Therefore, US images reflect contours, including those of anatomic structures, based on differing acoustic impedances of tissue or fluids. Significant reflection of sound waves occurs at interfaces between substances of different acoustic impedance, resulting in good contour definition between different tissues. High US beam reflection, from high impedance/dense structures (e.g., bone, connective tissue), results in a bright (hyperechoic) image, often with dorsal shadowing underneath; low impedance structures reflect beams to a smaller extent and appear gray (hypoechoic); minimal impedance structures/spaces (e.g., fluid in vessels) appear black (anechoic).
Higher frequencies offer the best spatial resolution at superficial locations (e.g., brachial plexus at supraclavicular fossa), while lower frequencies are often required for structure delineation at deep locations (e.g., sciatic nerve in the subgluteal region). Block location and depth of target nerve structures determine which transducer offers the best imaging and resolution. Several functions of the US system are important to have familiarity with, including field and gain functions as well as Doppler effect. Doppler effect is very useful for identifying blood vessels during nerve localization using US guidance, since many nerves are situated in close proximity to vascular structures.
Practical Guidelines
Both the probe and the skin of the patient should be prepared for maximum sterility and optimal imaging. Probe sterility is paramount if performing real-time, or dynamic, US guidance during block performance. This can be maintained by standard sleeve covers but these can be expensive and cumbersome. For single-shot blocks, it is practical to use a sterile transparent dressing (e.g., Tegaderm; 3M Health Care, St Paul, MN) without the full cover of a sterile sleeve (Fig. 35-3A).35 An issue when using standard long covers is the potential for air to track between the probe and the skin, which reduces image quality. The target area should be surveyed (scanned) using a generous amount of US gel (water soluble conductivity gel is optimal) prior to sterile preparation. One of the most common reasons for poor visualization is lack of sufficient gel for skin-probe contact.
FIGURE 35-3. A: Probe sterility using a sterile transparent dressing (e.g., Tegaderm; 3M Health Care, St Paul, MN) without the full cover of a sterile sleeve. Other dressings may create multiple small wells over the probe surface due to adhesive pockets and lead to poor image quality.36 B: In-plane and out-of-plane needle alignment and subsequent visibility of the needle.
For nerve localization during US-guided PNB, it is helpful to first identify one or more reliable anatomical landmarks (bone or vessel) with a known relationship to the nerve structure. The operator can then localize the nerve at a location near the landmark, and proceed to follow along, or “trace” the nerve to the optimal block location (Table 35-1).36,37 Generally, nerve structures are most visible when the angle of incidence is approximately 90 degrees to the US beam. Obtaining a transverse axis view of the nerve usually allows the best appreciation of the anatomical relationship of the nerve with its surrounding structures. To obtain the best possible view of the shaft and tip of the needle, it is imperative to align the needle shaft to the longitudinal axis (“in-plane”; IP) of the US transducer (probe) (Fig. 35-3B). The nerve structure is often placed at the edge of the US screen to ensure adequate viewing distance for the needle shaft. An alternative approach uses a transverse or tangential (“out-of-plane”; OOP) alignment, which only allows appreciation of the needle in cross section and usually only during movement (Fig. 35-3B). The nerve structure is often placed in the center of the screen to guarantee that aligning the needle puncture with the center of the probe will ensure close needle tip-nerve alignment. This approach can be beneficial in certain block locations (compact areas) and for inserting catheters (e.g., at the subgluteal area), but should never be used in areas where needle tip visibility in relation to vital structures is critical (e.g., supraclavicular fossa near the pleura).
TABLE 35-1. USEFUL ANATOMICAL LANDMARKS FOR LOCALIZING NERVES DURING COMMON ULTRASOUND-GUIDED PERIPHERAL NERVE BLOCKS
After the needle is seen to be close to the nerve(s), a 1- to 2-mL test dose of local anesthetic or D5W can be injected to visualize the spread and perform a “Raj test” if a stimulating needle is being used. The solution will be seen as a hypoechoic expansion and will often illuminate the surrounding area, enabling better visibility of the nerves and block needle. If NS is being used to confirm nerve identity, it is useful to administer D5W in order to maintain accurate motor responses.27 This will be especially important during catheter introduction and advancement. If the test shows undesired injection near or within vessels or cavities, subsequent injection of local anesthetic should be postponed until better needle localization is achieved. If suboptimal spread of injectate is observed, the needle can be repositioned to allow another injection.
There can be a lengthy learning curve for US-guided nerve blocks, and techniques to improve needle and catheter visibility during advancement are important in order to improve training for this technology. Two such approaches have been described experimentally:
The “walk-down” approach to facilitate needle tip identification during OOP needling.37,38 This technique involves calculating the required depth of puncture (with measurement to the desired neural structure recorded using US prior to the block) and using trigonometry with the shaft angle and length to calculate a “reasonable” location to place the initial needle puncture site. The initial shallow puncture will be easily seen as a bright dot on the screen, and the needle tip can be followed as it is “walked down” to the final calculated depth. For example, if the final depth of penetration for the block is 2 cm, the needle will ultimately obtain a 45-degree angle if the initial puncture site is 2 cm from the probe and the needle is incrementally angled to this level.
A method of needle-probe alignment using a laser attachment for the probe has been reported; the laser line will project onto both the needle shaft and the midline of the probe, indicating an IP position.37,39 Aligning the visible optical laser line with the longitudinal axis of the US probe will mimic the “invisible” beam from the US probe and allow improvements with IP needle alignment. With the laser-unit attachment, any misalignment of the needle to the US beam can be easily detected and adjusted in real-time. Recently, commercially available GPS guidance systems intended to guide the needle tip location have been developed, but the merit of these devices remains to be determined.
Other Related Equipment
Needles
Needles used for regional techniques are often modified from standard injection needles. Although reports may speculate that needle design is a determinant of nerve or other tissue injury, there is insufficient evidence to fully substantiate this claim. For PNB, the “short bevel” (i.e., 30 to 45 degrees) or “B bevel” is often used to reduce the potential for injury to nerves.40 Other modifications, such as the “pencil-point” needle, have been introduced in attempts to reduce nerve injury. Single-shot PNB techniques generally require using 22- to 24-gauge insulated needles with short bevels. If superficial and field blocks are performed, smaller gauge (e.g., 25 to 26 gauge) sharp needles can be utilized. Continuous blocks require larger-bore needles to facilitate catheter introduction (e.g., 18-gauge needles for 20-gauge catheters). Blunt-tipped Tuohy needles are commonly used for continuous PNB with success.41 Short-bevel and Tuohy needles offer more resistance and give a better “feel” when traversing different tissues. Desired needle length will depend on each specific block and individual patient characteristics. Clear markings throughout the entire length of the needle are important for measuring depth of penetration, particularly for correspondence to US measurements.
Practical Tips
Techniques and devices have been proposed to limit injection pressure, since there is considerable variation among anesthesiologists in the amount of pressure they apply during injections42 and high-pressure injections into the nerve (especially intrafascicular) have been associated with damage in animals.43,44 Disposable, in-line injection pressure monitors are available, although their ability to prevent long-term injury is not well documented. Alternatively, a compressed air injection technique (CAIT) has been described to limit the generation of excessive pressure during injection. With this method, air is drawn into the syringe and compressed by 50% during the entire injection to maintain pressures of approximately 760 mm Hg (Boyle’s law: Pressure × volume = constant).45
Catheters
Continuous-infusion catheter kits suitable for PNB are available that include a standard polyamide catheter, such as those previously used for epidural analgesia, combined with an insulated Tuohy needle with NS capability. Recently, catheters have been advanced to the point of making them amenable to stimulation (an electrode is placed into the catheter tip). This may enable more accurate advancement of catheters for substantial distances to provide continuous analgesia. Some studies have suggested that it may be helpful to inject a solution to dilate the perineural compartment to facilitate the advancement of catheter. The reader is referred to the discussion of practical guidelines of NS in the section Common Techniques: Nerve Stimulation and Ultrasound Imaging for discussion of injection solutions for perineural dilation. There are a number of continuous-infusion devices now available for both inpatient and outpatient use, which allow delivery of dilute local anesthetic concentrations for as long as 72 hours after surgery. Standard precautions are required to maintain sterility of the catheter and the insertion site, but complications have been rare with these techniques and new devices.
Avoiding Complications
In general, regional anesthesia has an excellent safety record. Complication rates are as low as 8 per 10,000 for seizures2 and <0.1% to 1% for nerve injury,7,46 and only rare cases of severe chronic pain syndromes following regional anesthesia have been reported.47 Nevertheless, the incidence of some complications is often higher in PNB than other regional anesthesia/analgesia techniques, and results can be devastating. Choosing a suitable patient and applying the right dose of local anesthetic in the correct location are the primary considerations. Careful attention to any unusual responses or report of pain during block performance as well as follow-up prior to and after discharge is equally important, although often overlooked.
Patient Selection
Patient selection is a critical element for the performance of safe and effective PNB. Not all patients are suitable candidates for PNB. In general, patients scheduled for extremity, thoracic, abdominal, or perineal surgery should be considered potential candidates for peripheral regional anesthetic techniques. Adamant refusal of regional anesthesia by a patient is a contraindication to the procedure.
Other contraindications include local infection, systemic anticoagulation, and severe systemic coagulopathy. In most cases, schizophrenic patients should only receive regional techniques if general anesthesia is also performed. The presence of pre-existing neurologic disease is a controversial topic and, while a limited amount of data is available in the case of spinal anesthesia, the safety of PNB is unclear. One must be cognizant of the potential to compound existing neurologic deficit; therefore, clear documentation of the deficits prior to the procedure and a careful discussion of the potential risks and benefits are critical. For every clinical situation, the use of regional anesthesia must be carefully evaluated as a matter of risk versus benefit. It is imperative to follow applicable national and international guidelines, such as those set by the American Society of Anesthesiologists (ASA) for patient monitoring and those in place for anticoagulated patients, as provided by the American Society of Regional Anesthesia and Pain Medicine (ASRA).
Local Anesthetic Drug Selection, Toxicity, and Doses
This section will provide an overview of drug selection and toxicity during PNB. For a more detailed discussion of the pharmacology and toxicity of local anesthetics, the reader is referred to Chapter 21.
Rates of systemic and local toxicity and nerve injury with PNB are generally low, but the use of available methods to reduce inadvertent intravascular and intraneural injections is clearly warranted. It is important to note that lower concentrations of local anesthetic (e.g., 1% to 1.5% lidocaine, 0.125% to 0.5% bupivacaine) compared to those used for epidural anesthesia are appropriate for peripheral nerves. Neural toxicity of these anesthetics appears to be concentration dependent.48 The use of highly concentrated solutions may be useful to increase motor block, but increases the total milligram dose of local anesthetic. To limit total drug dose, lower concentrations are usually indicated when larger volumes are required to anesthetize poorly localized peripheral nerves or to block a series of nerves. Nevertheless, there is no clinical evidence that prolonged exposure (as with continuous PNB) of nerves to local anesthetic solutions of appropriate concentration predisposes to neurotoxic injury.49
Systemic toxicity is most often related to accidental intravascular injection, and rarely to the administration of an excessive quantity of local anesthetic to an appropriate site. The risk of systemic toxic reactions is often related to the drug used. Ropivacaine (generally at 0.5%) is a recent example of a drug introduced into clinical practice in order to reduce central nervous system and cardiovascular toxicity through its physiochemical and stereoselective properties.50,51 Despite this, there are examples of ropivacaine toxicity during PNB.52–55 One strategy to potentially reduce the volume and concentration of local anesthetic solution required to produce a successful block is the use of US imaging to more accurately position the needle in close proximity to the nerve and to visualize the spread of solution to ensure adequate exposure.56,57 Of greatest importance is the ability to avoid intravascular injection. This risk may be reduced when using US, especially if combined with color Doppler for vessel localization.
The degree of systemic drug absorption and the duration of anesthesia can also vary depending on the site of injection (i.e., level of vascularization) and addition of vasoconstrictors. The highest blood levels of local anesthetic occur after intercostal blocks, followed by caudal, epidural, brachial plexus, intravenous regional, and lower extremity blocks. Equivalent doses of local anesthetic may produce only 3 to 4 hours of anesthesia when placed in the epidural space, but 12 to 14 hours in the arm, and 24 to 36 hours when injected along the sciatic nerve. Many believe that the addition of epinephrine (1:200,000 to 1:400,000) is advantageous in prolonging the duration of block and in reducing systemic blood levels of local anesthetic, although this has more relevance to local anesthetics like lidocaine and less to ones like bupivacaine. Its use is not appropriate in the vicinity of “terminal” blood vessels, such as in the digits, penis, or ear or when using an intravenous regional technique. Using significant quantities of local anesthetic during PNB should not be performed unless oxygen, suction, and appropriate resuscitation equipment is immediately available. However, even small doses of local anesthetic may produce significant side effects when injected into susceptible regions such as the neck. When performing PNB, a test dose of an epinephrine-containing solution and small incremental injections are recommended to reduce the risk of unrecognized intravascular injection. Toxicity can also occur from peripheral absorption of excessive doses of local anesthetic. Patients should be observed carefully for at least 30 minutes following injection because peak blood levels may occur at this time.
Animal studies58 and recent case reports59,60 have shown successful resuscitation from local anesthetic toxicity by intravenous administration of intralipid (20% lipid; not the 10% lipid of propofol), using one or more boluses (each of 1 to 2 mL/kg or 100 mL) followed by a 30-minute infusion (0.5 mL/kg/min). It is important to use this strategy as an acute resuscitation agent, only after standard measures have proven ineffective.
Nerve Damage and Other Complications
Peripheral nerve injury in humans may result from intraneural injection61,62 or direct needle trauma,63 although there are other causes, including those related to the surgical procedures (e.g., patient positioning, proximity of nerve to surgical site, and tourniquet application).64 Needle-related trauma without injection may result in injury of lesser magnitude than that from injection injury.65 In animal studies, nerve injury appears to occur when high injection pressures are applied intrafascicularly and particularly when highly concentrated local anesthetic solutions or their preservatives are used.43,44,66 One major sequela from intrafascicular injection is endoneural ischemia.67 While in some cases these syndromes resolve uneventfully, full recovery of some peripheral injuries may never occur or may require several months, a result of slow regeneration of injured peripheral nerves.61
Other minor complications that have been reported following PNB include pain at the site of injection and local hematoma formation, but these are self-limited side effects and are best dealt with by communication with the patient and reassurance by the anesthesiologist. A hematoma around a peripheral nerve is not of the same significance or risk as that occurring in the epidural or subarachnoid space. It is important to address concerns expressed by patients and to make every effort to relieve any pain or discomfort resulting from various interventions.
Discharge Criteria
Stable vital signs must be present in order to fulfill criteria for discharge from the recovery area. In some cases, acceptable evidence of regressing sensory and motor blocks should be present. However, if a long-lasting local anesthetic was used to perform the block or a continuous catheter with an infusion of local anesthetic is present, the block may not show evidence of regression at the time of discharge. Postoperative follow-up is important to confirm that neurologic function has returned to normal. If a deficit is suspected, early neurologic assessment is critical to determine the appropriate course of management.
Patients should have well-controlled pain upon discharge. Incorporating a standard level of pain relief (e.g., on a verbal rating scale) prior to discharge home or to the ward is prudent. Specific common risks for certain blocks should be discussed with the patient prior to discharge. When discharging patients from postanesthesia care units while an extremity is still anesthetized (e.g., the block was performed to provide extended analgesia), it is absolutely necessary to provide in-depth instruction related to the risks and their prevention (e.g., risk of burns to anesthetized areas will require avoidance of certain forms of cooking; potential for developing pressure neuropathies). A clear understanding of the information provided is important for both the patient and their caregivers. Written instructions including expected course, common side effects, and 24-hour contact information should be provided.
Premedication and Sedation
The best preparation for a regional technique is careful patient selection and ensuring that the patient is adequately educated and informed about the anesthetic and surgical procedures. Supplemental medication is often helpful. Appropriate sedation and analgesia is an essential part of successful regional anesthesia in order to produce maximum benefit with minimal side effects. Effective sedation can be achieved with a variety of medications, including but not limited to propofol, midazolam, fentanyl, ketamine, remifentanil, alfentanil, or a combination of these drugs. The dosages should be titrated to reach an appropriate level of sedation for the individual patient, specific nerve block procedure and length of surgery. Some examples are listed below.
Bolus
Midazolam 1 to 2 mg (titrated up to 0.07 mg/kg)
Fentanyl 0.5 to 1 μg/kg
Alfentanil 7 to 10 μg/kg
Ketamine 0.1 to 0.5 mg/kg
In addition to the general comments about premedication discussed in earlier chapters, regional anesthesia techniques have special requirements. Sedation must be adjusted to the required level of patient cooperation. In the case of elicitation of a paresthesia (as during several blocks in the head and neck region) or electrical stimulation techniques, the level of sedation must be sufficient to allow the patient to identify and report nerve contact. Although a low dose of opioid (50 to 100 μg of fentanyl or equivalent) will help ease the discomfort of nerve localization, patient responsiveness must be maintained. This does not preclude the use of an amnestic agent; small doses of propofol or midazolam may provide excellent amnesia while maintaining levels of consciousness that still allow cooperation.
CLINICAL ANATOMY
Anatomical descriptions of major nerve structures, including plexuses and terminal/peripheral nerves are discussed in this section. The section is divided on the basis of regions of the body and includes the head and neck, spine, upper extremity, trunk, and lower extremity.
Head and Neck
Trigeminal Nerve
Sensory and motor innervation of the face is provided by the branches of the fifth cranial (trigeminal) nerve. The roots of this nerve arise from the base of the pons and send sensory branches to the large semilunar (trigeminal or Gasserian) ganglion, which lies on the dorsal surface of the petrous bone. Its anterior margin gives rise to three main branches: The ophthalmic, maxillary, and mandibular nerves (Fig. 35-4). A smaller motor fiber nucleus lies behind the main trigeminal ganglion and sends motor branches to one terminal nerve, the mandibular. The three major branches of the trigeminal nerve each have a separate exit from the skull:
FIGURE 35-4. Major branches of the trigeminal nerve. The roots of this nerve arise from the pons and form the large Gasserian (or semilunar) ganglion. The three major branches have separate exits from the skull. The main terminal fibers of the ophthalmic nerve—the frontal nerve—terminate as the supraorbital and supratrochlear nerves and exit their respective foramen. The maxillary and mandibular branches emerge from the skull medial to the lateral pterygoid plate. The maxillary nerve terminates as the infraorbital nerve (through such named foramen), and the mandibular nerve provides the inferior alveolar nerve (as well as motor branches), which exits at the mental foramen as the mental nerve.
The uppermost ophthalmic branch passes through the sphenoidal fissure into the orbit. The main terminal fibers of this sensory nerve, the frontal nerve, run to behind the center of the orbital cavity and bifurcate into the supratrochlear and supraorbital nerves. The supratrochlear branch traverses the orbit along the superior border and exits on the front of the face in the easily palpated supraorbital notch; the supraorbital nerve runs in a medial direction toward the trochlea (Fig. 35-5).
FIGURE 35-5. Lateral view of the surface of the head, showing the cutaneous innervation of the superficial/distal trigeminal nerve branches to the face and the anatomy and block needle insertion angles to perform a superficial cervical plexus block. The needle is initially inserted perpendicular to the skin at the midpoint of the lateral border of the sternocleidomastoid muscle (where it is crossed by the external jugular vein). Subsequently, the needle can be inserted in superior and inferior angulations to reach the entire cervical plexus. SO, supraoribtal nerve; ST, supratrochlear nerve; IO, infraorbital nerve; M, mental nerve.
The maxillary nerve contains only sensory fibers. It exits the skull through the round foramen (foramen rotundum), passes beneath the skull anteriorly, and enters the sphenopalatine fossa. At this point, it lies medial to the lateral pterygoid plate on each side. At the anterior end of this channel, it again moves superiorly to re-enter the skull in the infraorbital canal in the floor of the orbit. It branches to form the zygomatic nerve, which extends to the orbit, the short sphenopalatine (pterygopalatine) nerves, and the posterior dental branches. The anterior dental nerves arise from the main trunk as it passes through the infraorbital canal. The terminal infraorbital nerve penetrates through the inferior orbital fissure to the base of the orbit, to the infraorbital groove and canal (just below the eye and lateral to the nose), and reaches the facial surface of the maxilla. It then divides into the palpebral (lower eyelid), nasal (wing of the nose), and labial nerves (upper lip).
The mandibular nerve is the third and largest branch of the trigeminal, and the only one to receive motor fibers. It exits the skull posterior to the maxillary nerve through the oval foramen (foramen ovale), forms a short thick trunk, and then divides into an anterior trunk, mainly motor, and a posterior trunk, which is mostly sensory. The main branch (posterior trunk) continues as the inferior alveolar nerve medial to the ramus of the mandible and innervates the molar and premolar teeth. This nerve curves anteriorly to follow the mandible and exits as a terminal branch (mental nerve) through the mental foramen. The mental nerve provides sensation to the lower lip and chin. Other terminal nerves include the lingual nerve (floor of mouth and anterior two-thirds of tongue) and the auriculotemporal nerve (ear and temple).
Cervical Plexus
Sensory and motor fibers of the neck and posterior scalp arise from the anterior rami (branches) of the first four cervical (C1–C4) spinal nerves. The cervical plexus is unique in that it divides early into cutaneous branches (penetrating the cervical fascia) (Fig. 35-6) and muscular branches (deeper branches that innervate the muscles and joints), which can be blocked separately (Fig. 35-7). The dermatomes of the cervical nerves C2–C4 are illustrated in Figure 35-8.
FIGURE 35-6. Schematic of the cervical plexus, which arises from the anterior primary rami of C2–C4. The motor branches (including the phrenic nerve) curl anteriorly around the anterior scalene muscle and travel caudally and medially to supply the deep muscles of the neck. The sensory branches exit at the lateral border of the sternocleidomastoid muscle to supply the skin of the neck and the shoulder.
FIGURE 35-8. The cervical, thoracic, lumbar and sacral dermatomes of the body.
FIGURE 35-7. Needle insertion points and angles for the deep cervical plexus blockade. The nerve roots exit the vertebral column via the troughs formed by the transverse processes. The needle is inserted to make contact with the articular pillars of C2–C4 using a caudad and posterior direction.
Classic cervical plexus anesthesia along the tubercles of the vertebral body produces both motor and sensory blockade. The transverse processes of the cervical vertebrae form peculiar elongated troughs for the emergence of their nerve roots. These troughs lie immediately lateral to a medial opening for the cephalad passage of the vertebral artery (Fig. 35-7). The trough at the terminal end of the transverse process divides into an anterior and a posterior tubercle, which can often be easily palpated.
These tubercles also serve as the attachments for the anterior and middle scalene muscles, which form a compartment for the cervical plexus as well as the brachial plexus immediately below. The compartment at this level is less developed than the one formed around the brachial plexus.
The deep muscular branches curl anteriorly around the lateral border of the anterior scalene and then proceed caudally and medially. Many branches serve the deep anterior neck muscles, but other branches include the inferior descending cervical nerve, the trapezius branch of the plexus, and the phrenic nerve, which give anterior branches to the sternocleidomastoid muscle as they pass behind it.
The sensory fibers emerge behind the anterior scalene muscle but separate from the motor branches and continue laterally to emerge superficially under the posterior border of the sternocleidomastoid muscle. The branches, including the lesser occipital nerve, great auricular nerve, transverse cervical nerve, and the supraclavicular nerves (anterior, medial, and posterior branches), innervate the anterior and posterior skin of the neck and shoulder.
Occipital Nerve
The ophthalmic branch of the trigeminal nerve provides sensory innervation to the forehead and anterior scalp; the remainder of the scalp is innervated by fibers of the greater and lesser occipital nerves (Fig. 35-9).
FIGURE 35-9. Greater and lesser occipital nerve distributions, supply, and block needle insertion sites.
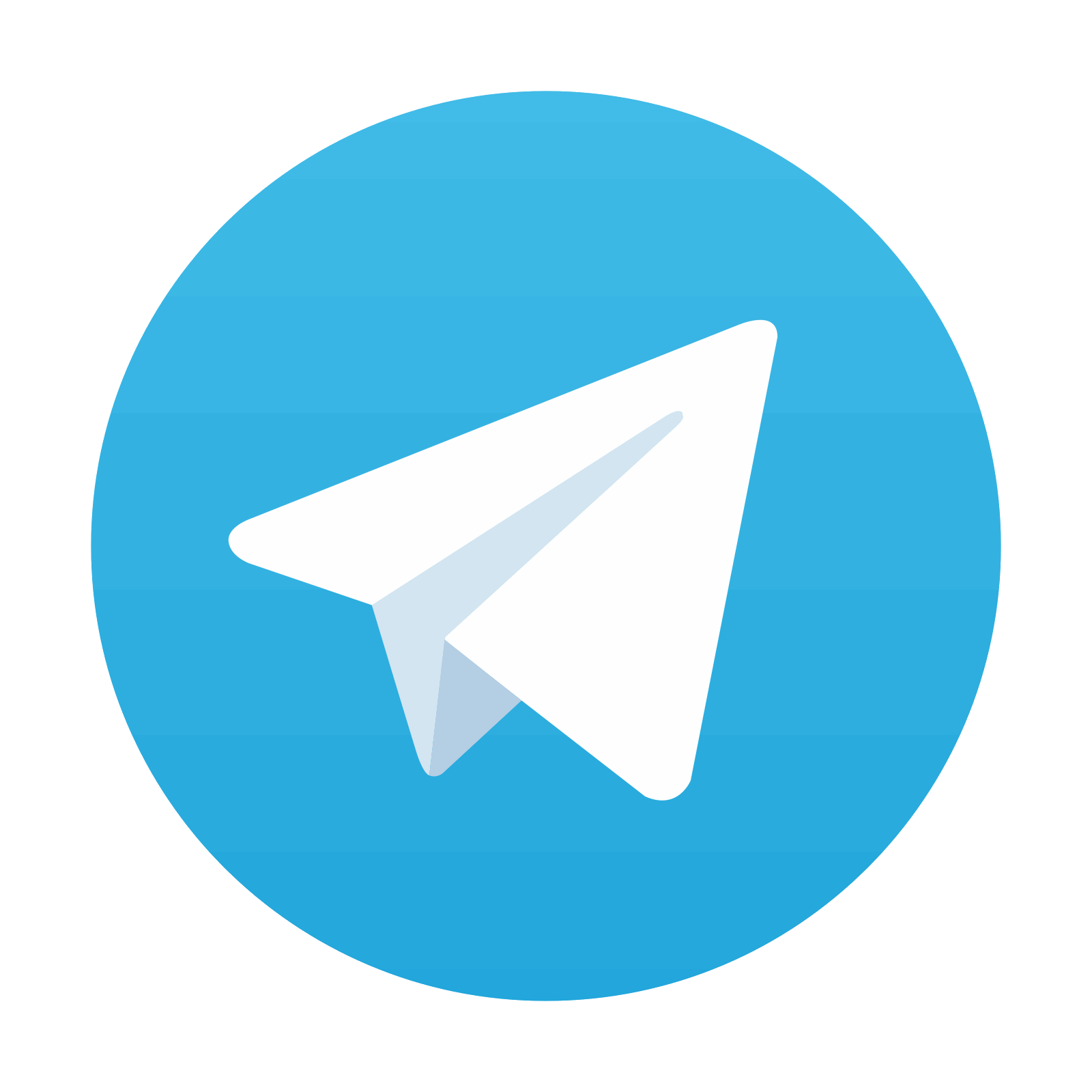
Stay updated, free articles. Join our Telegram channel

Full access? Get Clinical Tree
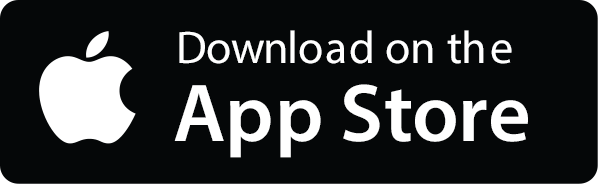
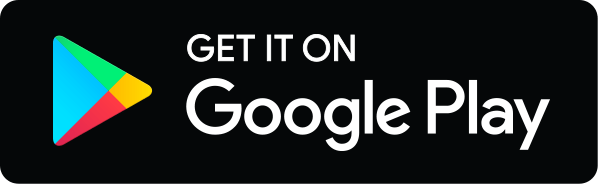