Mark D. Twite1 and Robert H. Friesen2 1 Congenital Cardiac Anesthesiology, Children’s Hospital Colorado, University of Colorado School of Medicine, Denver, CO, USA 2 Department of Anesthesiology, Children’s Hospital Colorado, University of Colorado School of Medicine, Denver, CO, USA Pulmonary hypertension (PH) has many different etiologies, in which all share the final common path of elevated pulmonary arterial pressure (PAP). After birth, PAP falls, reaching adult levels within a few months. In normal, healthy individuals, mean pulmonary artery pressure (mPAP) at rest is around 15 mmHg and is independent of age, ethnicity, and gender. During exercise, mPAP increases and is dependent on the level of exertion and age. During mild exercise, mPAP is 20 ± 5 mmHg in subjects under age 50 years compared to 30 ± 5 mmHg in subjects older than age 50 years, which makes it difficult to define normal mPAP during exercise, and hence, the definition of PH uses mPAP at rest [1]. Initially, PH was arbitrarily defined as an mPAP > 25 mmHg. However, this definition has recently changed, as two standard deviations above a normal mPAP of 15 mmHg would suggest an mPAP > 20 mmHg as the upper limit of normal (above the 97.5th percentile). However, this abnormal elevation of mPAP is not sufficient to define pulmonary vascular disease, as it can be due to an increase in cardiac output or pulmonary arterial wedge pressure. Therefore, the 6th World Symposium on Pulmonary Hypertension (WSPH) in 2018 proposed to include pulmonary vascular resistance (PVR) ≥3 Wood Units (WU) in the definition of all forms of precapillary PH associated with an mPAP > 20 mmHg [2]. The current hemodynamic definitions of PH are shown in Table 33.1. The Pediatric Task Force of the sixth WSPH has chosen to follow this new adult definition of PH to maintain a common universal language and to facilitate transition from pediatric to adult care. This Pediatric Task Force further reinforced the need to index PVR to body surface area in children [3]. PVR index (PVRI) relates the absolute value of PVR to the patient’s body surface area to account for the effect of body size on blood flow and it should be reported as WU m2 [4]. An mPAP > 20 mmHg does not carry any implication of the presence or absence of pulmonary hypertensive vascular disease. PVR is important in the diagnosis and management of pulmonary hypertensive vascular disease in patients with congenital heart disease (CHD). PH complicates the course of many children and adults with CHD. The increase in PAP associated with CHD is secondary to either increased “precapillary” pulmonary blood flow (left‐to‐right shunt lesion) or increased postcapillary pressures (left heart disease). Based on the hemodynamic definition of PH (mPAP > 20 mmHg), almost all patients with a large unrestricted left‐to‐right shunt will have PH. What is important in this setting is the degree of pulmonary hypertensive vascular disease resulting in an increase in PVR. A patient with a high pulmonary blood flow and low PVR will have PH but can be safely treated with the closure of the shunt. On the contrary, a patient with low pulmonary blood flow, shunt reversal (right to left), cyanosis, and high PVR, the so‐called Eisenmenger syndrome, will be harmed with shunt closure due to worsening right heart failure, as there is no longer a “pop‐off” for blood flow [5]. Eisenmenger syndrome is an end stage, and usually irreversible, because of PH with a PVRI > 10 WU m2 with reversed or bidirectional shunting of blood at an aortopulmonary, ventricular, or atrial level. However, between these two extremes of PH and associated CHD is a challenging gray zone that requires careful diagnostic evaluation to guide future management. Table 33.1 Hemodynamic definitions of pulmonary hypertension (PH) Source: Simonneau et al. [2]. Reproduced with permission of European Respiratory Society. mPAP, mean pulmonary arterial pressure; PAWP, pulmonary arterial wedge pressure; PVR, pulmonary vascular resistance; WU, Wood Units. a Clinical groups – see Table 33.2. Since inception in 1973, the World Health Organization (WHO) group classification system for PH has provided researchers and clinicians an evolving framework from which to investigate, diagnose, and treat patients with a diverse collection of pulmonary vascular diseases [2, 6]. The clinical classification of PH has five categories based on shared pathological mechanisms, hemodynamic characteristics, and management strategies (Table 33.2). Subsequent iterations of this clinical classification have maintained a comparable structure with changes paralleling scientific advancement. The definitions are accepted worldwide and used daily in practice by clinicians as inclusion/exclusion criteria for clinical research and in the labeling of new therapeutics for the treatment of PH. The WHO groups 1–5 classification system for children is problematic, as it does not reflect the complex heterogeneity of factors that contribute to pediatric PH. As a result, the Pulmonary Vascular Research Institute Pediatric Taskforce proposed a new classification of pediatric PH at its meeting in Panama in 2011 [7]. The classification has 10 categories based on clinical pediatric practice and reflects the heterogeneous nature of pediatric PH (Table 33.6). The Panama classification includes an additional definition of PH for children with univentricular circulations: following a cavopulmonary anastomosis, PH is defined as a PVRI≥ 3 WU m2 or a transpulmonary gradient (mPAP − left atrial pressure) >6 mmHg even if the mPAP is <20 mmHg (Box 33.1) [7, 8]. In this setting of nonpulsatile blood flow to the pulmonary arteries, even though mPAP may not be greater than 20 mmHg, significant pulmonary hypertensive vascular disease can lead to a poor outcome. With the agreement of the Pediatric Taskforce, some of these items were included in the most recent 2018 Pulmonary Hypertension Classification to have a more comprehensive classification, inclusive of both adults and children [9]. Children with CHD and PH are in group 1, pulmonary arterial hypertension (PAH). Group 1.4.4 PAH includes patients with simple operable and inoperable CHD, subgrouped as those with Eisenmenger physiology, those with PAH and left‐to‐right shunts, those with PAH thought to be incidental to their CHD, and those with postoperative defects. Transient PH following repair of CHD occurs in 21.9 cases per million and is one of the commonest forms of PAH in children, second only to persistent PH of the newborn [10]. Complex heart diseases have been assigned to group 5.4. Group 2, PH due to left heart disease, includes congenital and acquired left heart inflow and outflow tract obstruction, such as pulmonary vein stenosis, cor triatriatum, mitral stenosis, and cardiomyopathies. This group causes the “postcapillary” increase in PAP. In group 3, PH due to lung disease and/or hypoxia, developmental lung diseases are emphasized due to the important role of abnormal lung vascular growth in the pathogenesis of PH. Examples include congenital diaphragmatic hernia, bronchopulmonary dysplasia (BPD), alveolar capillary dysplasia, and surfactant protein abnormalities. Group 4, PH due to pulmonary artery obstructions, and group 5, PH with unclear and/or multifactorial mechanisms, including complex CHD, are rare causes of pediatric PH. The characterization of PH according to these classifications is an essential part of the diagnostic workup of PH. The treatment strategy and prognosis of pediatric PH largely depend on its subclass and associated conditions. It is, therefore, important to correctly characterize the type of PH using standardized diagnostic workup (Figure 33.1). However, in pediatric PH, there is often more than one associated condition identified, which leaves it to the clinician to determine the contribution of each condition to the PH and to decide on which condition therapy should be focused. However, there is now a shift away from this historic classification paradigm to a genotype–phenotype model, which potentially removes the boundaries between the current groups [11]. The guidelines for the diagnosis and management of pediatric and adult PH have comparable themes, but recommendations for treatment in children are based largely on expert consensus and extrapolation from adult data [12]. Identifying parallels in the genotype–phenotype profile among adult and pediatric patients with comparable genetic mutations may simplify investigation and treatment. Armed with specific knowledge about genotype–phenotype of their patient, clinicians may be better equipped to provide precision care regardless of patient age. The large‐scale PVDOMICS study (Redefining Pulmonary Hypertension through Pulmonary Vascular Disease Phenomics) is utilizing the principle of phenomics (e.g. genomics, transcriptomics, proteomics, metabolomics) to create a rich data resource from which to redefine PH classification at the molecular level [13, 14]. The major etiologies of pediatric PH cases are idiopathic PAH (IPAH), heritable PAH (HPAH), and PAH associated with CHD (PAH‐CHD). Gene mutations involved in the transforming growth factor‐β (TGF‐β) signaling pathway, primarily within bone morphogenetic protein receptor type‐2 (BMPR‐2), have been shown to be important in IPAH and HPAH. There are other mutations, which are also thought to be important in the pathogenesis of PAH, including activin‐like kinase‐1 (ALK‐1), T‐box 4, SOX17, and endoglin mutations [15, 16]. BMPR‐2 is the most common gene mutation associated with pediatric PAH and can be identified in 10–20% of pediatric patients with IPAH/HPAH [10, 17, 18]. Table 33.2 Updated clinical classification of pulmonary hypertension Source: Adapted from Simonneau et al. [2]. LVEF, left ventricular ejection fraction; PAH, pulmonary arterial hypertension, PCH: pulmonary capillary hemangiomatosis; PVOD, pulmonary veno‐occlusive disease. Table 33.3 Updated classification of drugs and toxins associated with PAH Source: Simonneau et al. [2]. Reproduced with permission of European Respiratory Society. Table 33.4 Definitions of acute and long‐term response Source: Simonneau et al. [2]. Reproduced with permission of European Respiratory Society. CCB, calcium channel blocker; mPAP, mean pulmonary artery pressure; NYHA, New York Heart Association; PAH, pulmonary arterial hypertension. a Nitric oxide (10–20 ppm) is recommended for performing vasoreactivity testing, but IV epoprostenol, IV adenosine, or inhaled iloprost can be used as alternatives. Table 33.5 Signs evocative of venous and capillary (pulmonary veno‐occlusive disease/pulmonary capillary hemangiomatosis) involvement Source: Simonneau et al. [2]. Reproduced with permission of European Respiratory Society. DLCO, diffusing capacity of the lung for carbon monoxide; PAH, pulmonary arterial hypertension. Table 33.6 The Panama classification: 10 categories of pediatric pulmonary hypertensive vascular disease Source: Cerro et al. [7]. Reproduced with permission of John Wiley & Sons. Two large registries have provided further insights into the etiology of pediatric PH. The Tracking Outcomes and Practice in Pediatric (TOPP) PH registry reported 362 patients with confirmed PH [19]. Of these patients, 317 (88%) had PAH, of which 57% were characterized as IPAH or HPAH and 36% as PAH‐CHD. Only three patients had chronic thromboembolic PH. Chromosomal anomalies, mainly trisomy 21, or syndromes were reported in 47 (13%) of the patients. Another large registry for pediatric PH is from the Netherlands. This registry reports 2,845 (87%) of 3,263 pediatric patients with PAH in group 1 of the PH classification, including transient PAH (82%) or progressive PAH (5%) [10]. The most common cause of transient PAH was Persistent Pulmonary Hypertension of the Newborn (PPHN) (58%), and the most common cause of progressive PAH was PAH‐CHD (72%). Trisomy 21 was the most frequent chromosomal abnormality (12%), a rate similar to that observed in the TOPP registry. Figure 33.1 Diagnostic algorithm for pulmonary hypertension (PH) in children. 6MWT, 6‐minutes walk test; AVT, acute vasodilator testing; CHD, congenital heart disease; CPET, cardiopulmonary exercise test; CT, computed tomography; CTA, CT angiography; CTD, connective tissue disease; CTEPH, chronic thromboembolic PH; DLCO, diffusing capacity of the lung for carbon monoxide; IPAH/FPAH, idiopathic/familial pulmonary arterial hypertension, mPAP, mean pulmonary arterial pressure; MRI, magnetic resonance imaging; PA, pulmonary artery; PAWP, pulmonary arterial wedge pressure; PCH, pulmonary capillary haemangiomatosis; PEA, pulmonary endarterectomy; PFT, pulmonary function test; PVOD, pulmonary veno‐occlusive disease; PVRI, pulmonary vascular resistance index; RV, right ventricular; V/Q, ventilation/perfusion; WU, Wood Units. (Source: Rosenzweig et al. [3]. Reproduced with permission of European Respiratory Society.) PH is a rare disease. The incidence and prevalence of PH in the pediatric population are not well known. In the Netherlands’ registry, the annual incidence of IPAH and PAH‐CHD were 0.7 and 2.2 cases/million, respectively. The prevalence of PAH‐CHD was 15.6 cases/million [10]. The national registry from the United Kingdom reports similar numbers with an annual incidence of IPAH of 0.48 cases/million children and a prevalence of 2.1 cases/million [20]. In a multicenter study in France, the estimated prevalence of PAH was 3.7 cases/million children, with IPAH (60%) and PAH‐CHD (24%) being the main etiologies [21]. Survival for patients with PH has improved with the introduction of management guidelines, dedicated centers, and new drug therapies. Before targeted drug therapies were available for PH, the estimated median survival of children and adults with IPAH were similar, 4.12 and 3.12 years, respectively [22]. After the introduction of targeted pulmonary vasodilators, the survival rate for children with PAH has continued to improve. In the combined pediatric and adult US REVEAL (Registry to Evaluate Early and Long‐Term PAH Disease Management) registry, patients with childhood onset PAH had 1‐, 3‐, and 5‐year survival rates of 96, 84, and 74%, respectively [23]. There was no significant difference in 5‐year survival between IPAH/HPAH (75%) and PAH‐CHD (71%). Similar data have been published from studies in the Netherlands and United Kingdom [20, 24, 25]. Although overall survival has improved, patients with repaired CHD and pulmonary hypertensive vascular disease have a high mortality risk. PH associated with CHD is classified in group 1, PAH. This group distinguishes itself from the other four categories by its characteristic pattern of vascular remodeling, progressive nature, and response to specific medical therapy. Heath and Edwards published the first description of pulmonary vascular remodeling due to CHD in 1958 [26]. They divided the histological progression from grades 1 to 6 in increasing severity. Later, Rabinovitch developed the morphometric approach, which quantifies the thickness of the pulmonary arterial muscle, the degree of abnormal distal extension of smooth muscle, and the density of small pulmonary arteries relative to the number of alveoli [27]. The morphometric structural findings are graded from A through C in increasing severity of changes. This morphometric analysis has contributed to our understanding of pulmonary vascular remodeling by establishing that abnormal extension of smooth muscle and a reduction of small artery density are a hallmark of pulmonary hypertensive vascular disease. This approach has emphasized that early age to correct congenital heart defects helps preserve small pulmonary arteries and predicts good pulmonary hemodynamics postoperatively. The pulmonary vascular remodeling that is characteristic for PAH involves adventitial thickening, medial hypertrophy, and intimal proliferation, including the formation of plexiform lesions [28]. This vascular remodeling leads to arterial wall thickening and occlusion of small distal pulmonary arteries. Together with other mechanisms including vasoconstriction, inflammation, and thrombosis, the vascular wall thickening and occlusion will consequently increase PAP and PVR (Figure 33.2) [29]. This increase in right ventricular (RV) afterload increases RV workload, eventually resulting in RV failure. The pulmonary vascular bed plays a key role in the presentation and management of children with almost all forms of CHD. Four variables are important in the development of pulmonary hypertensive vascular disease and whether it will regress or progress after repair of a congenital cardiac shunt lesion: The evolution of PAH‐CHD and the age at which it presents itself depends on the type of shunt lesion. In patients with a shunt at the pretricuspid valve level (e.g. ASD), there is an increased pulmonary volume load with a normal pressure load. Only 5–20% of these patients will develop severe PAH, and usually not until the third or fourth decade of life [30, 31]. In patients with nonrestrictive shunts at the posttricuspid valve level, the increased pulmonary volume is accompanied by an increased pressure load due to pressure equalization across the shunt defect. In these patients, PAH usually develops more rapidly during the first few years of life. If left uncorrected, these systemic to pulmonary shunts will result in steadily increasing PVR, and when this exceeds the systemic vascular resistance (SVR), the shunt will reverse resulting in hypoxemia and cyanosis, which are the hallmarks of Eisenmenger syndrome [5]. For this reason, most CHD shunt lesions should be repaired within the first 2 years of life. The exact level of PVRI that precludes safe closure of a defect is often debated and varies with each lesion. The WSPH proposed criteria for shunt closure in patients with net left‐to‐right shunting (Table 33.7) [32]. Complex congenital heart defects, such as transposition of the great arteries, truncus arteriosus, and aortopulmonary window, present with pulmonary hypertensive vascular disease much earlier and should, therefore, be repaired in the first weeks of life. Patient genetic and environmental factors may influence the timing of repair of congenital heart defects with PAH. Pediatric PAH occurs in 20–40% of children with genetic disorders, including trisomy 21, Noonan, Velocardiofacial, and Jacobsen syndromes [18]. Furthermore, many children with PAH have clinical signs of a syndrome, such as dysmorphic features, which cannot be explained by a known syndrome diagnosis or genetic test. The frequent occurrence of genetic abnormalities in children with PAH suggests the existence of still unknown genetic pathways involved in the disease process of PAH. The BMPR‐2 mutation has been described in 6% of children with CHD [33]. Comorbidities may also exacerbate PAH‐CHD. For example, children with trisomy 21 are at higher risk of developing PH due to sleep‐disordered breathing, upper airway obstruction, silent aspiration with recurrent pneumonias, and pulmonary hypoplasia [34]. The net result is episodes of hypoxia, which in turn may exacerbate the pulmonary vascular disease from CHD. In developing countries, there may be additional comorbidities to consider. In Africa, in a cohort of children with CHD, 53% were anemic, 47% underweight, and 33% had marasmus compared to a control group without CHD, of whom none had marasmus and only 14% were underweight [35]. Tuberculosis is twice as common in patients with CHD, especially in those with lesions with an increased pulmonary blood flow, and if treatment is available, the major cause of morbidity is delay of cardiac surgery [36]. Unfortunately, tuberculosis may mask the signs of CHD and result in a delay in diagnosis of CHD and subsequent surgical repair. Human immunodeficiency virus (HIV) infection is endemic in sub‐Saharan Africa. In Uganda, CHD affects 5% of children with HIV compared to 3% in developed countries [37]. More recently, coronavirus disease 2019 (COVID‐19) has had a catastrophic impact on global health. The virus responsible for the pandemic is severe acute respiratory syndrome coronavirus 2 (SARS‐CoV‐2), and the most frequently reported comorbidities in children who become critically ill with the virus are chronic lung disease, obesity, neurodevelopmental disabilities, and CHD. Patients with PH are vulnerable to SARS‐CoV‐2, as it is a respiratory virus that causes PH via multiple pathways including lung inflammation and fibrosis, vasoconstriction, and thrombosis [38]. Environmental factors may also influence the timing of surgical repair of congenital heart defects. High altitude has effects on normal neonatal pulmonary vascular transition that is related not only to altitude but also to ancestry. Ethnic groups with recent migration to high altitude have lower oxygen saturations and higher PAP than those with a long ancestry of high altitude living, such as native residents of the Andes mountain range and Tibet. In addition, the incidence of CHD increases at higher altitude, the ductus arteriosus is larger, and its closure delayed, with a higher pulmonary to systemic blood flow ratio for a given PVR. As a result, management decisions, particularly the timing of surgical repair, are different at high altitude [39, 40]. Figure 33.2 Pulmonary vascular changes in pulmonary arterial hypertension includes infiltrating adaptive and innate immune cells. In the top panel is a representative histopathology of a vessel with severe neointimal formation represented below by a diagrammatic illustration. The histopathology shows a single endothelial layer and an eccentric neointima (pale pink) that contains cells that have markers of inflammatory cells and others that stain with markers of smooth muscle but appear poorly differentiated. The medial muscular layer is expanded, and there is an abundant adventitial layer. This vessel is decorated with complement and autoantibodies, infiltrated by neutrophils in the lumen attacking the vessel wall and other inflammatory cells binding to the endothelium and infiltrating. The neointima is composed of pale cells and matrix and infiltrating T and B cells, and in the adventitia, there are dendritic cells, macrophages, and mast cells, and in the periadventitial space tertiary lymphoid follicles characterized by T cells, B cells, and plasmacytoid dendritic cells (APC). NK indicates natural killer; PH, pulmonary hypertension; and SMC, smooth muscle cell. (Source: Rabinovitch et al. [29]. Reproduced with permission of Wolters Kluwer Health, Inc.) Table 33.7 Guidance for assessing operability in pulmonary arterial hypertension associated with congenital heart disease Source: Rosenzweig et al. [3]. Reproduced with permission of European Respiratory Society. Special considerations include age of patient, type of defect, and comorbidities, resting or exercise‐induced desaturation is a concern and PAH therapy (treat with intent‐to‐repair approach has not been proven). The clinical assessment of the child with PAH and CHD is aimed at differentiating those with reversible pulmonary vascular disease who are operable from those with irreversible or fixed disease. In cases that are not straightforward, it is important to put together all the clinical information without relying on one single parameter. The clinical presentation of pediatric PH is nonspecific and age‐dependent. The most common presenting symptom is reduced exercise capacity accompanied by fatigue and dyspnea. Young infants may present with feeding problems and failure to thrive. Other presenting symptoms include cyanosis, cough, and chest or abdominal pain. Syncope as a presenting symptom is specific to pediatric PH and is reported in 25–30% of children with PH and is most frequent in patients without a shunt defect [18–20]. There is often a long delay between the onset of symptoms and diagnosis due to the low specificity of presenting symptoms and the rarity of the disease. Screening should take place for children belonging to high‐risk groups based on underlying conditions of family members with PH. The functional status of children and adults with PH, with respect to exercise capacity and symptoms, can be classified using the WHO Functional Classification (WHO‐FC) [41]. The WHO‐FC scale is based on the New York Heart Association (NYHA) classification, which evaluates symptoms including limitation of physical activity, dyspnea, fatigue, chest pain, and right heart failure. Although the WHO functional class is not designed specifically for children, it has been shown to correlate with 6‐minute walk distance and hemodynamic parameters [21, 23, 24, 42]. Another scale has been developed for children, the age‐adjusted Panama Functional Classification (Panama‐FC) [43]. In children, both the WHO‐FC and Panama‐FC scales have been shown to correlate well with PH disease progression. The Panama‐FC appears to be better for long‐term follow‐up, while the WHO‐FC appears to be better for short‐term monitoring, for example, after changes to treatment [44]. After a thorough history and physical examination of the patient, blood should be drawn for laboratory analysis including complete blood count, metabolic panel, coagulation profile, and biomarkers of PH. Biomarkers that specifically indicate the pathologic mechanism, the severity of the disease, and the treatment response would be ideal tools for the management of PH, but they are still the subjects of research. Anemia, hypocarbia, elevated uric acid, and C‐reactive protein levels are nonspecific markers of disease severity. Brain natriuretic peptide (BNP) and N‐terminal fragment of pro‐BNP, which reflect the degree of atrial stretch and heart failure, are recommended for disease monitoring [45]. Noninvasive imaging for the assessment of PH includes chest X‐ray, echocardiography, CT scan of the lungs, nuclear medicine ventilation perfusion scan, and magnetic resonance imaging (MRI) [46]. By the time the diagnosis of PH is made, 90% of patients have an abnormal chest X‐ray, although sensitivity and specificity are low [47]. Typical features on chest X‐ray include elevated cardiac apex due to RV hypertrophy, enlarged right atrium, prominent pulmonary outflow tract, enlarged pulmonary arteries, and pruning of peripheral pulmonary arteries. Echocardiography provides information about the anatomic details of the lesion, assessment of shunt direction, and estimates of pulmonary artery pressures (PAPs) [48]. Echocardiography may support the diagnosis of PH with qualitative images of elevated RV pressure, such as RV hypertrophy and septal wall flattening. Quantitative information may be obtained on echocardiography if there is tricuspid regurgitation during systole (Figure 33.3). In this case, the modified Bernoulli equation may be applied to estimate PAP with a tricuspid regurgitant velocity of greater than 2.8 m/s being highly indicative of PH (Box 33.2) [1, 49]. Measurement of the end‐systolic RV to left ventricular (LV) diameter ratio correlates with invasive measurements of the severity of PH [50]. Echocardiography can provide information on the direction of flow through a shunt. A pure left‐to‐right shunt across a defect indicates that the PVR is lower than the SVR. The presence of reversed or bidirectional flow across an ASD, PDA, or VSD is concerning and suggests the need for cardiac catheterization and acute vasoreactivity testing. Transthoracic echocardiography is an attractive method to assess children with PH and possibly enable the cardiologists to lengthen the interval between cardiac catheterizations required to monitor ongoing therapy. There are many echocardiographic techniques in the research and validation phase. One technique is to monitor the RV systolic to diastolic duration ratio, in which an increase has been shown to be associated with worse RV function, exercise capability, and survival [51]. Another technique using echocardiography is to measure the degree of tricuspid annular plane systolic excursion (TAPSE), which has been shown to reflect RV function and prognosis in PH [52, 53].
CHAPTER 33
Anesthesia for Pulmonary Hypertension
Incidence, anatomy, natural history
Definition
Definitions
Characteristics
Clinical groups a
Precapillary PH
mPAP > 20 mmHg
PAWP ≤ 15 mmHg
PVR≥ 3 WU
1, 3, 4, and 5
Isolated postcapillary PH
mPAP > 20 mmHg
PAWP > 15 mmHg
PVR < 3 WU
2 and 5
Combined pre‐ and postcapillary PH
mPAP > 20 mmHg
PAWP > 15 mmHg
PVR≥ 3 WU
2 and 5
Classification
Epidemiology
Renal carcinoma
Uterine carcinoma
Germ cell tumors of the testis
Other tumors
Uterine leiomyoma
Hydatidosis
Chronic hemolytic anemia
Myeloproliferative disorders
Pulmonary Langerhans cell histiocytosis
Gaucher disease
Glycogen storage disease
Neurofibromatosis
Sarcoidosis
Chronic renal failure with or without hemodialysis
Fibrosing mediastinitis
Definite
Possible
Aminorex
Cocaine
Fenfluramine
Phenylpropanolamine
Dexfenfluramine
L‐Tryptophan
Benfluorex
St. John’s wort
Methamphetamines
Amphetamines
Dasatinib
Interferon α and β
Toxic rapeseed oil
Alkylating agents
Bosutinib
Direct‐acting antiviral agents against hepatitis C virus
Leflunomide
Indirubin (Chinese herb Qing‐Dai)
Acute pulmonary vasoreactivitya for patients with idiopathic, hereditable, or drug‐induced PAH
Reduction in mPAP ≥ 10 mmHg to reach an absolute value of mPAP ≤ 40 mmHg.
Increased or unchanged cardiac output
Long‐term response to CCBs
NYHA Functional Class I/II
With sustained hemodynamic improvement (same or better than achieved in the acute test) after at least 1 year on CCBs only
Pulmonary function tests
Decreased DLCO (frequently <50%)
Severe hypoxemia
Chest high‐resolution CT
Centrilobular ground‐glass opacities/nodules
Mediastinal lymph node enlargement
Response to PAH therapy
Possible pulmonary edema
Genetic background
Biallelic EIF2AK4 mutations
Occupational exposure
Organic solvent (trichloroethylene)
Category
Description
1
Prenatal or developmental pulmonary hypertensive vascular disease
2
Perinatal pulmonary vascular maladaption
3
Pediatric cardiovascular disease
4
Bronchopulmonary dysplasia
5
Isolated pediatric pulmonary hypertensive vascular disease
6
Multifactorial pulmonary hypertensive vascular disease in congenital malformation syndromes
7
Pediatric lung disease
8
Pediatric thromboembolic disease
9
Pediatric hypobaric hypoxic exposure
10
Pediatric pulmonary vascular disease associated with other system disorders
Pathophysiology of pulmonary hypertension in congenital heart disease
PVRI wood units (m2)
PVR wood units
Correctability/favorable long‐term outcome
<4
<2.3
Yes
>8
>4.6
No
4–8
2.3–4.6
Individual patient evaluation in tertiary center
Assessment of pulmonary hypertension
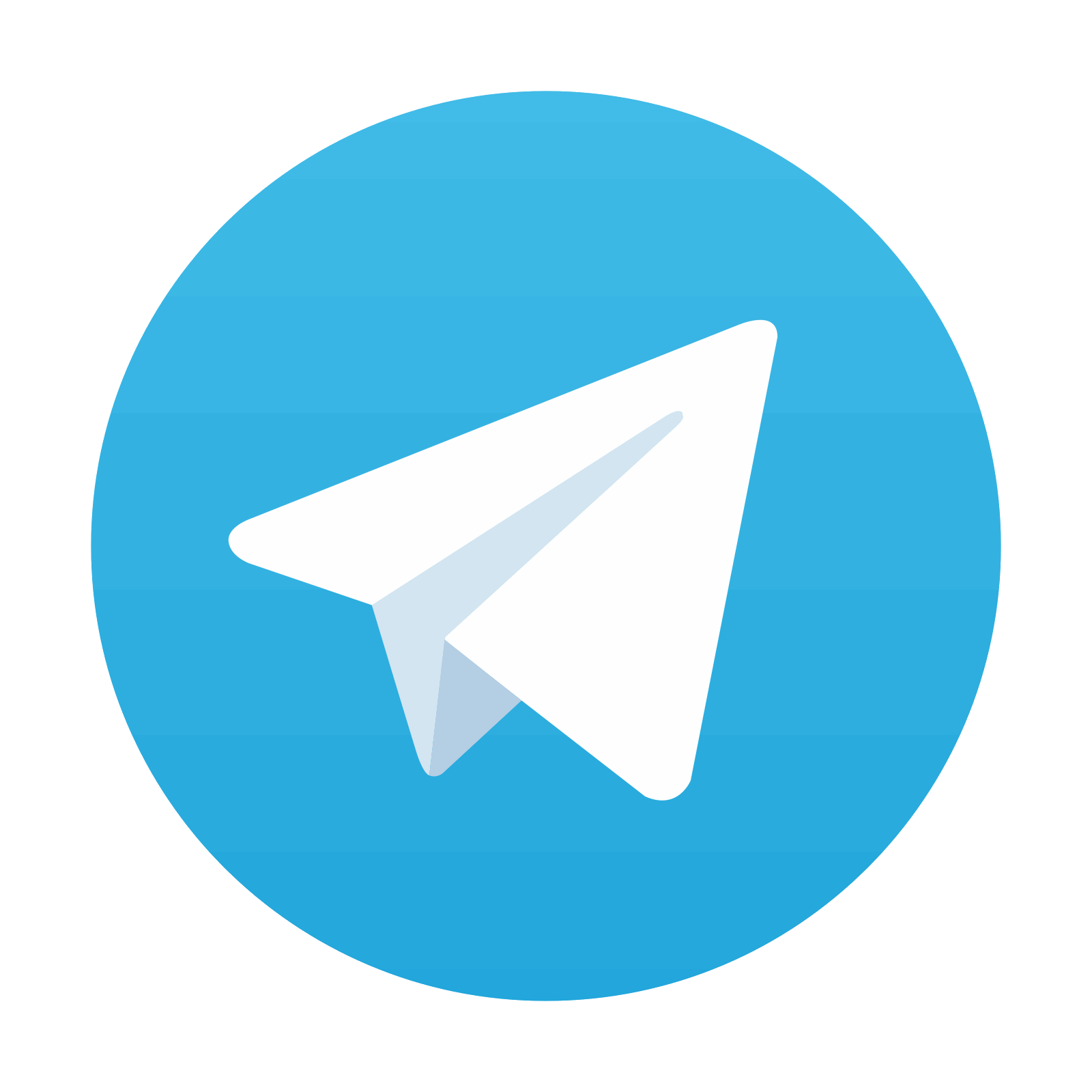
Stay updated, free articles. Join our Telegram channel

Full access? Get Clinical Tree
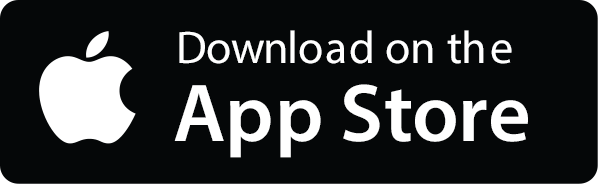
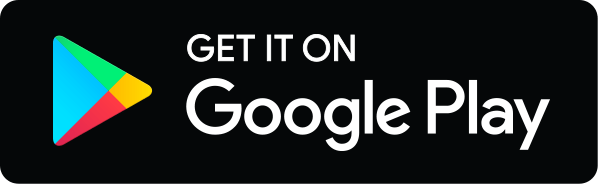