Jane Heggie1, Catherine Ashes2, Andrea Girnius3, and Pablo Motta4 1 Medical Director Cardiovascular Intensive Care Unit, Peter Munk Cardiac Center, Staff Cardiovascular Anaesthesiologist, Toronto General Hospital, University of Toronto, Toronto, Ontario, Canada 2 Consultant Anesthetist, St. Vincent’s Hospital, Sydney, New South Wales, Australia 3 Assistant Professor of Clinical Anesthesiology, Division Director, Obstetric Anesthesia, University of Cincinnati Medical Center, Program Director, Obstetric Anesthesia Fellowship, University of Cincinnati College of Medicine, Cincinnati, OH, USA 4 Staff Cardiovascular Anesthesiologist Service Lead, Adult Congenital Cardiac Anesthesiology, Arthur S. Keats Division of Pediatric Cardiovascular Anesthesiology, Texas Children’s Hospital, Associate Professor of Anesthesiology, Department of Anesthesiology, Baylor College of Medicine, Houston, TX, USA The adult congenital heart disease (ACHD) population is in constant growth due to improvements in diagnosis and treatment. In the last 70 years, the survival rate improved five times from 15% in the early 50s to more than 90% in the current era [1, 2]. In a comprehensive prevalence study in the province of Quebec, the median age of all individuals with severe congenital heart disease (CHD) in 1985 was 11 years and increased to 17 years in 2000. At that time, the ACHD population exceeded for the first time the children living with severe CHD. Currently, the ratio continues to rise in favor of the adult populations [3]. Worldwide the estimated prevalence of CHD population has also increased by 18% from 1990 to 2017 [4]. This increase is mostly due to increased survival rate in middle to higher income countries since the prevalence rates of CHD at birth has changed modestly. The are several challenges facing the anesthesiologist taking care of patients with ACHD. First, the ACHD population requires anesthesia often to treat residual cardiac defects, arrhythmias, and heart failure since most of these patients have sequelae from their initial repair or palliation or the natural progression of the CHD. Second, access to medical information and previous surgical intervention is challenging since the transition of care from pediatric to adult service is not seamless [5, 6]. Finally, ACHD patients with moderate‐to‐severe disease by anatomic and or physiological criteria have an increased perioperative risk in cardiac and in noncardiac surgery [7, 8]. Risk stratification is key to optimize high risks patients perioperatively and define the best setting for treatment and recovery [9]. The perioperative risk of patients with ACHD is related to the anatomical complexity of CHD, physiologic status and acquired comorbidities. The 2018 American Heart Association (AHA)/American College of Cardiology (ACC) Guideline for the Management of ACHD adds the patient’s present physiological condition to the classic anatomical classification of CHD [10]. Traditional severe anatomical conditions include cyanotic CHD (unrepaired or palliated), double‐outlet ventricle, interrupted aortic arch, mitral atresia, single ventricle, pulmonary atresia, transposition of the great arteries (TGA), and truncus arteriosus. From the physiological standpoint, higher risk patients include those in symptomatic heart failure (New York Heart Association Functional Class III–IV), severe aortic enlargement (diameter ≥ 5.0 cm), arrhythmias refractory to treatment, severe hypoxemia (saturation at rest <85%), and severe pulmonary hypertension (systemic or supra‐systemic, Eisenmenger syndrome) and/or refractory end‐organ dysfunction. Certainly, acquired comorbidities affect patients with ACHD more commonly than the general population. These include metabolic syndrome (central obesity, dyslipidemia, hypertension, and diabetes mellitus), renal dysfunction, liver failure, restrictive lung disease, developmental delays, and acquired neurological injury [11–15]. Despite a higher incidence of metabolic syndrome coronary artery disease prevalence is similar to the overall population. Restrictive lung disease is not only related to CHD but also to the number of thoracotomies [16]. There is no well‐defined pathway to treat patients with ACHD. The treatment options are two, adult hospitals or children’s hospitals without a clear best choice. Adult hospitals carry the expertise of treating adult patients with acquired disease but lack the CHD knowledge. Alternatively, children’s hospitals have the skills to treat CHD, but they do not have the experience to treat adult‐acquired disease [17]. Independently of the type of facility successful ACHD centers use a team approach with a wide variety of providers including pediatric, and ACHD cardiologists, surgeons, and pediatric cardiovascular anesthesiologists. Currently, in the United States, there are only 41 Adult Congenital Heart Association (ACHA) accredited programs in a total of 25 states to treat over a million patients. ACHA accredited programs have better outcomes than those without that expertise. ACHD programs need to develop a transitional program to decrease the attrition to follow‐up. A comprehensive survey of patients with ACHD in North America identified that 42% of patients (mean age 19 ± 9.1 years) had gaps in the care of more than 3 years. This survey excluded adults cognitively incapable of responding directly to the survey and probably underestimates the gaps in care. The most common reasons cited for gaps in care were “feeling well,” “changing or losing insurance,” and “financial problems” for severe cardiac lesions. Many patients with ACHD perceive themselves as normal individuals who have had a heart problem in the past that is “fixed,” and fail to appreciate the need for follow‐up [18]. The goals of this chapter are to analyze the noncardiac sequelae of CHD and review the ACHD pregnancy consideration and the more prevalent cardiac lesions. Finally, the chapter will address the ACHD heart failure management (e.g., ventricular assist device and transplantation) and the management of the patient with ACHD for cardiac and noncardiac surgery. Pediatric patients who undergo cardiothoracic operations are at increased risk of developing scoliosis [19]. The described risk factors include female gender, developmental delay, and presence of genetic syndrome (e.g., Down’s or DiGeorge’s). Traditionally thoracotomy has been described as a risk factor for developing scoliosis but newer rib sparing surgical approaches had challenged this concept [20]. The mechanism of this predisposition is probably multifactorial including surgical injury, diaphragmatic palsy, chest remodeling due to cardiomegaly, and asymmetric growth post‐thoracotomy or sternotomy. Consequently, restrictive lung function is prevalent in surgically repaired CHD patients, and up 50% of these patients have restrictive spirometry testing profiles [21]. Patients with ACHD have decreased exercise tolerance based on oxygen consumption (VO2) index, diminished forced expiratory volume in 1 second (FEV1) and forced vital capacity (FVC), and significant restriction compared with normal (Figure 20.1) [22]. A diminished pulmonary function may have consequences postoperatively either in the recovery room or intensive care unit (ICU), and the ability to wean from the ventilator and needs to be addressed in the preoperative visit [23]. Moderate‐to‐severe impairment of FVC is a predictor of mortality in ACHD [24]. Figure 20.2 shows a representative patient with severe pulmonary sequelae. Pulmonary arterial hypertension (PAH) is a major perioperative risk factor in ACHD and is defined as an increase in mean pulmonary arterial pressure (PAPm) ≥25 mmHg at rest as assessed by right heart catheterization [25, 26]. PAH associated with ACHD can be classified into four groups: The anesthetic management of PH and weaning from cardiopulmonary bypass must be tailored to avoid vasoconstrictive triggers, minimizing PVR by optimal oxygenation, alkaline pH, and the use of the pulmonary vasodilators (e.g., nitric oxide, NO). In ICU, DTT should be started before attempting to wean ventilatory support and iNO. The DTT includes three pathophysiological pathways: the endothelin pathway (endothelin‐1 receptor antagonists), NO pathway (phosphodiesterase type‐5 inhibitors), and prostacyclin pathway (prostanoids). Bosentan an endothelin‐1 receptor antagonist has shown long‐term symptomatic benefits in exercise capacity in ACHD patients with PH [27]. Phosphodiesterase type 5 inhibitors besides the vasodilatory effect in pulmonary vasculature have a beneficial effect at a microcirculatory level in patients with ACHD [28]. Iloprost, a prostanoid, produces pulmonary vasodilation through the prostacyclin pathway, improves right ventricular function and exercise capacity in Eisenmenger patients [29]. Figure 20.1 Spirometry profiles in adults with congenital heart disease. Abbreviations: FEV1, forced expiratory volume in 1 second; FVC, forced vital capacity. ASO, arterial switch operation; ccTGA, congenitally corrected transposition of the great arteries; Coarc, coarctation of the aorta; TOF, tetralogy of Fallot; LVOTO, left ventricular outflow tract obstruction; PVS, pulmonary valve stenosis; ASD, atrial septal defect; VSD, ventricular septal defect; AVSD, atrioventricular septal defect. (Source: Ginde et al [22]. Reproduced with permission of John Wiley & Sons) Eisenmenger syndrome is a challenge, as the anesthesiologist must deal with both PH and the potential to exacerbate the right‐to‐left shunt, resulting in worsening cyanosis. It is more likely to present in patients with large and complex septal defects. The median survival after diagnosis of Eisenmenger syndrome is reported to be 35–60 years [30]. Patients with Eisenmenger syndrome will present for heart‐lung transplantation, but outside of this context, interaction with an anesthesiologist will be limited to diagnostic investigations and noncardiac surgery. Generally, Eisenmenger patients are easier to manage than patients with primary PH, as the right ventricle (RV) is robust and accustomed to a high afterload. Bennet et al. showed that hypotension is common during induction of Eisenmenger’s patients regardless of the agent used. The use of preemptive use vasopressor is recommended [31–33]. Manes et al. presented the survival data of ACHD patient with PH. The patients with small or corrected defects have the worse outcomes [34]. Chapter 33 contains an extensive discussion of PAH. Patients with cyanotic heart disease have erythrocytosis, an increase in the red cell line in response to hypoxia. Relative to the red cells there is a deficiency in vitamin K‐dependent clotting factors, fibrinogen, and platelets [35]. Particularly single ventricles or cyanotic patients have an increased risk of thrombosis yet are frequently coagulopathic intraoperatively [36, 37]. Phlebotomy is inappropriate unless there are extreme symptoms of hyperviscosity, as iron deficiency with microcytosis is an independent risk factor for stroke, thrombosis, and hemoptysis [38]. Figure 20.2 26‐year‐old with pulmonary atresia and right ventricle (RV) to pulmonary artery (PA) conduit. The patient’s weight is 45 kg, and the previous history includes pneumonectomy at age 16 years, paralyzed vocal cord, asthma, bronchiectasis, kyphoscoliosis. Forced expiratory volume in 1 second (FEV1) is 600 mL (20% of normal). The patient has RV–PA conduit obstruction with an RV pressure of 48/25 mmHg, and elevated pulmonary vascular resistance at 8–10 Wood units. Impaired renal function, as in patients with acquired heart disease, is a significant predictor of morbidity and length of stay in ACHD surgery [39]. Kwiatkowski et al. in a retrospective study described the principal risk factors for developing acute kidney injury after ACHD surgery [40]. Older age (≥35 years), preoperative left ventricular (LV) dysfunction, preoperative arrhythmia, longer bypass time, and vancomycin use were significant risk factors. The Acute Dialysis Quality Initiative Group has defined the range of renal dysfunction using the so‐called “RIFLE classification system” (Box 20.1), which has been endorsed by the Multi‐societal Database Committee for Pediatric and Congenital Heart Disease and further subdivided into renal insufficiency and renal dysfunction [41]. A total of 1,102 adult patients attending the Royal Brompton Hospital in London over a period between 1999 and 2006 had a serum creatinine measured and a glomerular filtration rate calculated. Renal dysfunction was mild in 41% of patients and moderate or severe in 9%. Not surprisingly, severe renal impairment correlated with increased mortality over the 4‐year follow‐up period [42]. Surgical, perfusion and interventional strategies allow early intervention in the neonatal period, with improved survival but with a concerning incidence of neurodevelopmental complications in survivors [44]. In CHD the lifetime impact of cardiovascular disease on neurovascular disease changes. In early childhood, neurodevelopmental disorders are the central issue but with age epilepsy, stroke and dementia become more prevalent [45]. Interestingly, the increased risk of epilepsy is also seen in nonsurgical CHD patients indicating the presence other neurodevelopmental factors unrelated with the perioperative period [46]. Patients with ACHD have an elevated risk of ischemic and hemorrhagic stroke [47]. Lanz et al. in a retrospective, population‐based cohort study showed that almost 8% of patients with ACHD will experience a stroke in adulthood [48]. The risk of stroke was to 9–12 times higher in younger patients (<55 years old) up and only double older patients (55–64 years old). Severe ACHD and left‐sided lesions carry the highest risk of stroke. The greatest predictors of ischemic stroke in patients with ACHD are heart failure, diabetes mellitus, and recent myocardial infarction. The thirty‐day mortality after stroke is comparable to the general population. Early‐onset dementia is more prevalent in patients with ACHD, and the burden of this disease is likely to increase with the growing population [49]. Patients with ACHD carry several risk factors for dementia including genetic disorders (e.g., Down syndrome), stroke, heart failure, atrial fibrillation, and diabetes mellitus. ACHD centers must acknowledge and accommodate some aspects of the pediatric anesthesia practice. Inhalational inductions are less practical, and premedication with hypnotics, and occasionally intramuscular ketamine, may be necessary. Patients’ impressions made on the first anesthetic encounter at the adult facility will influence future attitudes toward anesthetic care, and compliance with future visits. ACHD is associated with liver dysfunction. The mechanism of liver failure is multifactorial secondary to CHD, surgical repair or palliation, or blood transfusion [50]. Liver dysfunction is caused by passive venous congestion (e.g., central venous hypertension) or low‐output cardiac syndrome (LCOS). Tricuspid insufficiency and right ventricular failure cause liver failure by passive venous congestion. ACHD linked with passive venous congestion are repaired tetralogy of Fallot (TOF) with pulmonary insufficiency, Ebstein’s anomaly, single ventricle physiology (e.g., Fontan and Glenn), TGA with atrial switch repairs (e.g., Mustard‐Senning). Elevation of indirect bilirubin and prolonged international normalized ratio (INR) without affecting of the aminotransferases is typical in central venous hypertension. CHD conditions that cause LCOS, such as aortic coarctation or LV outflow tract obstruction, cause hepatic ischemia and eventually fibrosis. The ischemic burden is worse in the presence of an increased hepatic sinusoidal pressure. Acute ischemic hepatitis caused by LCOS is characterized by marked early rises of transaminases, followed by later increases in bilirubin and prothrombin time. Fontan patients are the highest ACHD risk group to develop liver failure with an incidence of over 40%, 30 years after the initial procedure [51]. Failing Fontan physiology patients are prone to develop liver fibrosis earlier due to the continuous back pressure on the sinusoids secondary to elevated non‐pulsatile central venous pressure (CVP). There is a strong correlation between hepatic fibrosis and high hepatic venous pressures, low cardiac index, and ventricular function. The gold standard for diagnosis of Fontan associated liver disease is the percutaneous liver biopsy under ultrasound guidance. Transvenous biopsies can be obtained at the time of the cardiac catheterization and transhepatic gradient can be measured. An elevated hepatic venous pressure gradient >5 mmHg is indicative of parenchymal liver disease. Gradients over 10 mmHg are associated with portal hypertension complications (e.g., ascites and variceal hemorrhage) [52, 53]. Model for End‐stage Liver Disease (MELD‐XI) scores, excluding INR scores, is used to classify the severity of liver disease in ACHD. The advantage of the MELD‐XI score is that the INR is excluded, and many patients with Fontan circulation are taking warfarin. In addition, there is a statistically significant correlation between MELD‐XI values and hepatic total fibrosis scores [52]. Once a Fontan patient is diagnosed with cirrhosis, close surveillance for hepatocellular carcinoma and esophageal varices assessment is recommended. There is no specific treatment for Fontan‐associated liver disease. Medical treatment should optimize Fontan physiology by increasing cardiac output (e.g., afterload reduction) and decreasing CVP (e.g., diuretics). Additional etiologies of liver injury should be avoided with immunization (e.g., hepatitis A and B) and limiting other toxins (e.g., alcohol or obesity). Combined heart and liver transplant is the ultimate treatment of failing Fontan physiology with associated liver disease. Hofer et al. recently reviewed the anesthetic management of combined heart–liver transplantation in patients with Fontan‐associated liver disease. The authors recommend keeping the transpulmonary pressure gradient close to 5 mmHg by optimizing preload, reducing PVR, and preserving single ventricular function [54]. Chapter 30 contains additional discussion of heart‐liver transplantation. Patients with ACHD undergo multiple interventions during infancy and childhood so by the time the reach adulthood the central and peripheral vascular access may be compromised. The use of ultrasound for vascular access is recommended in potential difficult intravenous access patients such as the ACHD population [55]. Chue et al. describe a 3.6% incidence of complications after femoral cannulation for percutaneous interventions in patients with ACHD [56]. The complications included hematoma and pseudoaneurysm. The preoperative visit should include examining the extremities for cut‐down scars and measuring blood pressure in all extremities. Planning for major cardiac surgery should consist of venous Doppler or magnetic resonance imaging (MRI) of the femoral vessels, central veins and arterial and venous studies. Computed tomography or MRI of the chest is essential to delineate the intrathoracic anatomy and the relationship between the heart and great vessels to the sternum [57]. The anesthesiologist should discuss plans for emergent cannulation of the femoral vessels in the event of a cardiac injury at sternotomy. Cardiac or vascular injury upon resternotomy in ACHD is more common in patients with extracardiac conduits and dilated structures (e.g., aorta, RV, or right atrium) [58]. One of the approaches to high‐risk sternotomy patients is establishing cardiopulmonary bypass through peripheral cannulation before sternotomy [59]. There are two types of unrestricted shunts. First, simple shunts, such as ASDs, VSDs, large PDAs, and aortopulmonary collaterals have a left‐to‐right shunt in the presence of normal or low PVR, and pulmonary blood flow may exceed systemic blood flow. Second, complex congenital lesions palliated with systemic‐to‐pulmonary artery shunt (e.g., BT, Waterston–Cooley, or Potts’ shunt) to augment pulmonary blood flow who may develop unilateral PAH. The Australian National registry for PAH in ACHD found that, of the first 50 registrants, 47 had systemic‐to‐pulmonary artery shunts [60]. At rest, breathing room air, these patients may have a 2:1 or as much as a 3:1 shunt ratio of pulmonary to systemic blood flow. The anesthesiologist should avoid 100% oxygen ventilation since it will lower the patient’s PVR, converting a 2:1 shunt to a 4–5:1 shunt, causing metabolic acidosis from diminished systemic oxygen delivery. Unrestricted left‐to‐right shunts can reverse with a profound decrease in systemic vascular resistance (SVR). The coronary circulation is dependent on the diastolic pressure and will compete with the run‐off into the pulmonary circulation when the PVR decreases by hyperventilation and 100% oxygen. After induction, baseline conditions should be targeted, maintaining the patient on a FiO2 that recreates their room air oxygen saturations at rest to avoid increasing the shunt fraction and coronary ischemia. Ventilation should be at lung volumes close to functional residual capacity (FRC) and maintain a normal PaCO2. The increase in adults living with CHD also reflected in the obstetrical population, with increasing numbers of women with ACHD needing pregnancy care. For example, in one analysis, the number of women with ACHD presenting for delivery increased from 6.4 to 9.0 per 10,000 delivery hospitalizations [61]. Effective communication among the care team is essential, and anesthesiologists play a crucial role in assuring appropriate care for women with ACHD during pregnancy, labor, and delivery. Anesthesiologists must understand the effect of pregnancy on the cardiovascular system, the unique physiology of each patient’s cardiac lesion or surgical repair, and the cardiovascular impact of various anesthetic techniques used on labor and delivery. A growing fetus requires increased oxygen delivery to the uteroplacental unit. Cardiac output increases by approximately 50% during pregnancy by increasing heart rate and stroke volume to keep up with the demand. Plasma volume significantly increases during pregnancy, reaching its peak around the early third trimester. Sympathetic tone increases in the first trimester (Figure 20.3) [62]. Transthoracic echocardiography (TTE) demonstrates increased cardiac performance and progressive LV remodeling. LV mass and LV end‐diastolic diameter increase, while LV strain decreases. These values return to normal in the postpartum period [63]. Systemic and PVRs both decrease. Mean arterial blood pressure decreases before returning to near‐normal values at the end of the third trimester [64]. Central filling pressures typically remain normal. Pregnancy is also associated with dilutional anemia. It is a hypercoagulable state with increases of most coagulation factors, a decrease in Protein S activity, and acquired Protein C resistance [65]. Labor and delivery bring additional cardiovascular demands that place stress on the heart. Autotransfusion from uterine contractions increases plasma volume and cardiac output up to 50% more than the pregnancy baseline throughout labor. In addition, painful contractions increase heart rate and SVR. During delivery, there is a large autotransfusion from uterine involution. This autotransfusion increases the circulating volume up to 100% over the baseline pregnant state, potentially overwhelming an already fragile circulation. As a result, it is a typical time for heart failure to occur [66, 67]. Cardiac and fetal risk during pregnancy and the postpartum period depends on the nature of the patient’s cardiac lesion, pre‐pregnancy functional status, and general health. Simple lesions, such as a repaired ASD or mild pulmonic stenosis, do not carry a significantly increased risk over the general population. However, complex ACHD, such as a Fontan circulation or unrepaired cyanotic heart disease, poses a greater risk to the patient and may become life threatening [68]. Ideally, patients with ACHD should plan their pregnancy after consultation at a tertiary care center with a cardio‐obstetric team. The team should consist of a high‐risk obstetrician, cardiologist, and obstetric anesthesiologist, all of whom have experience caring for patients with ACHD. Other experts should be consulted as necessary and include a cardiac anesthesiologist, cardiothoracic surgeon, cardiovascular intensivist, geneticist, neonatologist, hematologist, and others. In addition, preconception counseling should give the woman a clear understanding of the risks of pregnancy for her and her child. In some cases, additional testing and procedures may be warranted before it is deemed safe to embark upon pregnancy. Exercise testing is a valuable tool to aid in maternal and fetal risk stratification. Lack of a chronotropic response to exercise is predictive of both maternal and neonatal events [69]. Once pregnancy is confirmed, high‐risk patients should be referred to a tertiary care program with expertise in pregnancy in ACHD. Estimation of maternal and fetal risk during pregnancy and the postpartum period considers the mother’s underlying cardiac condition and the presence or absence of risk factors. The modified WHO (mWHO) pregnancy classification is regarded as the most accurate risk classification [64]. However, multiple risk scores are based on studies that have examined maternal and fetal risk in patients with cardiac disease. The mWHO classification is presented in Table 20.1 [68]. Its assessment of risk is based purely on underlying cardiac condition, assuming the mother is otherwise optimized. Figure 20.3 Hemodynamic changes in pregnancy. CO, cardiac output; SV, stroke volume; HR, heart rate; Hb, hemoglobin; TPVR, total peripheral vascular resistance. (Source: Ruys et al [62]. Reproduced with permission of Elsevier). In 2001, a large prospective observational trial examined 599 pregnancies in women with CHD (74%), acquired heart disease (22%) and arrhythmia (4%). A primary cardiac event occurred in 80 pregnancies (13%). Everyday cardiac events included heart failure and arrhythmia. They proposed four predictors of maternal cardiac complication (the CARPREG I score): prior cardiac event or arrhythmia, NYHA class >II or cyanosis, left heart obstruction, and systemic ventricular dysfunction [70]. An updated risk index, CARPREG II, was published in 2018. It included 1938 pregnancies in women with congenital (63.7%) and acquired (22.9%) heart disease. The new score incorporates different risk elements, including lesion‐specific variables, patient‐specific variables, and one process of care variable. It is outlined in Table 20.2 and Figure 20.4 [67]. The maternal complication rate was 16%, unchanged from their 2001 study. Arrhythmia (9.3%) and heart failure (6.2%) remained the most common cardiac events. Arrhythmias were most likely to occur in the antepartum period, where heart failure was most observed in the third trimester or the early postpartum period [67]. Table 20.1 Modified WHO classification of maternal cardiovascular risk Source: Adapted from Canobbioet al [68]. Abbreviations: PS, pulmonary stenosis; PDA, patent ductus arteriosus; MVP, mitral valve prolapse, ASD, atrial septal defect; VSD, ventricular septal defect, APVR, anomalous pulmonary venous return; HOCM, hyperthrophic obstructive cardiomyopathy; RV, right ventricle Table 20.2 CARPREG II risk stratification index Source: Adapted from Silversides et al [67]. Figure 20.4 Maternal complication risk based on CARPREGII score. (Source: Silversides et al [67]. Reproduced with permission of Elsevier). In a more extensive retrospective review from the ZAHARA group, 1802 pregnancies were observed in women with ACHD. The maternal cardiac complication rate was 7.6%. The most common maternal cardiac complications were arrhythmias (4.7%) and heart failure (1.6%). They confirmed several risk factors from the CARPREG studies. In addition, they identified further risk factors: the use of cardiac medication before pregnancy and systemic and pulmonic AV valve regurgitation in the context of complex ACHD [71]. A smaller study, examining 90 pregnancies in women with ACHD, identified two additional risk factors: impaired sub pulmonary ventricular systolic dysfunction and severe pulmonary regurgitation [72]. Single ventricle physiology was a risk factor for maternal cardiac events in a ACHD population [73]. External validation and comparison of the CARPREG I, ZAHARA, and mWHO risk classification systems in a ACHD population was undertaken in 2014. The mWHO score was best able to discriminate risk for cardiovascular events. In addition, a combination of the mWHO score, the total number of cardiovascular predictors, and disease complexity further improved prediction [74]. The most common fetal complications in women with ACHD include premature birth (12–19%) and small for gestational age (SGA) birth weight. In addition, neonatal death occurred at a higher rate than the general population, ranging from 2.8–5%. There was also a higher observed incidence of intrauterine growth restriction (IUGR), neonatal intensive care (NICU) admission, and neonatal CHD [70–74]. The increase in premature births has two causes: preterm labor and iatrogenic premature delivery due to maternal health status or decompensation. Risk factors for fetal complications include maternal NYHA class >II, cyanosis, maternal anticoagulation, left heart obstruction, mechanical valve, cardiac medications during pregnancy, single ventricle physiology, severe pulmonary hypertension, smoking, and multiple gestations. Patients with ACHD are at elevated risk for preeclampsia and postpartum hemorrhage (PPH). Risks for preeclampsia included nulliparity, systemic lupus erythematosus (SLE), and aortic coarctation [61]. In addition, several studies have identified CHD as an independent risk factor for PPH, and patients with Fontan circulation had a particularly elevated risk [75–77]. Peripartum anticoagulation and cyanosis are other identified risk factors for PPH [70]. While anticoagulation during pregnancy is a risk factor for PPH and neonatal complications, for some conditions, the additional thrombotic risk conferred by pregnancy and the catastrophic effect of a thrombus in these conditions is significant. These conditions include a Fontan circulation or the presence of a mechanical valve [78–81]. The balance of risk and benefit for anticoagulation in pregnant patients with ACHD is an individualized decision. Low‐molecular‐weight heparin (LMWH) is the preferred anticoagulant during pregnancy. Neither unfractionated heparin (UFH) nor LMWH crosses the placenta. LMWH is the anticoagulant of choice over UFH due to its reliability and relative ease of dosing. It is also associated with fewer injection site reactions and carries a lower risk of heparin‐induced thrombocytopenia (HIT). However, the longer half‐life LMWH and the inability to completely reverse its anticoagulant effect may limit the ability to provide neuraxial anesthesia. Warfarin is typically avoided during pregnancy as it is associated with well‐known fetal malformations, particularly with exposure in the first trimester. Alternatives to warfarin should be used if clinically feasible. Direct oral anticoagulants have gained popularity for anticoagulation for several indications. However, there is scant data for use during pregnancy, and they are not recommended [82]. The presence of a mechanical valve is a known risk factor for cardiac complications [64, 67, 71]. The main risks are valve thrombosis and bleeding complications related to anticoagulation. In a 2015 study, women with a mechanical valve only had a 58% chance of having an event‐free pregnancy, 4.7% experienced valve thrombosis, which carried a 20% mortality rate. Hemorrhage occurred in 23% of patients, usually around the time of delivery [83]. Management of anticoagulation in this population is a challenge. Multiple studies have shown an increased risk of thrombosis in mechanical valve patients treated with LMWH vs. warfarin [83–86]. Warfarin is optimal for thrombosis prevention and is recommended for use during pregnancy in patients with a mechanical valve despite the risk of embryopathy. Patients may remain on warfarin during their entire pregnancy, or warfarin can be changed to LMWH or UFH for the first trimester and again after 36 weeks [64]. Patients may present in pregnancy for cardiac interventional procedures or cardiac surgery. Ideally, cardiac procedures will occur in the second trimester when organogenesis is complete, and the risk of premature labor is still low. The uterus must be double shielded from radiation exposure during catheterization. Appropriate fetal monitoring is indicated both for intervention if the fetus is viable and to optimize hemodynamics in response to fetal distress. Discussion with the obstetric team should take place on the response to the diagnosis of fetal distress. The anesthesiologist should be cardiac trained with ACHD expertise. Cardiac surgery and perfusion should be on standby, and appropriate ICU resources available for the mother and fetus. Maternal cardiac surgery should only be performed during pregnancy if it is urgent, as in LV obstruction with symptoms at rest, or emergent, as in aortic dissection or a thrombosed mechanical systemic AV valve. Cardiac surgery during pregnancy and the use of cardiopulmonary bypass carries a high risk of fetal mortality. Normothermic perfusion, mean arterial pressure (MAP) >70 mmHg, minimal CPB times, and bypass pump flows >2.5 L/min/m2 are optimal to minimize fetal risk. Hypocapnia, which ordinarily may be used for patient’s dependent on a low PVR (TOF, Ebstein’s anomaly, and Fontan circulation), has adverse effects causing uteroplacental vasoconstriction and fetal hypoxia and should therefore be avoided. If the fetus is viable, a caesarean section (C‐section) may precede the urgent cardiac surgery [69, 87, 88]. Multidisciplinary collaboration is essential to planning a safe delivery for mother and baby. Thoroughly assessing each patient’s risk and anticipated complications allow mobilization of appropriate resources and determining who needs to be involved in the patient’s delivery. Additional teams consulted may include ICU, cardiac surgery, cardiac anesthesia, and others. The team should also determine the optimal delivery mode, location, timing, and anesthetic plan. The delivery hospital must have the necessary resources to care for the patient and handle any likely complications. This level of care depends on the estimate of the patient’s risk and severity of her condition. For example, low‐risk patients, mWHO class I and II, estimated cardiac event risk <10%, could safely be delivered at a local hospital. Patients with an intermediate risk (mWHO class II‐III, calculated risk 10–19%) should deliver at a higher‐level referral enter, while extraordinary risk patients (mWHO class II or IV) should refer to a tertiary care center experienced in the care of patients with ACHD [64, 89]. The mode of delivery determines birth location within the hospital, need for invasive monitoring, access to the escalation of care, and ability to perform emergency surgery quickly. The team should determine what resources and monitoring are necessary for delivery and then decide which location in their hospital best fulfill those needs. For example, if a C‐section is planned, the operating room should be equipped to accommodate standard C‐section needs in addition to possible contingencies, including demand for emergent ECMO cannulation or transesophageal echocardiography (TEE) and neonatal resuscitation. Vaginal delivery is preferred in patients with ACHD unless an obstetric indication for C‐section is present. Elective C‐section based on maternal cardiac disease is not recommended, as it does not confer maternal benefit but may carry increased neonatal risk [90]. Vaginal delivery is associated with decreased blood loss and a lower risk of venous thromboembolism. For certain patients, an assisted vaginal delivery minimizes Valsalva and has beneficial hemodynamic effects. C‐section recommendations include acute intractable heart failure, patients presenting in labor on oral anticoagulants, aggressive aortopathy, and severe pulmonary hypertension [64]. During labor, painful contractions can lead to tachycardia, hypertension, and hyperventilation. Early labor epidural placement can mitigate these unwanted effects. Sympathectomy associated with epidural local anesthetic administration causes vasodilation and can lead to hypotension. Therefore, cautious administration of a pre‐load or co‐load of IV fluid before or during epidural placement is beneficial. If the plan is to assist the second stage, good sacral nerve root coverage is required. The use of a combined spinal‐epidural (CSE) or dural puncture epidural (DPE) technique achieves good sacral coverage. Opioid‐only intrathecal injection (10 mcg Fentanyl) should be used in CSE to minimize the extent of sympathectomy and prevent hypotension. For patients with intracardiac shunts, consider using saline rather than air for the loss of resistance technique to minimize inadvertent air injection into a blood vessel during epidural placement. We do not advise the use of epinephrine in the epidural test dose. The intravascular injection of epinephrine could be detrimental to patients at high risk of arrhythmia. An alternative test dose is epidural fentanyl 50–100 mcg [91]. For patients undergoing C‐section, neuraxial anesthesia is preferred over general anesthesia. In Figure 20.5, the advantages, and disadvantages of each are outlined. The selection of neuraxial technique depends on the physiology and severity of the patient’s cardiac lesion. Spinal anesthesia produces a quick onset, reliable, dense surgical block. However, it is also accompanied by a rapid sympathectomy which causes an acute decrease in preload and afterload. Preload‐dependent patients or severe (mWHO III‐IV) lesions will not tolerate the sympathectomy. Epidural anesthesia can be titrated to ensure a slower onset and slow start sympathectomy but may produce a less dense or unilateral block that is inadequate for surgical anesthesia. A CSE with intrathecal opioids or a sequential CSE, in which a small dose of local intrathecal anesthetic (2.5–5 mg 0.75% hyperbaric bupivacaine) is given and then supplemented with a local epidural anesthetic, can provide the balance between a reliable, dense block and the need for slow titration to avoid rapid sympathectomy, decreased SVR, and hypotension. Neuraxial anesthetic dosing recommendations for cesarean delivery are summarized in Table 20.3. Figure 20.5 Advantages, and disadvantages of the administration of general vs. neuraxial anesthesia for cesarean section. Anticoagulated patients require general anesthesia, and those unable to lay flat, critically ill requiring mechanical ventilation, or emergent delivery. Induction and maintenance of anesthesia should balance obstetric and cardiac considerations. In obstetric patients, increased aspiration risk leads to the use of rapid sequence induction. One should avoid opioids before delivery due to concern for neonatal sedation and respiratory depression. However, patients with high‐risk cardiac lesions may require a slower induction to prioritize hemodynamic stability. Therefore, the administration of opioids to blunt the hypertensive response to laryngoscopy may be reasonable. Adequate monitoring for the patient during delivery, whether vaginal or C‐section, is vital for early recognition of maternal warning signs. For vaginal delivery, intermittent noninvasive blood pressure and pulse oximetry are essential for cardiac patients. Telemetry during and after delivery is mandatory for patients at risk for arrhythmia. For patients at higher risk of decompensation, invasive arterial blood pressure or CVP monitoring can be considered. Point of care TTE can be helpful to serially monitor cardiac function in patients with depressed systemic ventricular ejection fraction. For C‐sections, standard monitors are sufficient for low and intermediate‐risk patients. High‐risk patients will likely require invasive arterial blood pressure or CVP monitoring and possibly pulmonary artery (PA) catheter placement. In patients who are under general anesthesia, TEE can be used. Patients with ACHD need an individualized transfusion plan before delivery since they have an elevated risk of PPH. Most uterotonic medications have cardiovascular effects and need to be administered with caution. The hemodynamic results of commonly used uterotonic drugs are summarized in Table 20.4 [92]. Tranexamic acid (TXA) reduced PPH mortality in the WOMAN trial, which included over 20,000 women worldwide. There was no difference in cardiac failure, thromboembolism, or seizure between the TXA and placebo groups [93].
CHAPTER 20
Anesthesia for Adults with Congenital Heart Disease
Introduction
Noncardiac sequelae of CHD
Pulmonary sequelae
Pulmonary arterial hypertension
Hematological sequelae
Renal sequelae
Neurological sequelae
Hepatic sequelae
Vascular access considerations
Unrestricted shunts
Pregnancy
Relevant physiologic changes during pregnancy
Cardiac risk assessment before and during pregnancy
mWHO I
mWHO II
mWHO
II‐III
mWHO III
mWHO IV
Diagnosis (if otherwise well and uncomplicated
Uncomplicated, small or mild
Successfully repaired simple lesions (ASD, VSD, PDA, APVR).
Atrial or ventricular ectopic beats, isolated
Unoperated ASD or VSD
Repaired TOF
Most arrhythmias
Mild LV impairment
HOCM
Native or tissue valvular heart disease not considered WHO I or IV
Marfan syndrome without aortic dilatation
Aorta <45 mm in aortic disease associated with bicuspid aortic valve
Repaired coarctation
Mechanical valve
Systemic RV
Fontan circulation
Cyanotic heart disease (unrepaired)
Other complex congenital heart disease
Aortic dilatation 40–45 mm in Marfan syndrome
Aortic dilatation 45–50 mm in aortic disease associated with bicuspid aortic valve
PHTN of any cause
Severe systemic ventricular dysfunction (LVEF <30%, NYHA III–IV)
Previous peripartum cardiomyopathy with any residual impairment of left ventricular function
Severe mitral stenosis, severe symptomatic aortic stenosis
Marfan syndrome with aorta dilated >45 mm
Aortic dilatation >50 mm in aortic disease associated with bicuspid aortic valve
Native severe coarctation
Risk
No detectable increased risk of maternal mortality and no/mild increase in morbidity.
Small increased risk of maternal mortality or moderate
increase in morbidity
Intermediate increased risk of maternal mortality or moderate‐to‐severe increase in morbidity
Significantly increased risk of maternal mortality or severe morbidity. Expert counselling required.
If pregnancy is decided upon, intensive specialist
cardiac and obstetric monitoring needed throughout
pregnancy, childbirth, and the puerperium
Extremely high risk of maternal mortality or severe morbidity; pregnancy contraindicated. If pregnancy occurs termination should be discussed. If pregnancy continues, care as for class III.
Maternal cardiac event rate
2.5–5%
5.7–10.5%
10–19%
19–27%
40–100%
PREDICTOR
SCORE
Prior cardiac events or arrhythmias
3
Baseline NYHA III‐IV or cyanosis
3
Mechanical valve
3
Ventricular dysfunction
2
High‐risk left‐sided valve disease/left ventricular outflow tract obstruction
2
Pulmonary hypertension
2
Coronary artery disease
2
High‐risk aortopathy
2
No prior cardiac intervention
1
Late pregnancy assessment
1
Maternal obstetric and fetal risks
Care during pregnancy
Anticoagulation
Cardiac procedures during pregnancy
Delivery planning
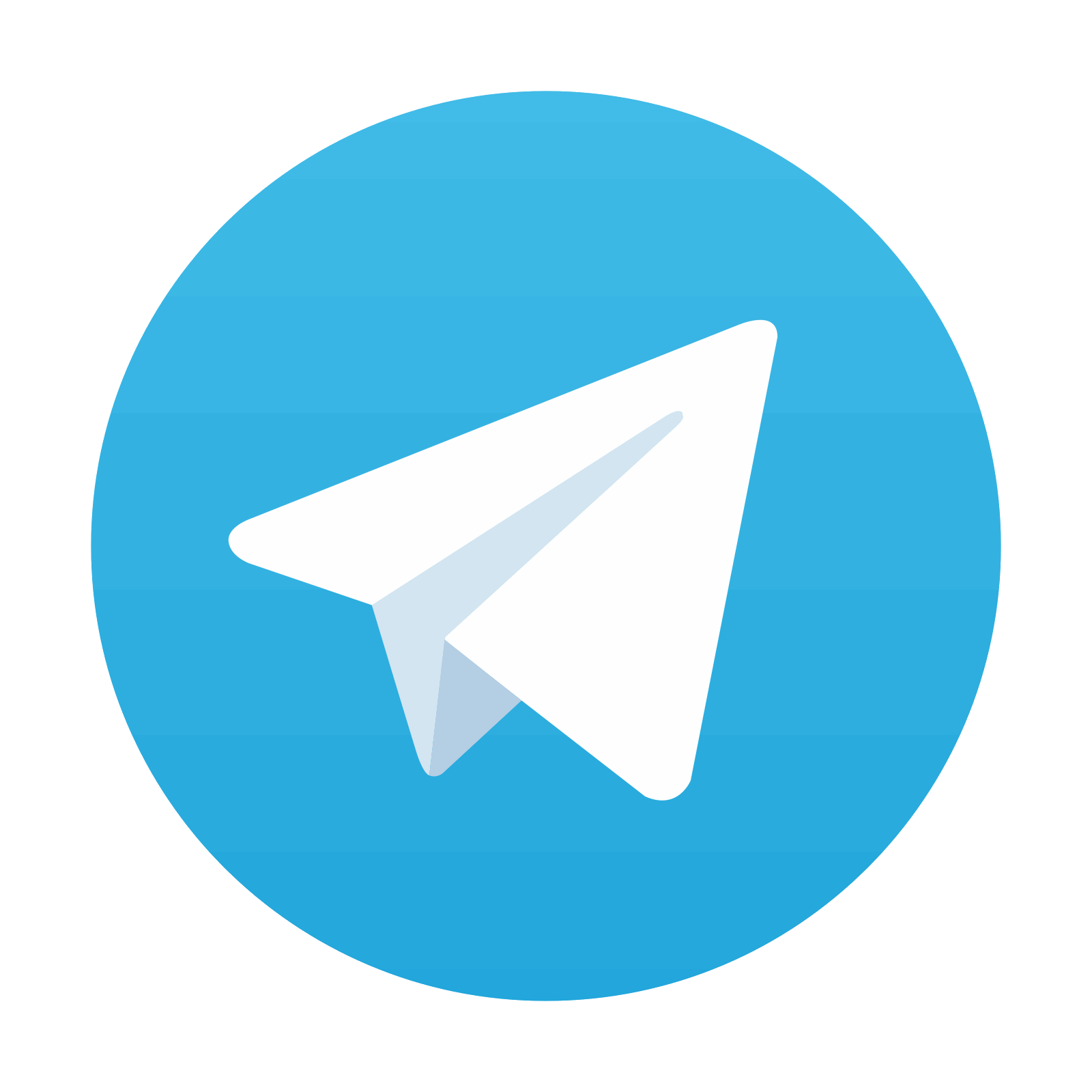
Stay updated, free articles. Join our Telegram channel

Full access? Get Clinical Tree
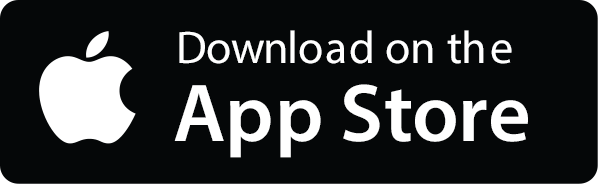
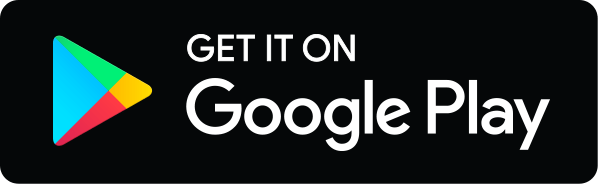