Abstract
Liver surgery can be remarkably safe: a zero mortality rate has been achieved with liver resections when patients are properly selected and with meticulous perioperative care [1]. In order to maintain liver function in individual patients undergoing anaesthesia and surgery, the single most important factor is maintaining its perfusion. In order to avoid hypoxic liver injury, preserving sinusoidal blood flow is best done by maintaining an adequate perfusion pressure and avoiding a high central venous pressure. Reducing intraoperative blood loss and maintaining systemic haemodynamics likely play major roles in avoiding hypoxic liver injury. It is still unknown which vasoactive drugs are preferred when haemodynamic instability occurs; Noradrenaline seems to be well tolerated as long as hypovolaemia is avoided. Ischaemic preconditioning and pharmacological preconditioning and postconditioning are promising, but their clinical relevance remains to be determined. Finally there are no good markers of hepatocyte damage that could be used intraoperatively to optimize anaesthetic management.
Summary
Liver surgery can be remarkably safe: a zero mortality rate has been achieved with liver resections when patients are properly selected and with meticulous perioperative care [1]. In order to maintain liver function in individual patients undergoing anaesthesia and surgery, the single most important factor is maintaining its perfusion. In order to avoid hypoxic liver injury, preserving sinusoidal blood flow is best done by maintaining an adequate perfusion pressure and avoiding a high central venous pressure. Reducing intraoperative blood loss and maintaining systemic haemodynamics likely play major roles in avoiding hypoxic liver injury. It is still unknown which vasoactive drugs are preferred when haemodynamic instability occurs; Noradrenaline seems to be well tolerated as long as hypovolaemia is avoided. Ischaemic preconditioning and pharmacological preconditioning and postconditioning are promising, but their clinical relevance remains to be determined. Finally there are no good markers of hepatocyte damage that could be used intraoperatively to optimize anaesthetic management.
Introduction
The goal of every anaesthesiologist is not just to anaesthetize the patient but also to protect the patient from potential harm, including that as a result of tissue injury caused by the surgical procedure. One of the key organs that has to be protected is the liver, because a properly functioning liver is required for survival. With minor surgery, there is virtually no chance that the liver can be harmed to any significant degree, and this is true whether the liver is healthy or diseased. In this situation the anaesthetic technique that is chosen plays virtually no role in the overall outcome of the liver, as long as global haemodynamics and oxygenation are maintained. Nevertheless, signs of minor liver injury postoperatively are not uncommon even in healthy patients [2]. The situation becomes more complex when the procedure is more extensive, with more tissue injury due to the surgery, with major abdominal procedures influencing splanchnic blood flow, when the liver is the subject of the surgical procedure, and when dealing with a diseased liver. Thus factors that play a role are changes in global and regional circulation, liver versus non-liver surgery, and presence or absence of preexisting liver dysfunction as the diseased liver is more susceptible to further injury. Liver trauma or vascular injury during any liver surgery can result in major bleeding requiring temporary interruption of liver blood flow through the Pringle manoeuvre, achieved by placing a vascular clamp on the hepatic pedicle, thereby interrupting arterial and portal venous inflow into the liver. However, it should be of limited duration because the degree of tissue injury is related to the duration of ischaemia [3], and preferably it should be avoided completely unless the risk of major bleeding justifies its use.
There are two main mechanisms to injure the liver perioperatively: reduce its blood flow, or submit it to toxins. Since in general modern anaesthetic agents are not hepatotoxic, we will focus on reduced liver blood flow as the cause of perioperative liver dysfunction. Liver blood flow can be reduced as a result of global haemodynamic instability, or as a result of hepatic pedicle clamping (Pringle manoeuvre). The Pringle manoeuvre, in combination with low CVP, is used to reduce blood loss during parenchymal transection.
It is imperative to first discuss normal liver blood flow at a macro- AND a micro-level. It is also important to recognize why the diseased liver is more vulnerable to a further reduction in blood flow. How exactly the hepatic sinusoidal blood flow in liver disease is abnormal (liver fibrosis, liver cirrhosis) will be presented in order to appreciate that perioperative hepatic injury is more likely to occur in the already diseased liver.
Physiology
Liver Anatomy and Histology
Liver anatomy by itself is not really relevant to this topic. What is important is the histology: rows of hepatocytes are neatly lined up with the capillaries called sinusoids, separated by highly fenestrated endothelial cells; this results in an optimal environment for extensive bidirectional metabolic exchange between sinusoidal blood and hepatocytes. The space between the sinusoids and the hepatocytes is called the space of Disse and it contains loose connective tissue, phagocytic Kupffer cells and hepatic stellate cells (Ito cells). The excessive fluid in the space of Disse is drained into the lymphatic system (Fig. 17.1 and Fig. 17.2) [4, 5].
Fig. 17.1 Vascular and architectural alterations in cirrhosis.
Hepatic sinusoids receive blood from the portal vein and hepatic artery. (A) Healthy liver: terminal portal tract blood runs through hepatic sinusoids where fenestrated sinusoidal endothelia that rest on loose connective tissue (space of Disse) allow for extensive metabolic exchange with the lobular hepatocytes; sinusoidal blood is collected by terminal hepatic venules that drain into one of the three hepatic veins and finally the inferior vena cava. (B) Cirrhotic liver: activated myofibroblasts that derive from perisinusoidal hepatic stellate cells (Ito cells) and portal or central-vein fibroblasts proliferate and produce excess extracellular matrix. This event leads to fibrous portal-tract expansion, central-vein fibrosis and capillarization of the sinusoids, characterized by loss of endothelial fenestrations, congestion of the space of Disse with extracellular matrix, and separation or encasement of perisinusoidal hepatocyte islands from sinusoidal blood flow by collagenous septa. Blood is directly shunted from terminal portal veins and arteries to central veins, with consequent (intrahepatic) portal hypertension and compromised liver synthetic function [4].
Fig. 17.2 Pathological sinusoidal remodelling in cirrhosis and portal hypertension.
Hepatic stellate cells (HSC) align themselves around the sinusoidal lumen in order to induce contraction of the sinusoids. While in normal physiological conditions HSC contractility and coverage of sinusoids is sparse, in cirrhosis, increased numbers of HSC with increased cellular projections wrap more effectively around sinusoids, thereby contributing to a high-resistance, constricted sinusoidal vessel. At the cellular level, a number of growth factor molecules contribute to this process through autocrine and paracrine signalling between HSC and sinusoidal endothelial cells (SEC). A number of these molecules are depicted along with their proposed role in paracrine function. PDGF: platelet-derived growth factor; VEGF: vascular endothelial growth factor; Ang-1: angiopoietin 1; NO: nitric oxide; TGF-β: transforming growth factor beta [5].
Liver Function
The function of the liver is complex; the liver is involved in production of most proteins in the blood (except immunoglobulins), including albumin, most coagulation factors and several inhibitors of the coagulation system. The liver plays a key role in carbohydrate metabolism, including storage (glycogen), glucose breakdown and gluconeogenesis. Lipid metabolism as well as cholesterol synthesis is complex. Bilirubin is cleared from the blood and after conjugation secreted into the intestinal tract with the bile. Bile production and secretion into the gut facilitates the digestion of ingested fats. Finally, biotransformation (deactivation but sometimes activation) of drugs, chemicals such as alcohol and endogenous substances occurs in the liver.
Global Liver Blood Flow
The liver receives a dual blood supply: the hepatic artery delivers oxygenated blood to the hepatic sinusoids, while the portal vein delivers partially deoxygenated but nutrient- and hormone-rich blood from the splanchnic organs. Hepatic arterial blood flow represents about 25–30% of total liver blood flow, and portal venous blood flow about 65–70%; each contributes about 50% of the oxygen supply to the liver. A decrease in hepatic oxygenation results in increased oxygen extraction rather than an increase in hepatic arterial blood flow [6]. A decrease in portal vein blood flow leads to dilatation of the hepatic artery, increasing hepatic arterial blood flow, and vice versa: this is the so-called hepatic arterial buffer response that is based on changes in adenosine concentrations [7, 8, 9].
Effects of Anaesthesia and Surgery on Liver Blood Flow and Liver Function
Every major anaesthetic technique (general anaesthesia, spinal and epidural anaesthesia) results in a reduction in total liver blood flow. The modern inhaled agents (sevoflurane, desflurane, isoflurane) reduce liver blood flow less than halothane. The reduction in liver blood flow is mainly the result of a reduction in cardiac output and portal venous blood flow; hepatic arterial blood flow usually increases as a result of the hepatic arterial buffer response, but this does not restore liver blood flow to normal levels [10]. In general, hypotension is associated with a reduction in liver blood flow. Halothane reduces total hepatic blood flow more than other agents because it also increases hepatic arterial vascular resistance [11]. Major surgery, but especially upper abdominal surgery, results in a further reduction in liver blood flow [10].
Intravenous anaesthetics and opioids probably have less effect on liver blood flow than inhaled anaesthetics, although they have not been studied as well. While thiopental and etomidate seem to decrease total liver blood flow, it is unchanged with ketamine and increased with propofol, likely the result of splanchnic vasodilatation which results in increased portal inflow [12]. Intravenous anaesthetics have little or no adverse effect on liver function when haemodynamics are maintained.
Liver function tests are usually mildly increased after surgery and anaesthesia [13, 14], but anaesthesia with modern potent inhaled anaesthetics without surgery does not result in abnormalities in liver function tests [15, 16, 17].
There are very few studies determining the effect of general anaesthesia on liver blood flow and hepatic function in patients with preexisting liver disease. Overall, current inhaled anaesthetics do not seem to affect liver function in adult surgical patients with chronic liver disease [18].
Xenon has no direct haemodynamic effects and does not seem to influence total liver blood flow. In addition, liver function tests remain unchanged after xenon anaesthesia. It may be the ideal anaesthetic with regard to hepatic perfusion and function although its potential hepatic protective effects remain unstudied [19].
Pathophysiology: Liver Fibrosis and Cirrhosis
Changes in Histology and Sinusoidal Blood Flow
The most typical forms of liver disease are fibrosis and cirrhosis. Fibrosis is an abnormal healing response to liver injury, with activation of Ito cells resulting in their proliferation. The Ito cells transform into active fibroblasts that deposit an excessive amount of fibrin strands in the peri-sinusoidal areas, and into myofibroblasts that can contract and increase the resistance to flow in the sinusoids. There is the formation of fibrous septa, interfering with the metabolic exchange between hepatocytes and sinusoidal blood (Fig. 17.1 and Fig. 17.2).
Cirrhosis is the progression of fibrosis with the creation of regenerative nodules whereby there is distortion of the hepatic vasculature resulting in a further increase in intrahepatic vascular resistance, causing portal hypertension. An abnormal balance between intrahepatic vasoconstrictors and vasodilators contributes to the increased intrahepatic vascular resistance. The space of Disse contains fibrous tissue, and in addition the endothelial fenestrations are lost; as a result the sinusoidal blood drains into the terminal hepatic veins without undergoing adequate metabolic exchange (Fig. 17.1 and Fig. 17.2).
Metabolic Changes
Liver fibrosis but especially liver cirrhosis has significant metabolic consequences; these are the direct result of the inadequate metabolic exchange between sinusoidal blood and hepatocytes. Cirrhosis results in hepatocellular dysfunction, leading to hyperbilirubinaemia, reduced synthesis of albumin and coagulation factors, and reduced clearance of toxins and intestinal vasoactive substances. Pharmacokinetics of drugs that undergo hepatic metabolism is altered. Portosystemic collateral circulation worsens the effects of hepatocellular dysfunction.
Changes in Sinusoid Blood Flow and Liver Blood Flow
Cirrhosis results in reduced sinusoidal perfusion due to the mechanical obstruction of the sinusoids by regenerative nodules and constriction of sinusoids by contraction of surrounding myofibroblasts, resulting in hepatocellular dysfunction and portal hypertension. The portal hypertension is aggravated by the reduced local production of nitric oxide and excessive production of vasoconstrictors, both the result of endothelial dysfunction. An increase in nitric oxide production in the splanchnic circulation, however, leads to an increased intestinal inflow, again worsening portal hypertension. Release of vascular endothelial growth factor in the splanchnic system results in the development of portosystemic shunts (collateral vessels between the portal vein and the azygos system), further contributing to overall hepatic dysfunction (portal blood bypassing the liver). Although poorly studied, it seems that the arterial inflow into the hepatic sinusoids in the cirrhotic liver is reduced.
Changes in Macrohaemodynamics
There is a change in global haemodynamics: hyperdynamic circulation with high cardiac output, low systemic vascular resistance and relatively low systemic blood pressure. The hepatocellular dysfunction and the blood bypassing the liver result in globally increased concentrations of nitric oxide, prostaglandins, oestrogen, bradykinin and vasoactive intestinal peptide. In addition, there is a reduced sensitivity to vasoconstictors such as noradrenaline, vasopressin and endothelin-1 due to a decreased number of receptors and post-receptor defects. The low splanchnic vascular resistance is a major contributor to the low systemic vascular resistance. Although there is an increase in total blood volume, central blood volume is reduced, resulting in activation of baroreceptors with subsequent activation of compensatory mechanisms that are proportional to the degree of liver disease [20]. These profoundly affect the macrohaemodynamics: there is activation of the sympathetic nervous system, activation of the ADH–arginine–vasopressin pathway, activation of the renin–angiotensin–aldosterone system and increased concentrations of circulating endothelin. These compensatory systems result in further increases in heart rate and cardiac output, reduced renal perfusion (sodium and water retention) and overall a gradual decrease in effective organ perfusion. With progressive liver failure, these compensatory systems become insufficient to maintain effective tissue perfusion, ultimately resulting in multiorgan failure.
Although cirrhosis is typically associated with an increased cardiac output, there is impairment of cardiac function as a result of cirrhotic cardiomyopathy. Cirrhotic cardiomyopathy is characterized by the following triad: systolic and diastolic dysfunction in combination with electrophysiological abnormalities. Pathophysiological mechanisms of cirrhotic cardiomyopathy include downregulation of beta-receptors, abnormal excitation–contraction coupling, circulating myocardial depressant substances and areas of myocardial fibrosis and subendothelial oedema. Cardiac systolic dysfunction may initially not be apparent at rest because it is masked by the low systemic vascular resistance, but it can be provoked by cardiac stress such as placement of TIPS or liver transplantation, or increase in afterload as a result of vasopressin, terlipressin or noradrenaline administration. Left atrial enlargement is frequently seen on echocardiography, with a reduction in E/A ratio across the mitral valve (E wave reflects passive flow through the mitral valve, and A wave is the flow that results from atrial contraction). The change in E/A ratio is a reflection of LV diastolic dysfunction.
Anaesthetic Management in Patients with Liver Cirrhosis
The basic management of general anaesthesia changes little in the presence of cirrhosis. The main concern is the altered pharmacokinetics of anaesthetic agents, and the maintenance of liver blood flow. The use of drugs that are metabolized by the liver needs to be adjusted. For example, rocuronium has an increased duration of action in patients with liver cirrhosis, but this likely has become clinically irrelevant with the availability of sugammadex. Other aspects of anaesthetic management (global haemodynamic goals and management, choice of anaesthetic agents for their ischaemic preconditioning effect) will be discussed later in this chapter.
Perioperative Liver Injury
Hypoxic Liver Injury: Overview
Hypoxic liver injury is also called ischaemic hepatitis, shock liver or hypoxic hepatitis. Hypoxic liver injury is the result of an imbalance between hepatic oxygen supply and demand in the absence of other acute causes of liver damage, resulting in a diffuse hepatic injury. The direct cause is inadequate oxygen delivery to the liver. Most frequently it is caused by severe and prolonged hypotension and low cardiac output, but sometimes severe hypoxaemia or low haemoglobin concentration is the culprit. The Pringle manoeuvre interrupts liver blood flow completely and is obviously a risk factor. Rarely, increased oxygen consumption by the liver, seen with hyperthermia, could contribute to the imbalance. The severity of hypoxic liver injury depends on the duration of the insult. It is manifested by a large (usually > 10-fold) increase in serum transaminase levels which normalizes quickly over several days. During liver injury, intracellular enzymes such as AST and ALT are released into the plasma because of increased hepatocellular permeability. If the hypoxic injury is relatively mild, there will be acute hepatic impairment consisting of synthetic dysfunction (increased bilirubin, INR) and elimination dysfunction (e.g. reduced ICG clearance). Its more severe form is acute hepatic failure, characterized by encephalopathy, coagulation abnormalities, jaundice and intracranial hypertension; this has a high risk of mortality without liver transplantation. On liver biopsy centrilobular necrosis is seen because the area around the central vein is most prone to ischaemic damage. Differential diagnosis includes toxin-or drug-induced hepatitis (e.g. paracetamol) and acute viral hepatitis. It should be distinguished from hepatic infarction, which represents a focal injury to the liver. Treatment and prognosis are determined by the underlying disease. In the intensive care unit, hypoxic liver injury has a 50% mortality rate [21].
Risk Factors for Hypoxic Liver Injury
The diseased liver (liver fibrosis but especially cirrhosis) already has a reduced sinusoidal blood flow with inadequate metabolic exchange between sinusoidal blood and hepatocytes due to fibrosis; this makes the hepatocytes more susceptible to ischaemic injury, including in the perioperative setting. Portosystemic collaterals further reduce sinusoidal blood flow. Variceal bleeding, a common complication of cirrhosis, may result in hypotension, ultimately leading to hypoxic liver injury. Clinical experience has shown that anaesthesia and surgery in patients with cirrhosis carries increased risks, with an increase in perioperative mortality rate proportional to severity of liver cirrhosis, with abdominal and emergency procedures further increasing mortality [22].
Also, chronic hepatic congestion and sepsis increase the liver’s susceptibility to hypoxic liver injury [23]. The increased hepatic vein pressure not just reduces hepatic perfusion but also promotes translocation of intestinal bacteria into the portal vein as a result of congested ischaemic bowel, leading to the exposure of the hepatocytes to endotoxins, as is the case with sepsis. Endotoxins cause Kupffer cells to release pro-inflammatory cytokines that result in translocation of neutrophils. Substances released by both Kupffer cells and activated neutrophils activate platelets, causing the formation of microthrombi in the hepatic vessels. The inflammatory process also results in a worsening of the hepatocyte function.
Mechanisms of Ischaemia–Reperfusion Injury
Injury to the liver after ischaemia becomes apparent only after restoration of blood flow. A complete discussion of the mechanisms of ischaemia–reperfusion injury is beyond the scope of this chapter. Briefly, the initial injury is the deprivation of the organ of tissue oxygen. The severity of organ injury depends on the extent of activation of the Kupffer cells, the degree of platelet and leucocyte activation, and the extent of production of inflammatory mediators [24]. Reperfusion of the tissues following a period of ischaemia results in the generation of oxygen-derived radicals or reactive oxygen species and other mediators; this leads to production of pro-inflammatory mediators and activation of neutrophils, eventually resulting in tissue damage and potentially cell death. Sinusoidal endothelial cells are susceptible to cold ischaemia, leading to increased expression of vWF on their surface, resulting in platelet adhesion to the endothelial cell surface. Activation of Kupffer cells results in the release of reactive oxygen species and cytokines. Thus, hepatic injury occurs as the result of increased hepatic reactive oxygen species production, cellular pH changes, increased inflammatory responses and reduced hepatic microcirculation by sinusoidal vasoconstriction. Hepatic steatosis worsens the outcome of ischaemia–reperfusion because steatotic livers are less tolerant to the ischaemia–reperfusion insult [25]. Techniques that may temper this ischaemia–reperfusion injury will be discussed later.
Other Causes of Perioperative Liver Injury
Drug-related hepatotoxicity is fairly uncommon (except for paracetamol). Other potential causes of acute liver injury include toxins, acute hepatitis and autoimmune hepatitis. Early administration of intravenous N-acetylcysteine in non-paracetamol acute liver failure patients with encephalopathy is associated with an improved outcome [26].
Management of Perioperative Liver Injury
Management is based on the correct diagnosis and identification of the cause of the perioperative liver injury. Obviously if drug toxicity is suspected the causative agent should be discontinued. Supportive therapy should be initiated, but there is little direct intervention. It seems logical to maintain adequate organ perfusion, fluid balance and electrolyte concentrations.
Intraoperative Monitoring of Liver Well-being
Macrohaemodynamic Monitoring
Although there is an unclear relationship between global haemodynamics and liver perfusion, it is generally accepted that low cardiac output and hypotension result in hypoperfusion of the liver. Therefore, monitoring to allow proper maintenance and correction of cardiac output and perfusion pressure may include the use of a central venous catheter, pulmonary artery catheter and arterial catheter, depending on the expected haemodynamic instability and bleeding during the procedure. Also, it remains unclear whether monitoring of global oxygen delivery (DO2) can improve liver outcome. Finally transoesophageal echocardiography (TEE) helps in the early detection of liver outflow obstruction, allowing swift correction [27]; this does not mean that TEE should be used routinely during liver resection.
Microhaemodynamic Monitoring
Currently there are no microhaemodynamic monitoring tools available for routine clinical use. However, there are a few technologies that have been used experimentally, for example laser Doppler flowmetry, near-infrared spectroscopy, and in vivo fluorescence microscopy [28]. Although some of these are promising, none of these techniques is currently ready for clinical use.
Monitoring of Splanchnic Microcirculation
There may be a relationship between the microcirculation in sublingual and intestinal vascular beds during haemorrhagic shock [29, 30]. Preoperative abnormalities in sublingual microvascular flow seem to predispose to postoperative complications after major abdominal surgery, while global oxygen delivery or cutaneous tissue oxygenation did not correlate with the postoperative complication rate. It is unclear whether this monitoring technique has true value in monitoring liver tissue well-being. Gastric tonometry attempts to estimate gastric mucosal perfusion by determining the mucosal carbon dioxide partial pressure. However, outcome of clinical management based on gastric tonometry was no better than control, and the use of gastric tonometry has waned [31].
Markers of Liver Injury
Traditional Markers of Liver Injury
The most commonly used markers of liver injury include plasma concentrations of bilirubin, albumin, coagulation factors (the last one frequently assessed indirectly by PT and overall coagulation tests) and ‘liver enzymes’.
Hyperbillirubinaemia is a common marker of liver injury. Total bilirubin represents both conjugated (‘direct’) bilirubin and unconjugated (‘indirect’) bilirubin. Enzymes in the microsomes of hepatocytes convert unconjugated bilirubin into the more water-soluble conjugated bilirubin, allowing excretion in bile. Unconjugated hyperbilirubinaemia is seen in haemolysis or Gilbert syndrome, while conjugated hyperbilirubinaemia suggests cholestasis or hepatocellular dysfunction. Bile acids may be a better marker of hepatic biotransformation and cholestatic dysfunction than bilirubin [32, 33]. However, changes in bilirubin concentrations are not fast enough to detect intraoperative liver dysfunction.
Plasma concentrations of proteins synthesized by the liver do help in the assessment of liver function. However, since albumin has a half-life of about 21 days, it is not suited to detect acute liver dysfunction.
Because the half-life of clotting factors II, VII, IX and X is < 24 h, prothrombin time or INR could be a better marker of acute liver dysfunction. However, this one too may not be very helpful for the intraoperative determination of hepatic well-being because the half-lives of the clotting factors are still too long. Furthermore, coagulation changes can be induced by other factors, such as haemodilution, activation of the coagulation system (release of tissue factor) or activation of the fibrinolytic system. Therefore, changes in INR or in viscoelastic measures of coagulation (such as TEG and ROTEM) still don’t reflect acute changes in liver function intraoperatively.
The so-called liver function tests [such as aspartate aminotransferase or AST (not specific for liver damage), and alanine transaminase or ALT] reflect the release of these enzymes from damaged or necrotic hepatocytes. Another enzyme that is released with hepatocyte injury is lactate dehydrogenase, also a non-specific marker of ischaemic damage. However, the intraoperative and early postoperative determination of liver enzymes suffers from the same shortcomings as the ones previously mentioned: they reflect hepatocyte damage but provide no information about the function of the remaining hepatocytes. Thus, mild elevations of liver enzymes are common during and immediately after liver surgery but have little prognostic value.
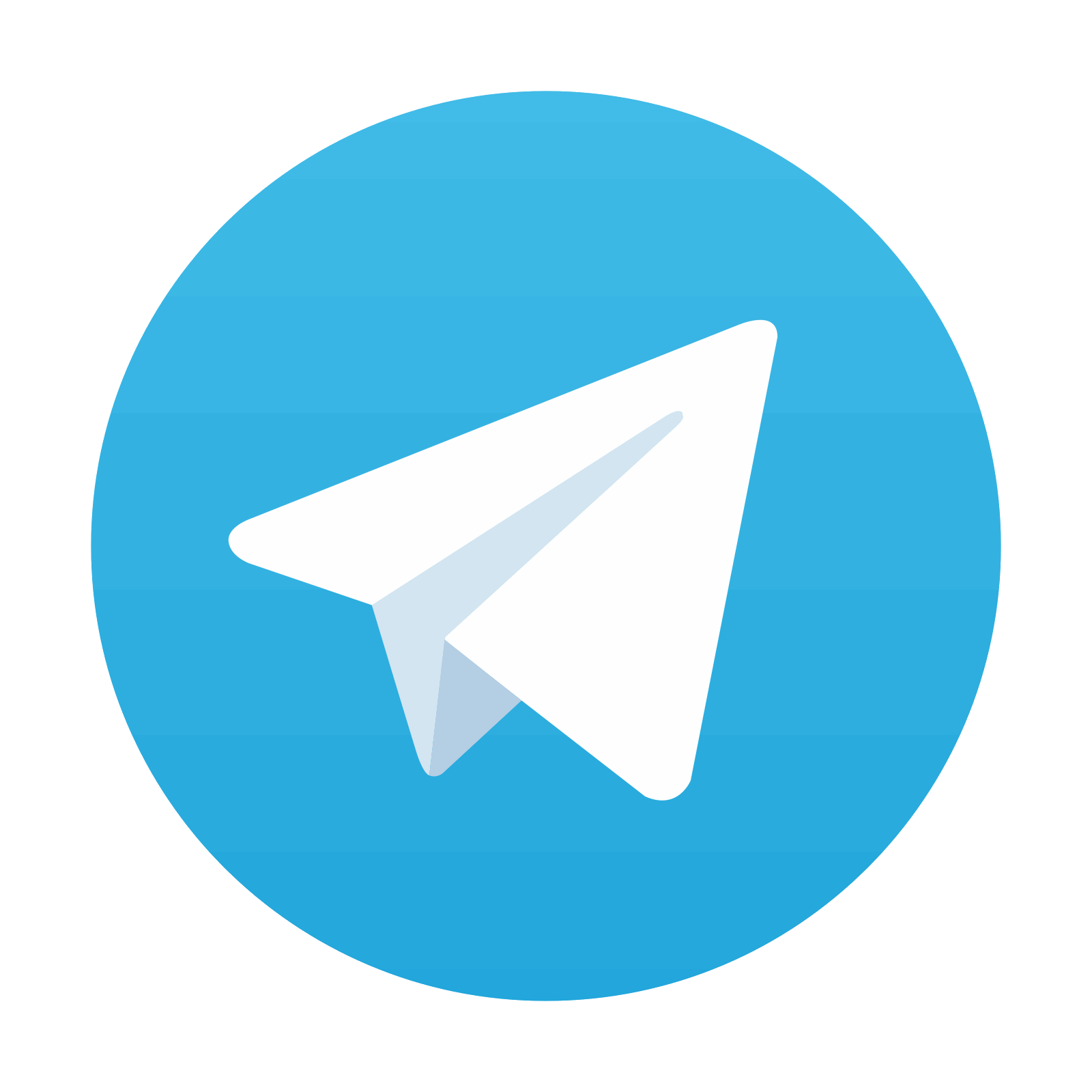
Stay updated, free articles. Join our Telegram channel

Full access? Get Clinical Tree
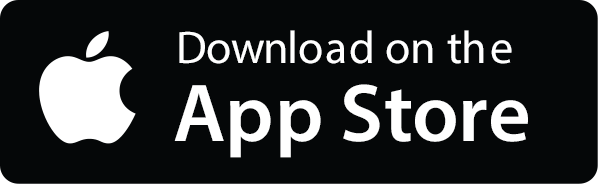
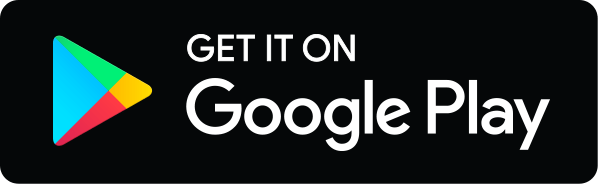
