Abstract
Because there is no disease condition that can be treated with the administration of anaesthetic medications, the specialty of anaesthesiology does not possess a curative effect in itself. Nevertheless, achieving the state of anaesthesia or the anaesthetic state relies completely on the use of drugs. Drugs used in anaesthesia are very powerful and able to transiently break the most deeply rooted physiological defence mechanisms. Some of the effects induced include lack of consciousness, absence of response to pain, absence of muscle tone, immobility, lack of breathing and dysfunction of the autonomic nervous system, to name just a few. Some of these effects might be considered target or ‘therapeutic effects’, such as unconsciousness, analgesia or immobility, but others are ‘side effects’ that are induced because of the relative low specificity of currently used anaesthetic drugs.
Introduction
Because there is no disease condition that can be treated with the administration of anaesthetic medications, the specialty of anaesthesiology does not possess a curative effect in itself. Nevertheless, achieving the state of anaesthesia or the anaesthetic state relies completely on the use of drugs. Drugs used in anaesthesia are very powerful and able to transiently break the most deeply rooted physiological defence mechanisms. Some of the effects induced include lack of consciousness, absence of response to pain, absence of muscle tone, immobility, lack of breathing and dysfunction of the autonomic nervous system, to name just a few. Some of these effects might be considered target or ‘therapeutic effects’, such as unconsciousness, analgesia or immobility, but others are ‘side effects’ that are induced because of the relative low specificity of currently used anaesthetic drugs.
Both the therapeutic and side effects are highly dynamic in their time course and they reach clinically effective ranges in a matter of seconds or minutes. If some of the collateral effects like respiratory depression are not adequately managed with measures such as securing the airway and providing mechanical ventilation, severe morbidity or even mortality might result. Fortunately, the current practice of anaesthesia relies on preoperative evaluation of patients as well as on several technological advances to try to predict and control the physiological changes in the patient. In addition, the effects of modern short-acting anaesthetic drugs fade very fast after discontinuing their administration.
It is of high importance to understand the fundamentals of pharmacology to be able to rationally dose anaesthetic drugs. Dosing has to be adjusted and individualized according to the characteristics of the patient, to the characteristics of the surgical or diagnostic procedure, and to the timing and events occurring during every procedure.
The present chapter will review basic concepts of clinical pharmacology. A quantitative perspective will be used. For instance, we will explain how mathematical models help understand drug effects, and how this will help us to define such concepts as onset of effect, peak effect and its time course, offset of effect after a bolus or continuous administration, context-sensitive decrement times, and the relevance of interindividual variability in anaesthetic drug response. These will serve as the basis for rational individualized drug dosing. Other chapters in this book will introduce topics such as estimating and characterizing inter-individual variability in drug response, incorporating covariate factor effects to decrease variability, signal analysis to measure actual responses to drugs with the goal of understanding, quantitating and individualizing drug responses in individual patients, for specific procedures, and for specific events during surgery.
The study of the principles governing the relation between dose and drug concentrations in the body constitutes pharmacokinetics (PK), and will be treated first. The relationship between drug concentration and effect will be presented in the pharmacodynamic (PD) concepts section.
Pharmacokinetic Concepts
Pharmacokinetics is the branch of pharmacology that studies the time course of the concentration of a drug resulting from initial drug dosing, its distribution to the different organs and tissues, and its disposal by the body through biotransformation and elimination. It also describes how it is absorbed when the drug is not intravenously administered. In real life, these processes occur simultaneously. The fundamental PK concepts are volume of distribution and clearance, the basic building blocks of the compartment models that are used to explain PK and PD of intravenous drugs (Fig. 1.1). For inhaled agents, physiological models are most often (but not exclusively) used.
Fig. 1.1 Classical compartment model description. The left panel shows a three-compartment pharmacokinetic model. Drug input is marked by the red arrow and clearance, metabolic and intercompartmental, by the green arrows. The right panel shows a PK model with the addition of an effect site in the form of a long blue rectangle with no volume to meet the assumptions required for the modelling approach (generated with the use of the Cylinders software available at www.pkpdtools.com).
Volume of Distribution
The volume of distribution describes the relationship between the amount of drug in the body and its blood concentration [1]. When a bolus dose of any drug is administered intravenously, the drug gets diluted by and in the blood. It can be seen as analogous to introducing a substance into a bucket of water or fluid where physical laws regulate the speed and degree of dissolution. However, in the case of drugs and tissues, the degree to which a substance is diluted or how it reaches the tissues will also depend on drug properties such as lipophilicity, protein binding, partitioning into tissues and the flow of blood to the different organs and tissues.
Once the drug is in the fluid, which most often is the blood within the context of anesthesia, its presence can be measured as a concentration. If the concentration of a drug and the amount of drug administered are known, the volume of distribution can be estimated:
A volume of distribution is an apparent volume, i.e. a calculated value that does not have to bear a resemblance to any anatomical entity; it does not need to be equal to the volume of tissues in the body. For example, if a drug is highly bound to tissues, its concentration in blood will be very low, and therefore the estimation of the distribution volume can be very high, higher than any rational estimate of human body volume.
Volume of Distribution in the Central Compartment versus Peripheral Compartments
The central compartment is defined as the compartment into which a drug is initially injected and from which samples are taken for measurement. In the context of anaesthetic drugs, it is usually the volume of blood contained in the heart and blood vessels. The volume of distribution of the central compartment is the ratio of the administered dose over the first measured drug concentration. The ‘maximal’ concentration, i.e. at the time of injection, is obtained by back extrapolation of the concentration–time curve to time zero. The ‘volume of distribution’ concept is calculated with this concentration, which obviously is a theoretical construct because there is not yet a single drug molecule in the circulation at time zero because it takes some time for the drug to be transported from the vein to the central circulation.
There are several factors that affect estimation of the volume of distribution of the central compartment. Some are related to study design, for instance the sampling site and the timing of the samples. The sampling site, venous versus arterial blood, may affect the estimation because the concentration is lower in the venous than in the arterial blood: the estimation of volume of distribution based on venous blood samples thus will be significantly larger than that based on arterial blood. Timing of the first sample also has an effect: the longer it takes to obtain the first sample, the lower the ‘initial’ concentration will be because distribution and elimination start immediately after the drug enters the body, and thus the larger the calculated volume of distribution will be, leading to an overestimate. Erroneous distribution volume estimates will have consequences when they are going to be used for dosing guidelines because the recommended dose to achieve a given concentration will be much larger if the volume of distribution has been overestimated. This will result in excessively high actual concentrations. It also underscores the need for prospective testing of a model.
While it is known that several anaesthetic drugs undergo some initial distribution and degradation in the lungs, estimates of central volume do not usually take this into account. The study and integration of the changes taking place during the initial phase of mixing and distribution of drugs in the blood, its first-pass through the lung circulation, and possible degradation of drugs by the lungs is what is known as ‘front-end kinetics’. Front-end kinetics has been studied and characterized, and more complex models have been proposed that could be implemented in infusion devices [2–4].
Depending on the characteristics of drugs and their ability to reach peripheral tissues, compartments other than the central compartment might be added and estimated. For most anaesthetic drugs it is assumed that the drug permeates rapidly from the central compartment to a group of highly perfused tissues, considered to be a fast peripheral compartment, and more slowly to a group of poorly perfused tissues, a slow peripheral compartment, mainly composed of fat. A drug that has reached fat tissues slowly will also slowly return to the central compartment. However, the possibility of a drug returning from fat to blood in significant quantities is very unlikely with the rapidly acting drugs that we use nowadays, therefore the probability of significant side effects related to significant amounts of drug back from fat to blood is very low.
To determine whether a one, two, or three compartment model best describes the time course of drug concentration, an adequate number of blood samples has to be drawn at predefined times, otherwise the whole PK process may not be accurately described.
At this point it is important to remark that PK not only describes the relation between dose and concentration of drugs, but that it is an important driver for pharmacological effect over time. Volumes of distribution and clearances not only have a combined influence on the PK, but also on the time course of the effect of each drug. This can be seen in Fig. 1.2 where a larger volume of distribution slows the onset of effect and decreases the duration of effect as well.
Clearance
Clearance describes the relation between the concentration of a drug and the rate of elimination of the drug from the body. Clearance reflects a real physiological phenomenon, namely the ability of the body to eliminate an external substance, in this case a drug. It is measured in flux units (volume per time unit, i.e. litres/hour), and it describes how many litres of blood are irreversibly cleared of the drug in one hour.
Intuitively, clearance can be compared to a vacuum cleaner absorbing dust from the air in a room: clearance deals with the ability to clear ‘the solvent (air or plasma)’ from ‘the solute (dust or drug)’ per unit of time rather than with the amount of the solute (dust or drug) that is being removed. It can apply to a single organ (hepatic clearance for instance) or to the whole body (sometimes referred to as ‘metabolic clearance’) [5].
Clearance describes the intrinsic ability of the body to extract drugs from blood or plasma – it is not the rate of elimination or removal of the absolute amount of the drug per time unit. The amount of drug eliminated will depend on the concentration of the drug present in the blood at any moment. If drug clearance is constant at 1 L/h, the drug elimination rate will be zero if there is no drug but would be 1 mg/h if the concentration is 1 mg/L, and 100 mg/h if the concentration is 100 mg/L. Clearance is the proportionality constant that relates the rate of elimination to the measured concentration.
Clearance parameters, expressed in units of flow (L/min or L/kg/min), simply quantify the volume of plasma from which the drug is completely cleared per unit of time. Clearance is constant and independent of drug concentration for drugs that have a so-called linear behaviour meaning that doubling the dose will result in doubling of the drug concentration.
Clearance has been extensively used to calculate drug dosing schemes:
Age affects clearance: as adults grow older, their capacity to clear a drug is also decreasing. Organ failure can also affect the clearance by that particular organ. Concomitant drugs can also affect the clearance of a subject, for instance by altering cardiac output or simply by interfering with metabolic mechanisms. The two main organs with clearance ability are the liver and the kidneys.
As mentioned before for volume of distribution, clearance is a key component of the PK of any drug, but it also has an important influence on the time course of effect. Figure 1.3 presents the predicted effect-site concentration of propofol with normal and with doubled (metabolic) clearance to illustrate the effect of PK changes on drug effect.
Hepatic Clearance
From a clearance point of view, the liver is the most important organ. The liver clears drugs from the blood or the plasma. Two different drug classes are discerned, those with a high and those with a low extraction ratio. The first class are those drugs for which the only limiting factor for hepatic clearance is the flow of blood to the organ, and these drugs are said to have a high ‘extraction ratio’, close to one, as is the case for propofol. Drugs in the second class are those where the ability of the liver to clear blood or plasma is limited by the function of the hepatocyte, due to its internal enzymatic mechanisms or because some drug characteristics make it difficult to enter the cell. Drugs with these characteristics are said to have a low extraction ratio (well below one). Alfentanil is a good example of this class of drugs.
Renal Clearance
Renal clearance is composed of two different mechanisms: glomerular filtration, and secretion at the tubular level. As with any other organ, ageing affects kidney function and consequently drug clearance, causing drug concentrations after the same dose to be higher and thus causing their effects to last longer. Some anaesthetic agents undergo liver biotransformation to generate water soluble compounds that can be excreted through the kidney. A classical, now historical, example is the neuromuscular blocking agent pancuronium, 85% of which is excreted by the kidneys. Many anaesthetic drugs also alter kidney function by altering renal blood flow.
Distributional Clearance
Distributional clearance reflects the transfer of a drug from the central volume of distribution, blood or plasma, to peripheral tissues. It will depend on the blood flow to each tissue or organ and on the permeability of capillary walls to different drugs. For propofol, a highly lipophilic hypnotic drug, the sum of metabolic clearance plus distribution clearance is almost as high as cardiac output, and this is because it is avidly captured by peripheral tissues. When it comes to drugs undergoing plasma enzymatic metabolism, such as remifentanil or Dynorphin A 1–13, the sum of metabolic and distributional clearance is higher than cardiac output. Distributional clearance is responsible for terminating a drug’s effect after the initial bolus.
Compartment Models Applied to Pharmacology
When a bolus of a drug is administered and blood samples are collected to quantitate plasma drug concentrations, a certain concentration decay pattern can be observed. This pattern is similar when the same drug dose(s) and blood sampling sequence are repeated in a number of individuals. As can be seen in Fig. 1.4, Panel A, there is a common trend or tendency: there is a fast decay after the initial administration that subsequently slows down. Panel B represents how there is a common pattern for all blood samples from all individuals studied: three different decay parts can be visually identified in the curves, especially when a semi-log representation is used (Panel C). This pattern (Panel D) can be represented by an equation of the form:
Fig. 1.4 Constructing a PK model. Panel A shows the time course of concentrations for a single subject. Panel B shows the common time course pattern for six individuals. If the results are plotted on a graph with a semi-logarithmic concentration on the Y-axis, three different decay phases can be observed in Panel C. Panel D shows the typical curve describing the time course of drug concentrations for all individuals and for the curve representative of the population or ‘typical’ individuals.
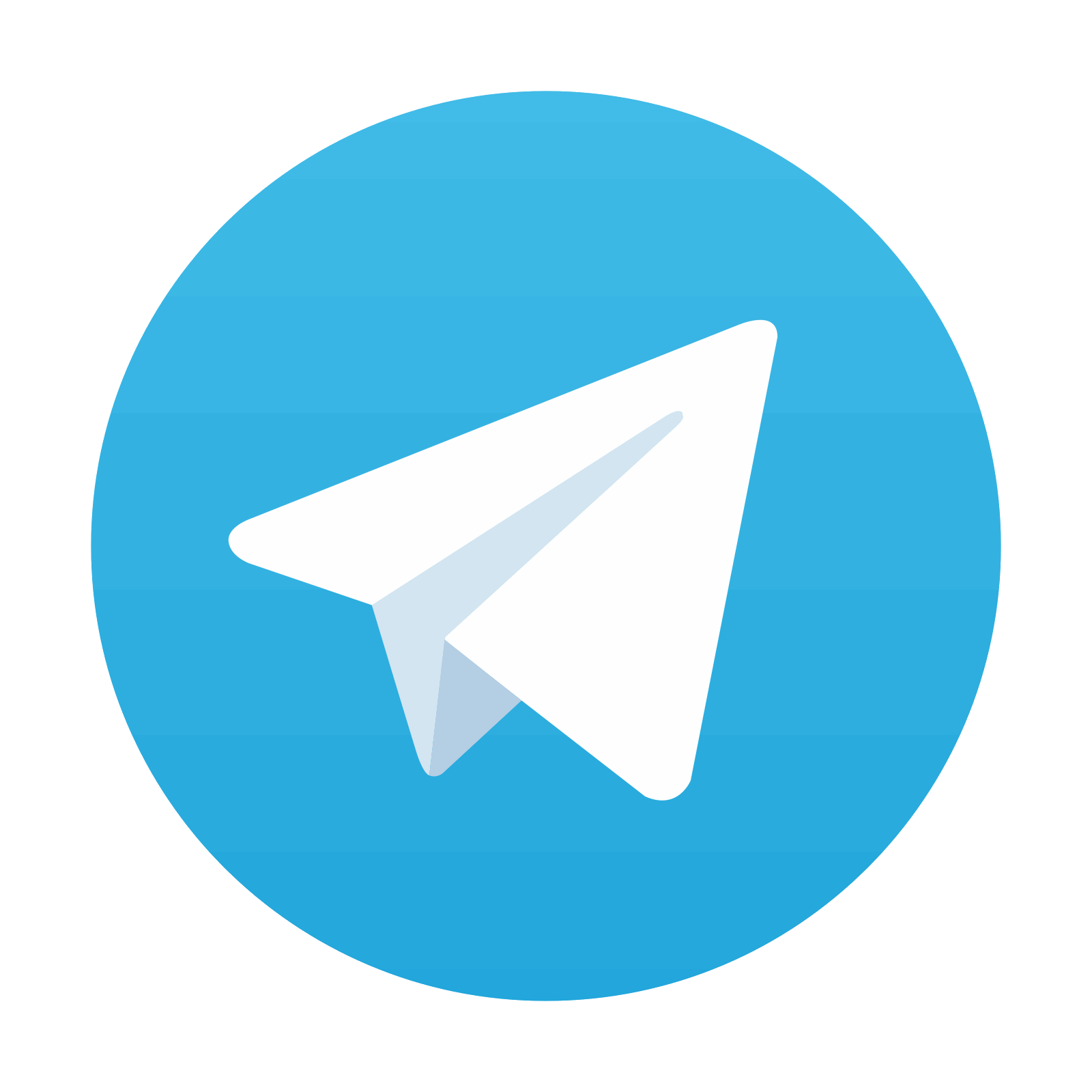
Stay updated, free articles. Join our Telegram channel

Full access? Get Clinical Tree
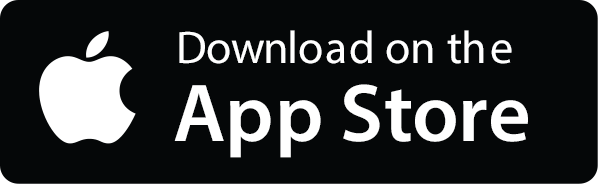
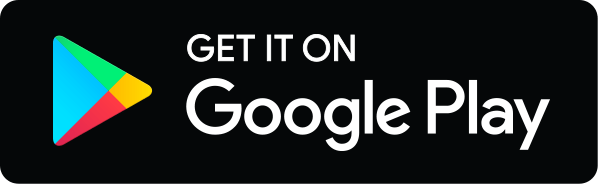
