Chapter 11
Pain pharmacology and the pharmacological management of pain
OVERVIEW
In the general population, the sensation of pain is generally perceived in a negative connotation because of its association with physical damage to the body and its unpleasant affective quality (Apkarian 2008). This perception is reinforced by the International Association for the Study of Pain (IASP) definition of pain as ‘an unpleasant sensory and emotional experience associated with actual or potential tissue damage, or is described in terms of such damage’ (Merskey & Bogduk 1994).
Pain detection and signalling involve a complex cascade of events. Nociceptors are free nerve endings that detect potentially damaging mechanical, electrical, thermal and chemical stimuli, resulting in the generation of action potentials (Woolf & Ma 2007). These action potentials are transmitted predominantly via first-order neurons (Aδ and C fibres) to laminae I and II of the dorsal horn of the spinal cord, from where they are relayed by second-order neurons via spinothalamic tracts to higher centres in the brain (Sherrington 1906). This nociceptive input may in turn activate descending inhibitory pathways to reduce the severity of the perceived pain (Sherrington 1906). Levels of pain reported by patients comprise nociception and interpretation of its emotional significance by the anterior cingulate cortex (Rainville et al 1997).
It is well understood that the pathobiology of human pain encompasses a mosaic of biological and psychological phenotypes that are underpinned by multiple contributing factors (Diatchenko et al 2006). However, despite great advances in our collective understanding of the neurobiology of chronic pain over the last two decades, with the identification of a vast array of receptors, ion channels and enzymes as potential novel drug targets, translation of this knowledge into new pain medicines for clinical use has been painstakingly slow. For this reason, the medications currently available for prescribing by frontline clinicians for the pharmacological treatment of pain in patients are similar to those available a decade ago.
Pain classification
Pain may be classified according to a number of different criteria, including duration (acute and chronic), type (nociceptive, inflammatory and neuropathic) and severity (mild, moderate and severe); the corresponding definitions are given in Table 11.1.
Nociceptive pain
Physiological or nociceptive pain is part of an early warning system that has evolved to instruct motor neurons of the central nervous system to produce actions that minimize further injury after detection of physical harm (Zhuo 2007). Examples of acute nociceptive pain include post-operative pain and pain following trauma with pain resolving as healing takes place.
Inflammatory pain
Inflammatory pain occurs in response to tissue injury and sensitization of nociceptors by a range of pro-inflammatory mediators that are released at the injury site. For acute inflammatory conditions, inflammatory pain resolves once the initial tissue injury heals (Reichling & Levine 2009). However, in chronic inflammatory disorders such as rheumatoid arthritis and visceral pain conditions such as chronic pancreatitis, the pain persists while inflammation is active (Michaud et al 2007).
Neuropathic pain
Neuropathic pain is a type of pain that occurs after injury to either peripheral nerves or the sensory pathways in the spinal cord or brain. It resembles inflammatory pain in that spontaneous pain and hypersensitivity are usually present, but the underlying disease pathology is in the nervous tissue (Jensen & Finnerup 2009).
Peripheral sensitization
Following tissue and/or peripheral nerve injury, multiple chemical mediators are released, forming an ‘inflammatory soup’ comprising cytokines, growth factors, kinins, hydrogen ions, ATP, serotonin, histamine, neuropeptides and prostaglandins. This results in an inflammatory response and sensitization of various components of the somatosensory system (Fig. 11.1) (Thacker et al 2007). Following peripheral sensitization, there is neuronal hyperexcitability, resulting in ectopic discharge of primary afferents and the development of so-called ‘central sensitization’ in the spinal cord. The net result is that innocuous stimuli are detected as painful (allodynia) and/or there is a heightened response to painful stimuli either at the site of injury (primary hyperalgesia) or extending into the surrounding uninjured tissue (secondary hyperalgesia) (Vanderah 2007).
Fig. 11.1 Schematic diagram summarizing neuronal and non-neuronal mechanisms implicated in the pathobiology of chronic pain.
Multiple receptors and ion channels have been identified on the peripheral terminals of primary afferent nerve fibres, including receptors for serotonin (5-HT), bradykinin (B1 and B2), histamine, nerve growth factor (TrKA), ATP (purinergic), epinephrine, prostaglandin E2 (PGE2), tumour necrosis factor alpha (TNF-α) and interleukins (IL) as well as sodium channels, acid-sensing ion channels (ASIC) and vanilloid (TRPV1) receptors (Basbaum et al 2009; Coggeshall & Carlton 1997; Sato et al 1993; Woolf & Mannion 1999). Nociceptor hyperexcitability develops after exposure to the ‘proinflammatory soup’ of chemical mediators released from damaged blood vessels, nerve terminals and immune cells that accumulate at the site of injury. This in turn results in activation of multiple receptors and ion channels to induce and maintain peripheral sensitization (Reichling & Levine 2009). Enhanced excitability of peripheral nerves with lowered activation thresholds and/or increased action potential frequency secondary to inflammation-induced peripheral sensitization is transduced intracellularly by kinase pathways including mitogen activated protein kinase (MAPK) such as p38 MAPK and extracellular signal related kinase (ERK) (Crown et al 2008; Ji 2004; Zhuang et al 2005), protein kinase A (PKA) (Bhave et al 2002) and protein kinase C (PKC) (Bhave et al 2003). Activated kinases contribute to peripheral sensitization through phosphorylation and activation of multiple receptors and ion channels (Reichling & Levine 2009).
As neuropathic pain persists after ganglionectomy involving removal of the injured afferent soma, it is clear that maintenance of neuropathic pain is not dependent solely on changes that occur in injured afferents (Sheth et al 2002). A hallmark of neuropathic pain is ectopic activity in damaged primary afferents underpinned by accumulation and dysregulated function of voltage-gated sodium channels after peripheral nerve injury (Costigan et al 2009). This aberrant activity spreads rapidly to the cell bodies in the dorsal root ganglia to generate ectopic activity in adjacent undamaged sensory afferents (ephaptic transmission), which in turn leads to an expansion of the perceived painful area (receptive field). Furthermore, sympathetic efferents have been shown to activate sensory afferents via α-adrenoceptors, with these interactions between adjacent sensory and autonomic afferents and ganglion cells providing a mechanism for the spread of ectopic discharge between nerve fibre types (Zhang & Strong 2008).
More recently, satellite glial cells (SGCs), which form complete sheath-like structures around sensory neurones in the DRG, have been shown to respond to pro-inflammatory agents including ATP, nitric oxide and bradykinin (Hanani 2005). SGCs are implicated in nociceptive signalling as they express a broad range of transmitter molecules (e.g. somatostatin, NGF, BDNF, GDNF and IL-6), as well as receptors (e.g. trkA, bradykinin B2 receptors, orexin-1, somatostatin, TNF-α type-1, IL-1 and peripheral benzodiazepine receptor) and ion channels (e.g. inwardly rectifying K + channels) (Takeda et al 2009). Hence, SGCs are proposed to have a chemosensory role and contribute to sensory neurotransmission after tissue or peripheral nerve injury (Hanani 2005; Ohara et al 2009; Takeda et al 2009).
Central sensitization
So-called central sensitization that develops at spinal and supraspinal levels of the central nervous system (CNS) secondary to persistent inflammation or nerve injury is underpinned by multiple factors (Latremoliere & Woolf 2009). These include increased responsiveness of nociceptive neurons to normal or subthreshold afferent input, changes in the expression of receptors, ion channels and enzymes, changes in the properties of inhibitory interneurons, and activation of descending inhibitory as well as opposing descending facilitatory mechanisms (Fig. 11.1; Latremoliere & Woolf 2009; Urban et al 1999; Vanegas & Schaible 2004). Furthermore, recent research implicates activated non-neuronal cells in the development and maintenance of the central sensitization of neurons in the CNS (Vallejo et al 2010; Watkins et al 2007).
Mechanisms underpinning central sensitization include persistent activation of the N-methyl-D-aspartate (NMDA)-nitric oxide synthase (NOS)-nitric oxide (NO) signalling cascade, upregulation of sodium channels and acid-sensing ion channels, as well as TRPV1, TRPM8 and α-receptors (Baron 2009; Costigan et al 2009; Harvey & Dickenson 2008). Other mechanisms include enhanced dynorphin signalling at supraspinal levels of the CNS (Lai et al 2001) as well as degeneration of inhibitory GABAergic interneurons in the spinal cord to cause disinhibition and increase sensitivity (Scholz et al 2005).
Under normal homeostatic conditions, non-neuronal cells such as microglia and astrocytes have important ‘house-keeper’ roles to support the ongoing function and survival of neurons in the CNS. Microglia comprise 5–10% of glia in the CNS (Watkins et al 2007) and their major function is immune surveillance (Raivich 2005). Astrocytes comprise 40–50% of all glial cells in the CNS to provide trophic support, energy and neurotransmitter precursors to neurons, regulation of extracellular concentrations of various ions and neurotransmitters, as well as neurite outgrowth, formation of synapses, neuronal differentiation and survival (Perea & Araque 2005). However, following activation, microglia and astrocytes release a range of pronociceptive substances, including cytokines, chemokines, neurotrophic factors, ATP, excitatory amino acids and nitric oxide, that enhance pain by increasing the excitability of nearby neurons (Fig. 11.1) (Vallejo et al 2010). Recent studies also implicate a role for activated microglia and astrocytes in the development of analgesic tolerance and opioid-induced hyperalgesia that may develop following chronic morphine administration (Wang et al 2010).
Nociceptive neurotransmitters and their target receptors
Factors contributing to the pathophysiology of persistent pain include pro-inflammatory mediators released in response to nerve injury, transcriptional changes at the level of the dorsal root ganglia, phenotypic changes in neural pathways, activation of glial cells in the nervous system, structural modifications including nerve sprouting and neurodegeneration of GABAergic neurons in the CNS, resulting in a hyperexcitable nervous system (Basbaum et al 2009). Specific molecular interactions that drive the aforementioned changes in neuronal excitability may differ according to the specific injury and the consequent chemical environments that are created (Basbaum et al 2009). The molecular interactions involve all major families of regulatory proteins, including G-protein coupled receptors (GPCRs), ion channels, enzymes, neurotrophins and kinases, thereby offering an abundance of potential analgesic targets and therapeutic opportunities (Stone & Molliver 2009). Examples include glutamate (Mayer 2005), GABAergic (Goudet et al 2009), neurokinin (Seybold 2009), calcitonin gene-related peptide (Seybold 2009), bradykinin (Dray 1997), prostanoid (Zeilhofer 2007), purinergic (Teixeira et al 2010), protease (Vergnolle et al 2003), neurotrophin (Hefti 1997), opioid (Snyder & Pasternak 2003), cannabinoid (Brown 2007), cytokine (Uceyler et al 2009), chemokine (Abbadie et al 2009) and transient receptor potential vanilloid receptors (Broad et al 2009), as well as sodium (Bhattacharya et al 2009), calcium (Yaksh 2006) and acid-sensing ion channels (Sluka et al 2009).
Despite the aforementioned large number of receptors and ion channels serving as potential targets for the development of a range of novel pain therapeutics, these are yet to reach the clinic. Hence, pain will continue to be managed with the currently available medications according to the principles succinctly encapsulated by the World Health Organization’s three-step Analgesic Ladder (World Health Organization 1986).
MAJOR GOALS FOR THE PHARMACOLOGICAL TREATMENT OF CLINICAL PAIN
1. Increase inhibitory neurotransmission to provide analgesia by decoupling the response between an acute noxious stimulus (e.g. post-surgery or trauma) and the painful sensation that would normally be evoked (Doubell et al 1999).
2. Prevent development of peripheral or central sensitization under circumstances where this would normally occur (Doubell et al 1999).
3. Restore the normal responsiveness of the nociceptive signalling system in patients suffering from states of either hypo- or hypersensitivity so that responses to defined low- or high-intensity stimuli are perceived correctly as innocuous or painful sensations, respectively (Doubell et al 1999).
PHARMACOLOGICAL TREATMENT OF PAIN
WHO analgesic ladder
Almost a quarter of a century after its introduction in 1986 to guide the pharmacological management of chronic cancer pain, the WHO three-step analgesic ladder (Fig. 11.2) is now widely used to more broadly guide the pharmacological treatment of both acute and chronic pain.
According to step 1 of the analgesic ladder, non-opioid analgesics, including paracetamol (acetaminophen), non-steroidal anti-inflammatory drugs (NSAIDs, e.g. aspirin and ibuprofen) and coxibs (e.g. celecoxib), are recommended for the treatment of mild pain. Adjuvants (e.g. tricyclic antidepressants, anticonvulsants and anti-arrhythmics) may be co-administered if pain has a neuropathic component. When mild pain progresses to moderate pain (step 2), weak opioid analgesics such as codeine, tramadol and dextropropoxyphene are added to non-opioids with adjuvants co-administered for pain with a neuropathic component. For moderate to severe pain (step 3), strong opioid analgesics, including morphine, oxycodone, hydromorphone and fentanyl, are recommended. Morphine is recommended by the WHO as the strong opioid analgesic of choice due to its worldwide availability at low cost. Strong opioid analgesics may be co-administered with non-opioids and/or adjuvants, as required (World Health Organization 1986).
The WHO guidelines also recommend that patients receive individualized dose titration to ensure an adequate dosage of the selected analgesic and/or adjuvant with drug treatment administered on a scheduled ’round the clock’ basis rather than ‘as required’ (World Health Organization 1986). Controlled-release and sustained-release formulations of opioid analgesics allow the convenience of once or twice-daily dosing, which improves patient compliance and analgesic outcomes. For the treatment of breakthrough pain, such as that which occurs during dressing changes or due to incident pain upon mobilization, additional bolus doses of immediate-release opioid analgesic formulations may be administered on an ‘as required’ basis.
Whilst the oral dosing route is preferred for most patients, it is not practical during labour or in the immediate post-operative period due to impaired gastrointestinal transit. In circumstances where more rapid pain relief is required or there is inadequate pain relief and intolerable side effects, such as severe vomiting, severe dysphagia or bowel obstruction, changing the route of administration to parenteral (e.g. intravenous, subcutaneous, intramuscular), rectal, buccal, sublingual, transdermal or spinal (epidural, intrathecal) may restore adequate analgesia with tolerable adverse effects (Walsh 2005). Another strategy for restoring satisfactory analgesia with tolerable side effects involves ‘opioid rotation’ by switching from the first opioid to another (Walsh 2005).
Recently, modifications to the original WHO analgesic ladder have been proposed. For example, Eisenberg et al suggested that invasive procedures such as neurolytic blocks should be considered for patients experiencing inadequate pain relief or intolerable side effects as an adjunct or alternative to pharmacotherapy (Eisenberg et al 2005). More recently, Riley et al proposed the addition of two steps to the original WHO three-step analgesic ladder (Fig. 11.3), with the fourth step recommending use of ‘opioid rotation’ for patients experiencing inadequate pain relief and intolerable side effects; if opioid rotation fails, progress to a fifth step involving use of anaesthetic intervention is recommended (Riley et al 2007).
Fig. 11.3 Overview of proposed five-step WHO analgesic and side-effect ladder. Reprinted from Riley J, Ross J R, Gretton S K, A’hern R, Du Bois R, Welsh K, Thick M 2007 Proposed 5-step World Health Organization analgesic and side effect ladder. European Journal of Pain Supplements 1:23–30, with permission of John Wiley & Sons.
ANALGESIC AGENTS
Non-opioid analgesics
A summary of commonly used non-opioid analgesics and their dosing schedules is provided in Table 11.2.
Table 11.2
Clinically available non-opioid analgesics and their dosing schedules
(adapted from (AHRQ 2009)
Paracetamol
Paracetamol (acetaminophen) is a non-opioid analgesic that is widely utilized for the symptomatic relief of fever, headaches and minor aches and pains (Remy et al 2006). Paracetamol is an active ingredient in a large number of pharmaceutical preparations, including over-the-counter cold and flu products as well as prescription medicines (Munir et al 2007). Paracetamol produces pain relief and antipyretic actions after oral administration of usual adult doses of 1 g three or four times daily but not exceeding a total daily dose of 4 g (Myers & LaPorte 2009). In contrast to NSAIDs, paracetamol does not inhibit platelet aggregation and does not damage the gastric mucosa (Munir et al 2007; Remy et al 2006). A recent systematic review concluded that single 1 g doses of paracetamol provide effective analgesia for up to 4 hours in ~50% of patients with acute post-operative pain. In addition, patients reported few, mainly mild, adverse effects whose severity and frequency were similar to those reported by patients administered placebo (Toms et al 2008).
Although paracetamol readily crosses the blood–brain barrier, the mechanism through which it produces its analgesic and antipyretic effects is unclear. Proposed mechanisms include modulation of serotoninergic signalling, a central cyclooxygenase-3 (COX-3) mechanism and even a cannabinoid-mediated mechanism (Bonnefont et al 2003; Myers & LaPorte 2009; Pickering et al 2006). The poor ability of paracetamol to inhibit COX-1 and COX-2 and its central model of action likely underpins its lack of anti-inflammatory efficacy (Lee et al 2004; Shamoon & Hochberg 2000).
Paracetamol adverse effects
The most serious adverse effect of paracetamol is potentially fatal liver toxicity, which may occur if therapeutic doses are exceeded. Hepatotoxicity is mediated by a reactive metabolite, N-acetyl-p-benzoquinoneimine (NAPQI), which normally accounts for only 5–8% of a therapeutic dose of paracetamol and is detoxified by conjugation with glutathione in the liver (Larson et al 2005; Whitcomb & Block 1994). However, if the amount of NAPQI formed exceeds liver stores of glutathione, then hepatotoxicity will occur, potentially resulting in liver failure. In the presence of glutathione depletion due to starvation, alcohol abuse or impaired liver function, the daily dosage of paracetamol should not exceed 3 g due to the increased risk of hepatotoxicity (Food and Drug Administration 1999; Larson et al 2005; Remy et al 2006; Whitcomb & Block 1994).
If a patient seeks medical attention soon after paracetamol overdose, activated charcoal can be used to decrease paracetamol absorption. The mainstay of treatment involves administration of acetylcysteine, a precursor for glutathione in an endeavour to increase glutathione production sufficiently to prevent liver failure. However, if liver damage is severe, then a liver transplant will be required. In the USA alone, almost half of the acute liver failure cases and a third of deaths are due to paracetamol overdose induced liver toxicity (Larson et al 2005).
Oral non-steroidal anti-inflammatory drugs
NSAIDs are chemically diverse compounds that have analgesic, antipyretic and anti-inflammatory properties, and are amongst the most widely prescribed drug products globally (Dugowson & Gnanashanmugam 2006). There are multiple chemical classes of NSAIDs, including salicylates (aspirin, methyl salicylate, diflunisal), arylalkanoic acids (indometacin, sulindac, diclofenac), the ‘profens’ or 2-arylpropionic acids (ibuprofen, naproxen, ketoprofen, ketorolac) and oxicams (piroxicam, meloxicam).
NSAIDs inhibit the synthesis of prostaglandins, endogenous molecules that have diverse paracrine effects, including pronociceptive signalling, as well as modulation of inflammation and temperature regulation in the hypothalamus (Zeilhofer 2007). With the exception of aspirin, NSAIDs produce their anti-inflammatory and analgesic effects by competitive inhibition of the two isoforms of the enzyme cyclooxygenase, COX-1 and COX-2, to inhibit formation of prostaglandins and thromboxane from arachidonic acid (Vane 1971).
COX-1 is constitutively expressed in platelets, the gastrointestinal tract and the kidneys, whereas COX-2 is inducible and found in the kidneys and the CNS (Zeilhofer 2007). The desired anti-inflammatory action of NSAIDs is largely due to inhibition of COX-2, whereas aspirin irreversibly acylates both COX-1 and COX-2 (Dugowson & Gnanashanmugam 2006). Although classified as mild analgesics, NSAIDs are effective for the treatment of pain involving peripheral tissue sensitization (Burke & Fitzgerald 2006) and they have opioid-sparing effects to reduce post-operative opioid consumption, resulting in reduced opioid-related adverse events (Marret et al 2005).
PGE2 release in the hypothalamus is responsible for triggering an increase in body temperature during inflammation (Zeilhofer 2007) and so NSAIDs produce their antipyretic effects secondary to inhibition of PGE2 formation in the hypothalamus. At doses as low as 30 mg/day, aspirin irreversibly inhibits COX-1-dependent formation of thromboxane A2 in platelets to inhibit platelet aggregation with an 8–12 day duration of action, i.e. the turnover time of platelets; it is this action that underpins the cardioprotective effects of aspirin (Munir et al 2007).
Adverse effects of NSAIDs
The most common adverse effects of NSAIDs are gastrointestinal, renal and respiratory. All NSAIDs, including coxibs, can cause or aggravate hypertension, congestive heart failure, edema and kidney problems (Agency for Healthcare Research and Quality 2009).
Prostaglandins, whose synthesis is catalysed by COX-1, have a cytoprotective effect in the gastric mucosa and so NSAID-induced inhibition of COX-1 may result in dyspepsia, gastric irritation, ulceration and bleeding as well as diarrhoea (AHRQ 2009). Although most NSAIDs show little selectivity between COX-1 and COX-2, selectivity is seen at low doses for some agents. For example, at relatively low doses such as 7.5 mg daily, meloxicam is a preferential inhibitor of COX-2 (Munir et al 2007).
Prostaglandins have an important role in maintaining normal glomerular perfusion and filtration rates through their vasodilatory effects on afferent arterioles of the glomeruli (AHRQ 2009). In renal failure, where the kidney is trying to maintain renal perfusion pressure by elevated angiotensin II levels that constrict the afferent arteriole into the glomerulus in addition to the efferent arteriole, prostaglandins have a protective affect on the afferent arteriole (AHRQ 2009). If this is blocked by NSAID administration, there is decreased renal perfusion pressure, resulting in renal toxicity (AHRQ 2009).
It is important to also be aware that aspirin-exacerbated respiratory disease presenting as rhinitis and asthma occurs in ~10% of the general population and in ~21% of adults when determined by an oral provocation testing (Dugowson & Gnanashanmugam 2006). Hence, NSAIDs should be avoided in patients with known aspirin sensitivity.
In terms of NSAID-induced adverse effects, it is important to recognize the following (AHRQ 2009):
1. NSAIDs increase the risk of gastrointestinal bleeding at higher doses; people older than 75 years have the highest risk.
2. NSAIDs should be avoided in people receiving anticoagulant therapy.
3. Consider using paracetamol instead as it is associated with a lower risk of gastrointestinal bleeding.
4. Co-administration of the PGE1 analogue misoprostol or proton pump inhibitors in conjunction with NSAIDs can prevent duodenal and gastric ulceration (Rostom et al 2002).
5. Celecoxib, ibuprofen at doses of 800 mg three times a day and diclofenac (75 mg twice a day) have increased risk of myocardial infarction. Naproxen does not increase the risk of myocardial infarction even at a dose of 500 mg twice a day.
Topical NSAIDs
Topical NSAIDs are often used to treat acute musculoskeletal conditions due to the potential of this delivery route to provide pain relief whilst minimizing the incidence of systemic adverse events (Massey et al 2010). Clinical trials show that topical NSAIDs are effective and relatively safe for the treatment of osteoarthritic pain, with fewer systemic side effects compared with oral NSAIDs (Altman et al 2009; Barthel et al 2009; Simon et al 2009). In a recent systematic review of 47 clinical studies that compared various topical NSAIDs in a range of formulations, including sprays, gels and creams, for the treatment of acute pain relative to the corresponding placebo preparations, the number needed to treat for clinical success (50% pain relief) was 4.5 for treatment periods in the range 6 to 14 days (Massey et al 2010). Topical preparations of ibuprofen, diclofenac, ketoprofen and prioxicam were of similar efficacy but indometacin and benzydamine were not significantly better than placebo. There were very few systemic adverse events and adverse-event-related withdrawals (Massey et al 2010).
Coxibs
Coxibs are selective COX-2 inhibitors that were developed to avoid the gastric irritation of NSAIDs due to their COX-1 inhibitory effects, whilst retaining equivalent analgesic properties to NSAIDs (Dugowson & Gnanashanmugam 2006). In 1999, celecoxib was the first coxib to be approved for the relief of pain and inflammation in patients with osteoarthritis, rheumatoid arthritis and primary dysmenorrhoea (Patrono et al 2001). It was quickly followed by rofecoxib, etoricoxib, valdecoxib, parecoxib and lumiracoxib (Capone et al 2005; Cheer & Goa 2001; Fenton et al 2004; Wittenberg et al 2006). However, after chronic administration for longer than 12 months, the selective COX-2 inhibitors rofecoxib and valdecoxib were shown to significantly increase the risk of untoward cardiovascular events and so these agents were withdrawn from the market (Munir et al 2007).
OPIOID ANALGESICS
Opioid analgesics may be classified on the basis of their (i) WHO Analgesic Ladder classification as ‘weak’ or ‘strong’, (ii) chemical structure or (iii) pharmacodynamic profiles as agonists, partial agonists or antagonists at opioid receptors (Krenzischek et al 2008; Trescot et al 2008). The various opioid receptor types/subtypes, the effects transduced and their respective endogenous ligands are summarized in Table 11.3.
Table 11.3
Opioid receptor types, effects transduced and endogenous ligands
1Names per current NC-IUPHAR recommended nomenclature.
2Adapted from Maher and Chaiyakul (2003).
Strong opioid analgesics are recommend by the WHO as the drugs of choice for the management of moderate to severe pain whereas weak opioid analgesics are used in patients with mild to moderate pain, particularly when there are contradictions for NSAID usage (Krenzischek et al 2008). A comparison of equi-analgesic doses of clinically ultilized opioid analgesics is shown in Table 11.4 and common starting doses for a range of orally administered opioids are listed in Table 11.5.
Table 11.4
Total daily oral morphine equivalent conversion table (Nissen et al 2001)
Opioid analgesic | Conversion factor |
Pethidine (oral) Pethidine (IV) Methadone Oxycodone Buprenorphine Codeine Dextropropoxyphene Morphine (IV) Morphine (oral) | × 0.125 × 0.4 × 1.5 × 1.5 × 50 × 0.16 × 0.1 × 3 × 1 |
Apart from pain relief, opioid analgesics produce a range of adverse effects, including nausea, vomiting, sedation, pruritis, respiratory depression, constipation, thermoregulatory effects, immunomodulation, tolerance and physical dependence (Zollner & Stein 2007). Although it is widely believed that the analgesic and other effects produced by opioid analgesics are evoked by activation of the μ-opioid receptor, it is widely appreciated by frontline clinicians that there are intra-individual between-opioid differences in terms of both analgesic and tolerability profiles (Smith 2008). However, the precise mechanistic basis underpinning these observations is poorly understood at present.
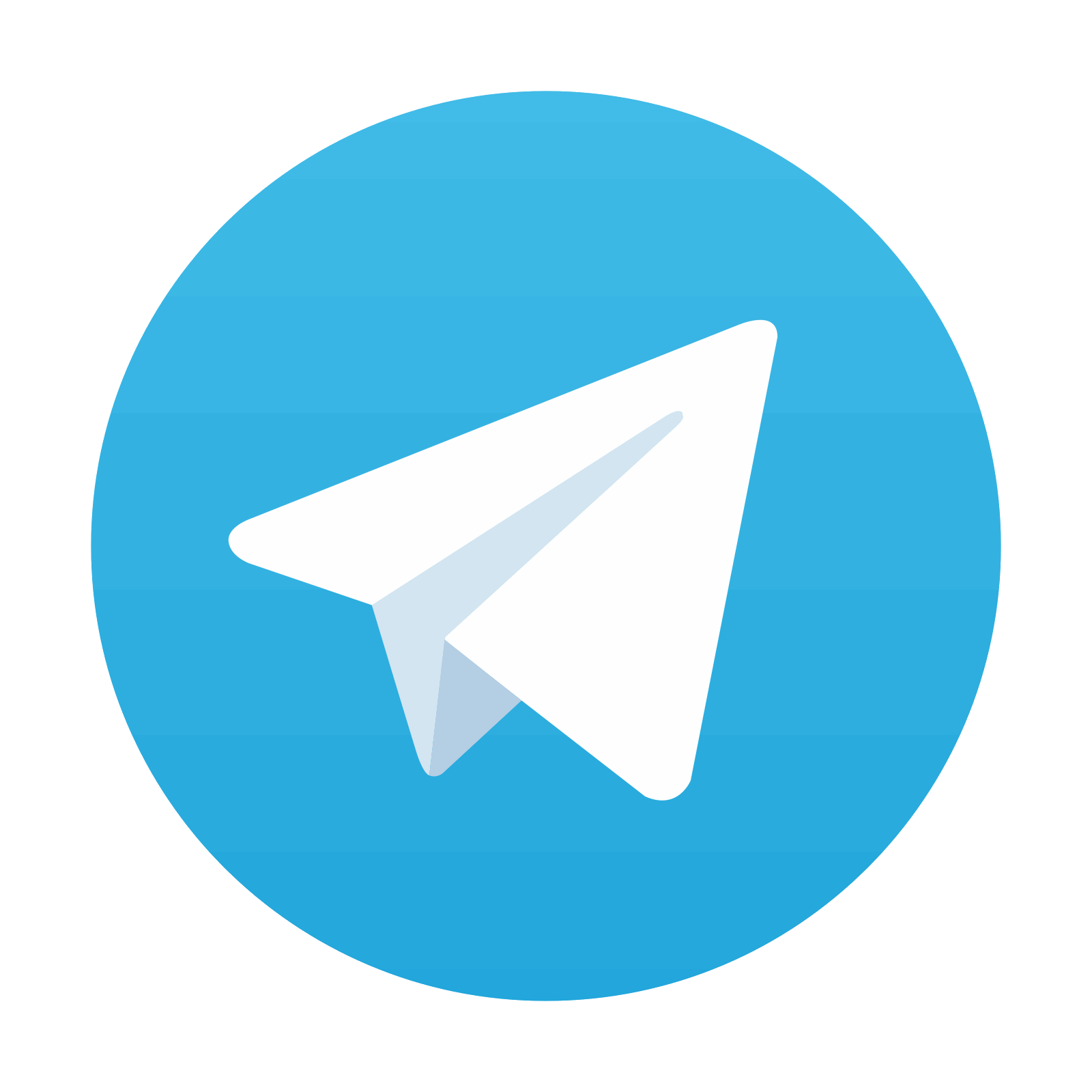
Stay updated, free articles. Join our Telegram channel

Full access? Get Clinical Tree
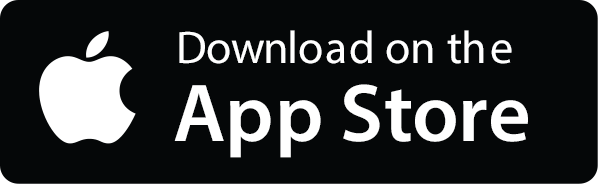
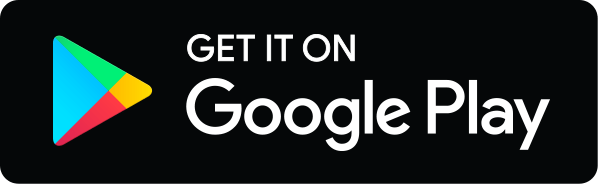