Downed tree branches and power lines on residential street in Toronto.
At lower elevations from October to April, populations of American, Asian, and European countries in extra-tropical northern latitudes are at risk from the effects of winter weather. However, regional probabilities for specific types of storms differ. In the United States, winter storms are more frequent in northern states, mountainous regions, and east of the Great Lakes (Figure 40.2). They uncommonly occur in the southern United States, but ice storms are especially treacherous when they do.6

Winter storm hazards in the United States. This graphic is in the public domain. It was reproduced from the National Weather Service’s pamphlet entitled Winter Storms: The Deceptive Killers.
As with all PICEs, the impact winter storms have on society is what defines their magnitude. Regions having little experience with winter storms often have the least prepared populations and, therefore, are at even greater risk. These areas may also have local and state governments with inadequate capacity to respond rapidly and effectively. Unprepared communities could exponentially increase the human and economic impact of an event. For instance, 1 m (3 ft.) of snow blanketing rural areas of America’s Great Plains may minimally disrupt populations and their associated agricultural and ranching industries. In distinction, 1 cm (0.4 in.) of ice from New York City to Washington, DC, could paralyze major commercial, financial, and governmental centers.
Understanding the human impact of catastrophic events, so society can be better prepared for future challenges, is a primary mission for practitioners of emergency management and disaster medicine. With regard to winter storms, the medical literature mostly analyzes events over the last three decades. Health effects of storms that move up the Ohio River Valley from the Texas Gulf Coast and the so-called nor’easters have been the most extensively reported data in the English-language medical literature, likely because of their relative frequency and effects on large population and economic centers in the north-central United States and northeastern seaboard.
A nor’easter that resulted in a major ice storm affecting an area of North America centered over the St. Lawrence River and extending east toward Nova Scotia, January 4–10, 1998, is one of the best described events. The storm resulted in over 4 million people being without electricity – some for up to 33 days – mostly in southern portions of Ontario, Quebec, and Nova Scotia and northern portions of New England.7 Those who were displaced from their homes in Ontario, and could not move in with a relative or friend, constituted almost 5% of the affected population, placing approximately 140,000 persons in 454 emergency shelters.8 Some Canadian hospitals were without power for 3 weeks.9 Estimated total damages for the two countries amounted to as much as 6 billion USD.7
Determining a cause of death to be from extreme temperatures is a diagnosis of exclusion, and difficult without awareness of risk factors and circumstances. Databases likely underestimate the numbers as some types of reporting are not required, no precise case definitions exist, and there is little quality control over death certificates. Nonetheless, U.S. data suggest that mortality from excessive cold is less common than from excessive heat.10 However, this might not be true on a regional basis, or in other countries with different climates.
Deaths from all causes in the United States are more common in January than for any other month. This is especially true for the elderly, and this higher mortality has been specifically linked to colder periods.11 A study of four consecutive winters in Minnesota showed a slight increase in mortality during cold days, but a greater increase in cardiovascular mortality during periods following snowfall.12 One report covering 6 consecutive January months in Pennsylvania found that there was a 1.27 times higher relative risk of dying during “extreme climatic conditions” – defined as when the temperature was less than –7°C (19°F) or more than 3 cm (1.2 in.) of snow had fallen.13 A British study noted a statistically higher risk of dying in winter months from 1986–1996 (1.5% higher for every 1.5°C [2.7°F] decrease in temperature), especially if no central heating was used in the household.14 The results of both studies demonstrated tight 95% confidence intervals.
The exact risk of storm-related mortality from all causes is not well known. There is no universal requirement to report that any individual’s death is or is not directly related to a weather phenomenon. Because of this, any generalized data must be viewed skeptically, unless the data set from which it is derived is specified. Therefore, planners cannot rely on these statistics to predict where resources will be needed to mitigate mortality rates before, during, or after a storm.
A massive blizzard in New England on February 6, 1978, was followed by two public health reports regarding mortality. Twenty-seven storm-related fatalities were identified in Massachusetts, but no overall increase in total mortality was appreciated.15 In Rhode Island, on the other hand, researchers concluded that there was an increase in total mortality in the first 5 days following the storm,16 although statistical methods were not used to compare the study group to an unexposed cohort.
Assuming any given healthcare system can maintain its capabilities during and immediately after a winter storm – or rapidly implement its existing plans or ad hoc processes to increase surge capacity – the emergency department (ED) is typically the first functional area affected, because it is the location where ambulances bring patients from the community and where the public is accustomed to seeking unscheduled care.
A Massachusetts study of fifteen hospitals found a significant decrease in ED visits on the day of the blizzard in February 1978, but daily census rapidly returned to baseline.15 A survey of five major hospitals in northeastern New York State noted a similar trend after a January 1996 blizzard, but this was then followed by a marked increase in ED volume the next day.17 Only one scientific article could be found that specifically reported the effects of a winter storm on a pediatric ED. The author found that the total daily census increased 35% in the 36 hours before a blizzard hit eastern Pennsylvania and Delaware in January 1996, decreased to very low levels during the storm, then slowly returned to baseline over the next 4 days.18 A post-event spike frequently reported in adult and combined adult/pediatric EDs was not seen, although there was an increase in the percentages of higher-acuity conditions and a near-tripling of the admission rate.18
Following a particularly heavy snow storm in a region of the United States where snow is expected, common storm-related mechanisms of injury (in descending order of frequency) included: slips and falls, MVCs, being struck by falling objects, carbon monoxide (CO) poisoning, and injuries and illnesses related to using clean-up equipment like chainsaws and snowblowers. Morbidity and mortality were also due to deficiencies in access to customary care, lack of home heating due to power loss, and inability to discharge patients to their existing home situations.17
Similar findings were reported from a single university medical center in the aftermath of an ice storm that affected much of North Carolina in December 2002, and interrupted power to 1.3 million homes.19 Ice more commonly downs power lines than does heavy snow. The most common mechanism of injury was from falling objects striking people who were conducting assessments and clean-up outside. An epidemic of CO poisoning was also seen in patients presenting to the same institution. These mechanisms accounted for all the life-threatening injuries, except one elderly patient who likely became hypothermic after a stroke. Slips and falls, injuries associated with darkness, and burns encompassed the other causes of injury. In this study, MVCs could not reliably be determined to be storm-related in many instances, so they were not specifically examined.19
One of the first epidemiological reports documenting an increased incidence of fractures associated with a winter storm was published by Ráliš in the aftermath of 5 days of snow and ice over 1 week around the New Year 1978–1979.20 In a UK ED with a census of over 93,000 patients per year, the number of patients with fractures increased 2.85 times over baseline, peaking at a rate of one in every five patients. In descending order of frequency, the locations of fractures were the wrist and forearm, foot and ankle, hand, hip, leg, chest, spine, and skull.20
In the midwestern United States, ice storms have led to numerous orthopedic injuries. Falls on ice, particularly in the elderly, more commonly resulted in extremity fractures than falls during equivalent periods of snow-cover in St. Louis.21 In the 9 days after a winter storm passed through Indianapolis in February 1994, 327 injuries in 259 individuals who slipped on ice were managed at a single hospital. Most were back injuries of various types, but over a third of patients were diagnosed with fractures of the non-axial skeleton.22 Following the 13-day ice storm of January 1998, Canadian EDs reported over one-third of the injuries seen were directly storm-related.23 This was also true in both adult and pediatric populations in Montreal.9 Physicians at Montreal General Hospital alone performed sixty emergency orthopedic operations for storm-related injuries.9
One statewide epidemiological study of 6,047 winter storm–related injuries in Oklahoma revealed that, for every eight injuries, six were due to falls and one was due to an MVC. Additional mechanisms included sledding, CO poisoning, clean-up activities, and “other” categories. Falls were more common in persons ≥40 years, whereas MVCs were more likely in persons <40 years of age. Falls were twice as likely to cause fractures in the older age group.24
CO poisoning is generally seen in winter months, and its incidence can be increased by a storm that results in widespread power losses. Sources of CO are usually burning fuel indoors for heat, electricity generation, or cooking with nontraditional fuel sources. Exhaust fumes filling automobiles with snow-obstructed tailpipes is another mechanism of CO poisoning following winter storms.
The rate of CO poisoning cases increased after a winter storm disrupted power to a large portion of the Seattle–Tacoma area of the northwestern United States in 1993.25,26 Thirty incidents resulted in eighty-one cases at thirteen hospitals through the 3-day storm. Another spike in CO poisoning cases was reported in the same area after two winter storms in late 1996.26 Following the North American ice storm of January 1998, over 1,000 cases of CO poisoning from at least 700 individual incidents were reported in Quebec alone, and this was likely fewer than actually occurred.9 Four hospitals in rural Maine reported 42 incidents causing 100 cases with up to 8 patients arriving from a single scene.27 About half that number was reported for two EDs in just one city in Ontario.28 After a December 2002 ice storm in North Carolina, a single university hospital saw 200 cases of CO poisoning in 1 week,29 while another one in a different city saw 48 after the same storm.19
Several studies report an increased incidence of fatal and nonfatal acute coronary syndromes (ACS) associated with winter storms.12,30,31 Specific risk factors could not be identified in a small cohort of patients surviving myocardial infarctions after a January 1979 blizzard in Chicago.32 Short-term cold exposure was not believed to be the cause of increased ACS when a Canadian study examined 15 years of epidemiological data.33 On the other hand, a Dutch study concluded that wind chill may have a greater effect on cardiovascular risk than cold air itself.34
Heavy snow shoveling has been shown to elicit sustained heart rates near age-calculated maximums with aerobic oxygen demands similar to arm-crank ergometry.35 Although a Canadian study concluded that the incidence of “heart attacks” was independent of snowfall, the ones that did occur were more likely to follow shoveling.36 In distinction, an American study of ten EDs in one New York county after a January 1996 blizzard affected much of the northeastern United States, found a 6.6 times increased relative risk of ACS events – primarily following snow shoveling, and often in persons without histories of coronary artery disease.37 Comparing consecutive January months from 1991 to 1996, a Pennsylvania study noted increased risk of cardiac mortality in males – progressively higher in younger age groups: 1.28–2.21 for those > 65 years old; 1.32–2.38 for those 50–64 years old; and 2.35–5.35 for those 35–49 years old.13 In another study, the rate of non-cardiovascular illnesses was not noted to change.37
Scope of Other Winter Hazards
Accidental hypothermia can occur at virtually any ambient temperature, but it is more strongly associated with cold air and cold-water submersion. Death rates in the United States are 0.08–1.99 per 100,000 population in the contiguous 48 states – although, as expected, they are higher in Alaska.38 Therefore, accidental hypothermia is not a common cause of death, but is a potentially preventable one. Disabled persons, the elderly, and the socially disadvantaged are at particular risk at all times. However, during and after a winter event, these and other groups may have more acute needs due to disruption of power or inability to access assisting services.
Cold-water immersion is a complex phenomenon, which may or may not have coexistent hypothermia. Although epidemiological statistics are readily available regarding drownings in several countries, few are particular to cold-water immersions. One that did specifically address this examined the winter months during the 1991–2000 decade in Canada. Researchers determined that, out of 2007 cold-water immersion deaths, 1,245 (62%) occurred between November and April. Although the human activities just before these incidents were not directly linked to winter months or storms, the relative percentages may be useful for mitigation purposes: 45% occurred during boating or other aquatic activities; 22% while on ice (the majority of these on snowmobiles); 15% during land transportation collisions; and 14% from other falls into water.39 Epidemiological data regarding nonfatal cold-water immersion injuries were not reported.
Snow avalanche is a natural phenomenon involving snow accumulated over time, but winter storms increase the risks for populations living in avalanche-prone terrain. The financial impact and public health implications of avalanches are often relatively small compared to those from widespread devastating storms. Generally, the consequences of snow avalanche predictably involve human-built structures, transportation systems, and power and telecommunications infrastructure; but people can be injured when caught in their destructive paths.
In North America and Europe, there are about 150 deaths each year attributed to snow avalanches. There were 329 deaths from avalanches in Canada between 1978 and 2007. Outdoor recreation is associated with about nine in ten fatalities. North American avalanche victims most often include snowmobile riders and HeliCat skiers. Snowmobilers are the fastest growing percentage of avalanche victims.
In an autopsy study in Utah, twenty-two avalanche deaths from asphyxiation also had mild to moderate traumatic brain injury, and all six of the cases where trauma was the cause of death had severe brain injury.40
In a Canadian autopsy study of 204 avalanche fatalities, the immediate cause of death in 75% of cases was asphyxia. Trauma was the cause of death in 24% and hypothermia in 1% of cases. Of the trauma victims with single-system injuries, 46% were chest-related, followed closely by head injury in 42%, then 8% with neck injury. About half of the trauma casualties were completely buried in snow. Victims of asphyxia were buried in 92% of cases. Trauma victims were buried an average of 90 cm (about 3 ft.), and asphyxia cases were buried an average of 150 cm (about 5 ft.). Burial time averaged 25 minutes for trauma cases and 45 minutes for asphyxia cases.41
A study of Austrian avalanche victims from 1994–2005 included 1,745 people. The authors reported 565 cases either partially or completely buried. Records were reviewed on ninety-four victims admitted to Innsbruck Medical Center. There were sixty-seven (71%) survivors to discharge. Cardiopulmonary resuscitation (CPR) was performed on arrival for twenty-three victims, with a mortality rate of 91%. The most frequent diagnosis in this study was hypothermia (56%). Spinal fractures were found in 7%. A protocol that used total-body computed tomography imaging missed only 2.1% of injuries detected later.42
The most common nonfatal injuries in avalanche victims are orthopedic, craniofacial, and soft-tissue injuries. In a radiological study of fourteen avalanche victims in Switzerland, 133 imaging studies demonstrated 61% musculoskeletal injuries and 39% extra-skeletal injuries. Fractures of the axial skeleton (26.8%) were more common than the extremities (9.8%). Non-skeletal findings were intrathoracic (39%), intra-abdominal (15%), and intracranial (10%).43 Chapter 42 contains additional information on avalanches.
Current State of the Art
Decision-makers must know a situation exists before they can implement preparedness plans and devote resources for a targeted and coordinated response. It is usually easy to detect that a winter storm has dropped precipitation on a given region, but its human impact is much more difficult to assess, especially when aerial over-flights and on-the-ground access to affected areas are limited. Establishing an incident command structure, regardless of size, collects the resources officials need to determine the security and safety of affected areas, identify hazards to responders, and coordinate the support necessary to begin rescue and recovery efforts.
Winter Hazards
Storms and their aftermaths affect both populations and societal systems, the latter of which includes the ability to respond to community emergencies and to deliver healthcare outside and inside hospitals. Public health surveillance and interventions, emergency medical services (EMS) systems, and regional hospital capacity can all be adversely affected by power losses, hazardous driving conditions, and geographic isolation. Snow avalanches can cause similar problems, although generally on more localized scales than widespread storm damage. Cold environments can be treacherous to both the general population and public safety personnel.
Weather Conditions
One of the purposes of government is to protect the public welfare, particularly with resources not available to individuals or private groups. Most developed nations have one or more methods of notifying their populaces of important weather conditions that may adversely impact people or property. In the United States, this responsibility begins with the National Weather Service (NWS).
NWS may issue a “winter storm watch” when there is an increased risk of a hazardous weather or hydrologic event, but its occurrence, location, and/or timing is uncertain. The purpose of a “watch” is intended to alert the population at risk to initiate protective actions. Once its weather predictions are more certain, a “winter storm advisory” or “winter storm warning” may be issued – or the message may be more specific, such as “blizzard warning.”
The term “advisory” highlights special weather conditions that are less serious than a warning. They are for events that may cause significant inconvenience, and if caution is not exercised, could lead to situations that may threaten life or property.
The term “warning” is issued when a hazardous weather or hydrologic event is occurring, is imminent, or has a very high probability of occurring. A warning is used for conditions posing a threat to life or property.
Although print media is useful for long-range forecasts (i.e., more than 24 hours in the future), broadcast media and the Internet are the most common methods used for more immediate notifications of approaching winter storms. The U.S. National Oceanic and Atmospheric Administration (NOAA) All-Hazards Weather Radio broadcasts important information directly from the nearest NWS office around the clock.
Storm severity categories may help populations at risk, emergency response organizations, and other public and private facilities (e.g., government centers, hospitals) prepare for potential effects. Hurricanes, known as cyclones in some parts of the world, and tornadoes each have a well-known severity index associated with them: 1–5 for the former and 0–5 for the latter. A seven-level categorization scheme, called the Local Winter Storm Scale (LWSS), has also been proposed.44 It is based on five weighted meteorological factors over any given area: sustained wind speed; speed of wind gusts; snow accumulation; ice accumulation; and visibility. The overall LWSS category may help predict a storm’s societal impact. Although it has been correlated to an older Realized Disruption Index, the LWSS has only been retrospectively derived to date, and is not yet in as widespread use as other storm categorization schemes. However, one advantage is that impact predictions can be modified moment-to-moment as constantly updated weather data are received and analyzed.
Responding to emergency scenes is hazardous in any environment. Dangerous winter road conditions and limited visibility caused by winter storms, as well as the increased risk of becoming stranded far from shelter amplify the risk. EMS, fire/rescue, and law enforcement personnel usually receive formal training on emergency driving. However, specific driving instruction for winter weather is often only didactic in nature. Few response organizations provide hands-on training that allows drivers to experience real winter emergency conditions in controlled situations using operational response vehicles, which handle much differently than passenger vehicles. Similarly, few private companies or public service organizations – including those delivering healthcare – provide any driver training to those who must respond during or after winter storms. When road conditions exceed driver abilities to navigate them, the number of emergency scenes may increase, and thereby compound the overall demand for rescues and out-of-hospital medical responses.
When driving during or after a winter storm, speed must be reduced to improve traction and decrease distance required to identify hazards and stop safely. Heavy snowfall and wind-swept snow can cause near “white out” conditions with visibility less than 15 meters (50 ft.). Slick conditions on road surfaces increases stopping distances. Negotiating hills and curves may be difficult. Even stepping out of vehicles can be more risky, and slips and falls can injure responders. Parking or exiting a vehicle on a roadway can be very dangerous when other vehicles are moving around it. Distracted by flashing emergency lights, other drivers may lose control of their own vehicles causing impacts with emergency response vehicles and their crews.
“Black ice” is a term referring to a thin layer of ice that cannot be seen when it is adhered to darkly colored road surfaces, yet is just as treacherous as an icy surface several centimeters thick. This invisible ice layer can develop in minutes when a wet roadway at the freezing point suddenly becomes colder due to evaporation via wind or with the loss of the sun’s heat (e.g., with clouds, sunset, or shade).
Avalanche Terrain
Avalanches are characterized by three physical zones: a starting zone, where unstable snow layers begin to move (Figure 40.3); a track, where snow flows down a slope below the starting zone; and the runout zone, where snow and debris collect at the bottom. Avalanches containing dry snow usually begin on slope angles greater than 25°, but avalanches on wet snow can begin on shallower slopes. Generally, wind-deposited snow is at greater risk for breaking loose. Places where snow is deposited by wind are near ridge lines, behind trees, and behind high or convex terrain.

Separation of snow from a ridge at the starting zone of an avalanche.
Orientation to the sun affects snow stability, and changes with temperature and season. Sunny slopes are more stable in winter, but rapidly become unstable as spring temperatures rise. Shady slopes allow snow to remain cold in winter, with a tendency to form unstable layers from frost. Shady slopes stabilize slowly as spring arrives.
Terrain features also influence the likelihood that snow will begin to slide. Forest cover generally stabilizes the snow. Timber harvesting from steep mountains in Europe was stopped almost 100 years ago to preserve protected forests. Surface obstacles such as tree stumps, boulders, shrubs, and rock formations can anchor snow until it is deep enough to form unstable layers above these features. In rough terrain, more than 1 meter (3 ft.) of snow is required to produce avalanche-favorable conditions.
Avalanche speed and impact pressure determine the destructive power of the slide. Avalanche material can accelerate rapidly near the starting zone and reach over 25 m/s (82 fps) within the first 200 meters (218 yd.). Dry-snow avalanches begin as a slab or as loose snow with a relatively dense core at the bottom of the slide covered by a snow cloud when speeds exceed 10 m/s (33 fps). The overhead cloud can be over 10 meters (33 ft.) high, which can seriously obscure visibility if trying to watch for victims during an observed avalanche (Figure 40.4). The depth of the core is typically 1–2 meters (3–6 ft.), but can be deeper. As the snow slides, friction causes water to form on the surface of snow particles such that, when the moving snow mass comes to rest in the runout zone, it hardens immediately as the water freezes. The hardened snow makes self-extrication nearly impossible, even when persons are only partially buried. Deposit densities from avalanches range from 200 kg/m3 (12.5 lbs./ft.3) for small dry-flow types to over 1,000 kg/m3 (62.4 lbs./ft.3) for large slush-flow types.

An avalanche in the Caucasus Mountains, Bezengi, Kabardino-Balkaria.
Powder-only avalanches lack a dense core, so they tend to cause less physical damage. Dry-flow avalanches are probably the most destructive overall due to their velocities. A dry-flow avalanche at Rogers Pass, British Columbia, generated impact pressures over 600 kPa (87 psi).45 An impact pressure of 100 kPa (14.5 psi) will uproot a mature tree, and 1,000 kPa (145 psi) will move a reinforced concrete structure. Air blast is a phenomenon where air in front of high-speed avalanche material is compacted into a high-pressure wave. This can cause additional damage in an area wider than the flow itself, but most avalanches are not associated with a significant air blast.
Cold Environments
Other winter hazards are generally less likely to result in disaster situations by themselves, but cold environments can affect indoor living with inadequate heat and complicate many outdoor activities. Recreation can be curtailed if necessary, but emergency responders may have fewer options. They must be prepared for hazardous driving or flying conditions to and from scenes; the effects of cold, wind, and wet conditions on themselves, their patients, and their vehicles; and on potential disruptions to radio, cellular, and satellite service created primarily by atmospheric conditions or secondarily by damage to power and telecommunications systems.
Responders must also ensure that their equipment and supplies are functional in cold environments. Vehicles must be well maintained and prepared for operations in cold weather or slick surface conditions. This is true for all ground-based vehicles, which are subject to maintenance standards at local and national levels in most countries if governmentally owned, but may not be if owned by individuals or businesses (e.g., utility companies). The same applies to aircraft used as ambulances or for other public service applications, the maintenance and operations of which are even more stringently controlled, especially as regards adverse environmental conditions.
Winter temperatures are harsh on vehicles. Engine and transmission oils become more viscous at lower temperatures, requiring longer warm-up times for vehicles kept in unheated locations for any period of time. Water in fuel lines may freeze. Block heaters are used to keep engine fluids warmer to facilitate starting. Windshield wipers become less efficient as rain freezes or turns to sleet or heavy wet snow, hampering visibility. Headlamps can become covered with wet snow, reducing the light output reaching roadways to almost nothing. Snow may also obscure directional signals, parking lights, and emergency flashers, making it difficult for other motorists to see vehicles at sufficient distances to avoid collision on slick roadways.
Crashes and vehicle incapacitation can result in response personnel being stranded while driving in inclement weather, ice, or heavy snow. Radio communications may be degraded and cellular or satellite telephones may be nonfunctional during or immediately after a winter storm. Response personnel must be prepared for the inability to communicate with their own dispatch authority, other emergency response agencies, or an Emergency Operations Center (EOC). Medical control for patient care advice or authorizations beyond an EMS crew’s standing orders or scope of practice might be limited. Communications problems may also make it difficult to call for assistance, if stranded.
All emergency response personnel venturing into the winter environment must have operational guidelines for a communications outage or cold-weather vehicle failure. Most authorities recommend that stranded motorists stay with their vehicles, but caution them to guard against cold injury if not heating the interior, and CO poisoning if running the engine or using some other heat source.
Many emergency response vehicles, which operate in areas likely to receive ice and snow, are equipped with chains that can be used either on their tires or in a deployable apparatus under the chassis such that, at the touch of a button, chains are rotated on a horizontal axis near the tires resulting in enhanced traction equivalent to chained tires.
Response personnel must be properly attired, equipped, and trained for work in cold, windy, and wet scenarios. Typical clothing worn by EMS responders on a day-to-day basis may not adequately protect them from the elements, because winter storms may prolong scene times in harsh outdoor environments.
Clothing worn should minimize the risk of injury yet maximize dexterity and function. The overarching goals are to preserve core body heat and to minimize exposure of skin to cold air and surfaces. Knowledge about environmental conditions is essential so that proactive and reactive behaviors will be appropriate to prevent tissue damage. Mental status changes from environmental exposures can lead to deleterious behaviors such as paradoxical undressing. The following are general recommendations for the prevention of cold-induced problems:
Adequate hydration, nutrition, and rest are essential. Being in excellent physical condition is an advantage. Use of tobacco products is detrimental to good circulation. Clean, healthy skin is better at preventing cold injury, but washing too frequently can dry and damage protective barriers.
Thermal insulation can be provided by garments themselves and trapped air between fabric layers. The mnemonic for prevention of COLD stands for Clean fabrics; Opening for ventilation during exercise (to avoid wetting perspiration); Loose layers to retain insulating air pockets, and allow for donning and doffing layers as conditions change; and Dry garments, which must be changed if they get wet. The head must be well insulated, because significant body heat can escape from the exposed scalp.
Avoid tight-fitting clothing and restrictive gloves or boots. Liners improve the insulation of both. When dexterity is not needed, mittens are more effective at retaining heat than are gloves.
A balance must exist between external water impermeability and the risk of retaining sweat inside protective garments that cannot breathe. Particularly with regard to the feet, wet conditions soften the skin, which can lead to debilitating nonfreezing injuries like trench foot. Extra socks must be available so that damp socks can be exchanged for dry ones.
Protect exposed skin surfaces from cold and windy air, as well as from cold liquids and surfaces. Avoid damaging skin through ultraviolet radiation directly from the sun or indirectly from reflection off ice, snow, water, windows, or light-colored building surfaces.
Use the buddy system, especially by trained individuals who consciously and frequently check each other, to decrease the likelihoods of insidious frostbite and hypothermia.
In addition to rescuers, these recommendations apply to all persons living indoors with inadequate heating or outdoors exposed to cold environments.
Local Medical Responders
Winter storms may severely limit the ability of responders to reach callers in a timely manner, or to find victims during a community needs assessment or deliberate search. Once located, care may have to be delivered in relatively austere medical conditions for periods of time that may far exceed those to which first responders and EMS personnel are accustomed. Transportation to a permanent or temporary medical facility, or even to a heated shelter with power and food, may be so treacherous that the risk–benefit ratio is higher than staying in place. Response organizations and systems must consider how to best plan, train, operate, and recover in these environments.
Access
Winter storms may severely limit the ability of responders to access known victims or areas to search for unknown victims. Much has been written in books and journals and posted on the Internet regarding tactics, techniques, and procedures for searching for victims who cannot call for help after a storm with freezing precipitation or after being buried in an avalanche runout zone. However, virtually nothing has been published on best practices for EMS and other public safety personnel who must access persons who have called for help during or after one of these events.
Response efforts need to be coordinated with other community resources when debris or fire, downed power lines, ruptured natural gas or water pipelines, or physical violence threaten the safety of responders. Response should be coordinated through a local or regional EOC functioning under an incident command structure. In advance of a winter storm, a local EOC could prohibit ambulances from traveling off plowed roadways, yet still be responsive to requests for assistance. For instance, response assets could be reorganized into task forces, such that a call for medical help would result in dispatch of a snow plow, a four-wheel-drive vehicle with a command officer and a medic, and a staffed fire engine. These resource groupings could be kept intact between calls. Public health representation could also facilitate data collection for a rapid needs assessment.
Access to avalanche victims is challenging. Survival of those not initially killed from injuries is associated with four parameters: 1) burial depth; 2) the victim’s ability to self-extricate; 3) body parts or objects attached to a victim exposed above the snow; and 4) terrain effects on victim access, care, extrication, and evacuation by rescuers.
According to the Colorado Avalanche Information Center, since 1950 about 63% of avalanche survivors were rescued by companions compared to 19% by organized rescue teams. Use of beacons can improve survival. However, in an Austrian study of 109 totally buried victims, only 31.1% of victims had transceivers and none were rescued by companions.46 It is rare to survive for more than 1 hour when buried. When people are caught in an avalanche, observers should establish a “last-seen point.” Rescuers must consider the risk of additional snow slides before entering an area to begin any search. Companions should organize a hasty search beginning from the last-seen point. The hasty search is performed by first looking for visible clues such as equipment or clothing, and listening for voices. If there is no surface clue to locate the victim, a beacon search should be undertaken. If beacons were not attached to individuals or a search for beacon signals fails to locate any victims, a probe search should be initiated. Communication with search-and-rescue teams should occur as soon as possible, but should not delay the hasty search.47
Out-of-Hospital Care
Hasty rescue and rapid out-of-hospital care of avalanche victims may be lifesaving. Asphyxiation is the leading cause of death after avalanche burial, mostly from decreasing oxygen and increasing carbon-dioxide fractions within any sealed air pocket. When victims are freed within 15 minutes, the survival rate is over 90%. However, when victims remain buried for 30 minutes, the survival drops to 30%. Survival beyond 30 minutes depends on the size of the air pocket around the victim’s face.48
Because rescuers may need to remove up to 1.33 metric tons (approximately 3,000 lbs.) of snow to reach a victim buried under just 1 meter (3 ft.) of snow; the priority of rescue efforts should be focused on the victim’s head and face. Once cleared of ice and snow, airway management and assisted ventilation, with or without endotracheal intubation, should be completed as soon as access is achievable, before further efforts to free the rest of the body.
With the exception of conditions directly related to heat loss, individual out-of-hospital patient care should be similar to that without winter conditions. Nevertheless, responders must be aware of the risks of cold weather to them and their patients, and must anticipate delays in access and evacuation during storms. Illnesses may be more advanced or complications of injuries may be more manifest when access is delayed. Additionally, the duration of care may be extended for longer than EMS and rescue personnel are otherwise accustomed. Depending on limitations in evacuation options or extensions of transportation times, care may have to be delivered for hours, or perhaps even days in extreme circumstances.
Evacuation delays may necessitate hemorrhage control methods less familiar to civilian EMS responders. Exsanguinating extremity hemorrhage may have to be controlled with a proximal tourniquet, either by inflating a blood-pressure cuff or applying a prefabricated or field-expedient device. Application of dressings or powders containing clot-enhancing agents such as kaolin, mineral zeolite, microporous polysaccharide microsphere, poly-N-acetylglucosamine (chitosan), or fibrin may be useful adjuncts.
Since people routinely postpone seeking medical care when there is no extreme weather, a delayed response under storm conditions may not pose additional challenges for responders. A notable exception is when patients’ health conditions require services that are disrupted by the storm, for example, a patient on a home ventilator with failure of back-up power. Injured patients, on the other hand, may be more likely to seek care immediately, yet medically trained providers may not be able to access them rapidly. Delayed complications (e.g., established wound infections, gangrene or tetanus, compartment syndromes) or progressive conditions (e.g., increased intracranial pressure, pulmonary contusions, slow intracavitary hemorrhage) may be less familiar to EMS personnel – and their scope of practice may only allow for supportive care, even when delays in evacuation to definitive care could result in loss of life or limb.
Frostbite and hypothermia are the most common cold-induced conditions. Out-of-hospital management consists of removing the source of the cold insult and supporting the patient during evacuation to a higher level of care. Active rewarming is difficult in the field. In situations of widespread power loss, the only source of added heat may be the interior of an evacuation vehicle. If specialized equipment is available and functional within the response vehicle, this represents an additional rewarming option. In general, though, active rewarming is conducted at a medical facility.
Cold injuries can be divided into nonfreezing and freezing categories. The former includes pernio, trench foot, and immersion foot. Pernio results from the combined effects of wet and cold skin, but may occur in dry nonfreezing conditions. Trench foot may occur in chronically wet feet exposed to near-freezing temperatures and made relatively ischemic by vasospasm and increased tissue pressure induced by standing or tight footwear. Immersion foot results from skin that has become waterlogged after prolonged cold-water immersion. Injuries that actually freeze tissue include frostnip and frostbite.
Pernio, also called chilblains, is a localized inflammatory condition of the skin, which results from an abnormal tissue response that develops over 12–24 hours after cold exposure. There is frequently a history of Raynaud’s phenomenon. Pernio most commonly manifests as tender bluish or purplish subcutaneous nodules in exposed areas. These lesions are often associated with edema or blister formation. Treatment consists of local massage to stimulate blood flow and slow rewarming at normal room temperatures. Active rewarming with higher temperatures significantly increases the intense burning and itching associated with resolution.49 Nifedipine may have a role in hastening clearance of the lesions with less discomfort, and possibly reducing the likelihood of recurrence.50
Trench foot and immersion foot are clinically indistinguishable. They both progress insidiously through three phases: pre-hyperemic, hyperemic, and post-hyperemic. Intense vasospasm causes skin blanching and mottling in the pre-hyperemic phase. Peripheral pulses may be diminished. Capillary refill is usually prolonged. Continued exposure results in anesthesia and gait disturbances from damage to sensory and proprioceptive nerves.49 Rewarming creates a hyperemic condition with erythema, petechiae, swelling, pain, and hypesthesia – yet is still associated with prolonged capillary refill.51 Epidermal sloughing may occur. Nerves controlling voluntary muscular action and vibratory sensation may be adversely affected. Therefore, the goal of treatment is rewarming core body temperature without directly warming the affected body parts, so as to keep the metabolic demands of the injured tissue low.49 The post-hyperemic phase is not normally seen in the field, unless evacuation is significantly delayed after rewarming.
Frostnip, which heralds the beginning of tissue ice-crystal formation, is a warning sign that frostbite is imminent. Vasoconstriction leads to pallor, localized pain, and sensory numbness. Clinical manifestations can be readily reversed by preventing additional cooling and by rewarming the affected body parts.49
Frostbite represents freezing of extra- and intracellular water with cell injury or destruction leading to tissue damage, which can be compounded by microvascular stasis and ischemia. Typical symptoms progress from feeling local cold to loss of sensation. Pain may occur in a “watershed” area between frostnip and frostbite, typically more proximal to fully involved areas of distal tissue freezing. Frostbitten skin may appear a waxy yellowish white or translucent bluish color. It may be frozen solid.
Prevention of further cooling should be the primary goal prior to in-hospital management of frostbite. Wet, or possibly frozen, clothing should be gently removed. If adhered to the skin, other portions of any garments can be cut away, leaving frozen bits of clothing temporarily attached. Out-of-hospital rewarming of frozen tissue is generally discouraged, unless evacuation to definitive medical care will be significantly delayed.52 A 10-year Canadian study found that delay to medical care was one factor associated with poor frostbite outcome, so delayed access or prolonged evacuation times may have implications for prognosis.53 One author suggested 2 hours of field time as a cutoff to begin thawing in the field, but only if no chance of refreezing is possible.54
The position of the International Commission for Alpine Rescue provides a useful extrapolation from mountaineering to victims of other winter hazards.55 Guidelines are divided into whether the victim is out in the open or inside shelter, but commonalities include: removal of wet clothing; orally administered warm fluids; and aspirin up to 1 g or ibuprofen up to 800 mg, if available. In shelter, if active rewarming is performed by immersion into a warm 37°C (99°F) bath, the patient should not be allowed to subsequently use the part, which includes walking if the feet are involved, until after definitive care has been rendered. Because edema will ensue as the part is warmed, the area should be elevated and dry dressings loosely applied.55
Prevention of further cooling is also the primary goal for the out-of-hospital treatment of hypothermia. Other field management options are limited. Patients with mild hypothermia (i.e., core body temperature 32–35°C [90–95°F] and still capable of shivering) may warm themselves by being covered with dry, heat-retaining clothing, blankets, or other items. These interventions allow endogenous heat production through metabolism and the shivering reflex, and constitute the passive external rewarming technique. Active external rewarming involves the addition of exogenous heat to the body through a warm environment or radiant heaters. External rewarming interventions can be supplemented by warm oral fluids not containing caffeine or warm intravenous crystalloid fluids, but these field methods of active internal rewarming have only minor temperature-raising effects – although they may have an additional benefit of volume repletion, because many victims of hypothermia are also dehydrated.52,54,56
Bradycardia is often observed at core temperatures less than 28°C (82°F). In severe hypothermia, when the temperature is less than 25°C (77°F), more serious cardiac dysrhythmias occur. Development of new atrial fibrillation is ominous, as it may herald progression to ventricular fibrillation. When dysrhythmias complicate hypothermia, the treatment of choice is rewarming. Medications are not likely to be helpful until the heart is warm.57 Transthoracic pacing is possible, but not required in most cases.57,58
The out-of-hospital management of cold-water immersion is much the same as for any drowning, and is primarily supportive. Hypothermia may or may not occur during cold-water immersion, but the field care of hypothermia is the same as delineated previously.59 However, many successful resuscitations with good neurological outcomes following prolonged submersion in cold water have been reported. Therefore, assuming the resources are available and transportation times reasonable, responders should consider initiating interventions even when immersion times are known to be up to 1 hour or more.
Avalanche victims found in cardiac arrest and buried for less than 1 hour are not likely to have core temperatures below 30°C (86°F), and resuscitation will probably fail because of prolonged asphyxia without hypothermia. An air pocket must be present for a victim to survive long enough to develop hypothermia as a cause of cardiac arrest.60,61 If the victim is buried for more than 1 hour and uncovered with an intact air pocket, relatively protective hypothermia could exist, and CPR and transport for core rewarming should be considered.
Evacuation
Just as winter weather can affect the ability of first responders to access victims, it can also make it hazardous or difficult to transport them from out-of-hospital scenes to medical facilities. The usual evacuation assets may not be available. Standard ambulances may not be outfitted for driving to or from a victim’s initial location. Few have four-wheel drive, so nonmedical vehicles of expediency may have to be used. Moreover, helicopters may not be operational or safe to fly. In a Canadian study, 30% of requested helicopter EMS missions were aborted due to weather, which was the next most common reason behind the 42% combination of the helicopter not being the appropriate vehicle and cancellation by medical control.62
Determining the most appropriate destination for patients should be guided by local protocols, but the winter environment may force additional considerations in a disaster setting. Some medical facilities may be directly affected by the winter storm. Impaired ground and air accessibility, power losses, and staff absenteeism are just a few of the reasons a hospital or other facility may not have the capacity to receive new patients from the field. Other facilities may be overwhelmed by high patient volume and higher patient acuity in a storm’s aftermath. Routing of transportation assets directly to centers offering specialty services may be required for pediatrics, major trauma, serious burns, and hyperbaric oxygen therapy to name a few; but transportation to these services may not be expedient or safe during hazardous winter conditions.
Finally, the possibility of being stranded during evacuation could be complicated by having to maintain care for one or more patients for much longer durations than medical training, supply quantities, and vehicle power were designed. Telecommunications with medical control, to assist medics in these unfamiliar situations, may or may not be available.
Despite governmental regulations and industry guidelines on operations of emergency vehicles, there are few standards regarding cold-weather storage and use of onboard medical equipment and pharmaceuticals. Ground-based ambulances can often be parked in some kind of a garage while awaiting calls. This is rarely an option for helicopters, especially when they are hospital-based. Two North American63,64 studies and one European65 study have examined temperatures inside medication containers on rotary-wing ambulances. Both identified temperatures far outside the range recommended for pharmaceutical storage, although the clinical impact of this is uncertain. An important exception would be cold intravenous fluids, which could be potentially harmful to many patients, if given in any significant quantity.
Local Medical Receivers
A challenge for hospitals is inadequate staffing resources (e.g., inability to surge with off-duty staff due to weather preventing travel from home and on-duty staff overworked due to lack of relief) or capability degradation (e.g., interruption of facility water and power and supply expenditure without replenishment). Nonetheless, patients continue to seek care for a variety of baseline and storm-related problems. As in the field, the care delivered to individual patients is similar to that in other situations, except that cold-induced injury, hypothermia, CO poisoning, and other storm-associated conditions may complicate conventional management. Establishing disaster triage protocols to screen victims for these potentially occult problems is recommended to enhance ED operations following a winter storm.
Primary Triage
Because the ED is the focal point of victim reception, it is also the site of initial triage of persons presenting for care. Patient conditions may or may not be directly attributable to the storm, but all individuals must be screened. In addition to standard triage questions for potentially serious illnesses and injuries, high levels of suspicion for frostbite, hypothermia, and CO poisoning are required when people present to the hospital following a winter storm. Such storm-related conditions can be coexistent with a seemingly unrelated chief complaint. In order to limit the risk of missing these potentially occult conditions, triage personnel should specifically ask about exposure to cold, wet, or windy conditions, as well as the possibility of exposure to burning fuels.
If sufficient resources are available for the volume and acuity of conditions, little or no adjustment to routine operations may be necessary. However, any excessive patient need or limitation in healthcare capacity may require a different approach to triage and activation of facility disaster plans. Protocols should address the probability of various conditions, establish reasonable diagnostic parameters recognizing the potential limitations in laboratory and radiology services, provide management guidelines, and identify admission and discharge criteria that may be different than those under non-disaster circumstances.
Emergency Care
Traumatic injuries directly related to winter storms include those sustained during outdoor movement (e.g., slips and falls, MVCs) clean-up efforts (e.g., snow clearing, tree removal, utility repair) recreational activities (e.g., sledding, snowmobiling), and those due to generally hazardous conditions (e.g., avalanche, ice-covered bodies of water). As most of these mechanisms are familiar to emergency professionals, only those unique to winter weather are discussed in this chapter.
Although often purchased for recreation, snowmobiles can be important transportation sources during and after a winter storm. While able to facilitate transport for both patients and staff, snowmobiles are also associated with increased need for healthcare resources. Snowmobile crashes involve drivers, passengers, pedestrians, and people being pulled on sleds. Most injuries are musculoskeletal, but head, chest, and abdominal injuries occur frequently. Trauma around the knee is common, and fractures of the femur and tibia encompass nearly half of the injuries. In a study of snowmobile collisions in Canada, 74% of victims required emergency surgery with a mean of 1.6 operative procedures per patient.66
Complex wounds caused by a variety of mechanisms may present after winter storms (Figure 40.5). Many follow the use of power equipment for clearing debris, fallen trees, and snow. Chainsaw injuries occur throughout the year,67 but snowblower injuries can be expected following a heavy snowfall.68 Following a 1997 storm in Rhode Island, seven of eleven patients injured by snowblowers indicated that they placed their hand into a running machine, though three of the remainder said that the machine was off at the time of injury. Amputation was common, as were open fractures and tendon injuries. Ten hand injuries were managed in the ED by hand surgeons and one required inpatient treatment. The majority of cases involved the index, middle, and ring fingers.69

Typical snowblower injury. The patient placed his hand into the running auger to remove a chunk of ice with resulting open fractures of index and long fingers. The avascular, denervated index finger was amputated at the metocarpalphalyngeal joint.
Management of open wounds should follow traditional wound care guidelines. Wounds should be thoroughly described with emphasis on size, shape, depth, foreign material, local perfusion, distal circulation, tendon function, neuromuscular strength, and two-point discrimination prior to anesthesia. Plain radiography should be routine. Computed tomography or ultrasound may be used to further evaluate for deep foreign bodies. Bleeding and pain must be controlled prior to imaging studies.
Resuscitation may be required, and significant blood loss might necessitate transfusion. However, administration of blood products in the face of a winter-weather emergency should be carefully considered, because of the limited ability to replace banked blood. Both regional anesthesia and procedural sedation are useful adjuncts for ED evaluation and management of large complex wounds.
An important prerequisite for complete assessment is a bloodless field. Arterial bleeding can be controlled by ligating small vessels or using a blood-pressure cuff inflated proximally. This tourniquet technique can be applied for up to 2 hours while vascular control is achieved, the wound is explored, and copious irrigation accomplished.
All open injuries from power tools should be considered crushed and contaminated, and thus require thorough cleaning to remove gross debris and reduce bacterial load. Clean tap water is an acceptable alternate to saline for irrigation of wounds. Debridement of devitalized tissue is important.
Every patient with a wound is susceptible to tetanus, even though there are few reported cases in developed nations. Public health authorities recommend a tetanus toxoid booster when there is evidence of a complete initial vaccination series and the last booster was over 5 years prior to any tetanus-prone wound. In the absence of the primary immunizations, tetanus immune globulin plus tetanus toxoid is recommended.
The use of antibiotics by any route in the management of simple wounds offers no clear advantage or disadvantage in preventing infection. In the face of a winter-storm disaster, physicians should consider the potentially limited availability of community pharmacies, and ability of patients to return for any complications when recommending antibiotics or other medications. Use of antibiotics for uninfected simple wounds is a questionable practice, and is generally not supported in the medical literature. However, management guidelines for wounds that involve tendon, bone, nerves, or large vessels generally include antibiotics.
Since a winter storm might delay presentation of patients with complex wounds and availability of consultants may be limited, emergency physicians should consider delayed primary closure, especially for injuries more than 6 hours old. If not prevented from restrictions on travel or limitations in personal resources, the patient could return for daily dressing changes prior to definitive debridement and closure in 3–5 days. Non-operative ED management of fingertip amputations is accepted practice, although telephone consultation with a hand specialist is advised prior to repair.
In addition to traumatic injuries, the winter environment is further associated with cold injuries and hypothermia in exposed individuals representing a wide range of demographics.55,56 Power failures after winter storms lead to use of alternate power and heat sources that are associated with CO poisoning.27 These three entities can lead to death or permanent disability, but may be clinically occult and missed as a primary or complicating condition, if not actively sought.
Freezing (e.g., frostbite) and nonfreezing (e.g., trench foot and immersion foot) cold injuries should be anticipated following winter storms. The risk for cold injury is markedly higher in both African-American men and women than in whites of either sex. Additional risk factors include alcohol or drug use, psychiatric disease, motor vehicle trauma, and motor vehicle failure. Patients with prior cold injury appear to be more susceptible to recurrence.56 All of these cold injuries can be permanently debilitating.
Symptoms and signs of cold injury are based on the changes associated with tissue ischemia and the freezing process. Vasoconstriction reduces blood flow when skin temperature falls below 15°C (59°F). Other skin changes occur when extracellular ice crystals form at temperatures below 0°C (32°F). Intravascular sludging occurs, endothelial leakage begins, and edema develops.55
Nonfreezing injuries are managed in manners similar to those already discussed for the out-of-hospital setting. Freezing injuries are definitively managed by active rewarming without massage. A practical, four-step approach is required to efficiently care for the frozen tissue: 1) stop ongoing insults by removing any constricting or wet clothing, and gently drying the involved area; 2) examine affected skin areas for signs of ischemia, change in texture (e.g., waxy, inflexible, solid), presence of blisters, and loss of sensation; 3) rapidly rewarm affected parts until all skin out to the most distal portions appears perfused and pliable; and 4) protect the rewarmed tissue from evaporative cooling and direct pressure with loosely applied dry dressings and padding.
Frostbite is treated in the ED with rewarming, hydration, wound care, and pain control. Rapid rewarming is the immediate objective once frostbite is discovered. Dry external heat is not appropriate for frostbite. Rewarming should take place in a water bath at 40–42°C (104–107°F), usually for 15–30 minutes until signs of skin reperfusion are evident (i.e., red or purple color and pliable texture). Systemic fluid resuscitation is usually not required, as it is in thermal burns. Once the part is rewarmed it should be elevated, splinted, and the toes and fingers separated with cotton. Rewarming is painful, so systemic analgesic medications are usually required. Debridement of the blisters that form after rewarming is controversial, and is generally not performed in the ED.
Use of thrombolytic agents showed some promise in reducing tissue loss when given within 24 hours of rewarming.70 However, later practice excluded the routine use of these medications for frostbite. Other attempts to use agents to enhance blood flow into and through thawed tissue have not been successful in clinical studies. Heparin, dextran, intra-arterial reserpine, hyperbaric oxygen, and surgical sympathectomy have been used in frostbite treatment without dramatic changes in tissue salvage.
Frostbite is categorized as deep or superficial. Some clinicians use a grading system of 1–4. Classification is made after rewarming. Favorable prognostic signs in superficial frostbite include sensation to pinprick, blisters containing clear fluid, and normal skin color. Poor prognostic signs in deep frostbite include non-deforming hard skin, loss of sensation, blisters filled with dark or bloody fluid, and non-blanching cyanotic skin color.55
It is difficult to define the full extent of cold injury and provide a prognosis for tissue loss at initial presentation. Best practices usually include a “wait and see” approach to amputation that can be quite prolonged since mummification can take up to 3 months. Researchers have studied the value of magnetic resonance imaging and magnetic resonance angiography in predicting tissue viability with limited success.71 Technetium (99mTc) scanning has also been investigated to identify tissue that will require amputation.72 One study of severely frostbitten hand injuries in France suggested that 99mTc scanning in the first few days after rewarming predicts the level of amputation for 84% of cases.73 However, other studies suggest that 99mTc images do not accurately identify eventual levels of gangrene.71 Generally, surgeons are reluctant to decide on an amputation level until complete demarcation several months later.
Patients discharged from the ED can experience long-term symptoms from their cold injuries. Nonfreezing trench foot can result in muscle atrophy and contractures. Deep frostbite is associated with cold sensitivity, increased sweating, pain, hypersensitivity, and skin color change. Superficial frostbite can lead to persistent cold sensitivity, numbness, and loss of sensation. Many cold injuries can result in chronic occupational impairment.
The incidence of accidental hypothermia is a public health issue year-round, especially as regards socially disadvantaged persons. These groups are at risk for being disproportionately affected by winter storms or unusually cold environments, while others may be displaced from their residences by power failures or structural damage. Hypothermia can masquerade as other illnesses, especially in people with other risk factors for altered mental status, syncope, and dysrhythmias. Hypothermia can be overlooked in patients who are homeless, use alcohol or drugs, or have chronic conditions like diabetes, hypothyroidism, and psychiatric illness.74 It can also present in trauma victims, who have more clinically apparent conditions. Triage procedures should include core temperature measurement during cold-weather disasters, which might require use of hypothermia thermometers.
Hypothermia can be a result of infection or a precipitant of infection. In either case, hypothermia associated with infection is associated with higher mortality. Patients with slow rewarming rates (<0.7°C [1.2°F] per hour) and low serum albumin should be carefully assessed for infection and these signs are predictive of outcomes. Hypotension, slow rewarming rates, and bradycardia might be predictors of death during or soon after rewarming.75 Sepsis should be included in the differential diagnosis for patients with all degrees of hypothermia.
Victims of trauma, particularly vehicle crashes, are at risk for hypothermia as a result of delays in discovery, access, extrication, or evacuation. Trauma patients with a core temperature less than 34°C (90°F) might have increased mortality. Hypothermia is associated with electrolyte abnormalities, acid-base disturbances, coagulopathy, and thrombocytopenia. Hypothermic coagulopathy appears to be similar to disseminated intravascular coagulation, and might require management with plasma, clotting factors, and platelets. Partial thromboplastin and prothrombin times should be measured early in the evaluation of hypothermic trauma patients. Thromboelastography (testing the efficiency of blood coagulation) may also be useful in some patients.
Complete blood count, basic metabolic profile, and arterial blood-gas analysis may be helpful in assessing hypothermic patients. Temperature correction of blood-gas results is not necessary during initial management. Severe hypothermia can be associated with lower cardiac output from loss of plasma volume, which decreases in hypothermic patients. The loss of intravascular fluid can potentially decrease renal blood flow by 50%, which may result in acute renal failure. In the absence of significant electrocardiographic changes, correction of low potassium is generally not required, because warming usually reverses the abnormality.
Endotracheal intubation and mechanical ventilation are necessary for patients with respiratory failure or cardiac arrest. Traditional rapid-sequence induction drugs are acceptable for hypothermic patients. However, care is needed to minimize airway and cardiac stimulation, because of the risk of ventricular fibrillation. Life-threatening arrhythmias associated with hypothermia are notoriously difficult to manage.57
Preventing additional heat loss through wet clothing and skin and exposure to ambient air is the first step in the ED management of hypothermia, but core rewarming is the focus of reversing its untoward effects. Passive and active external rewarming techniques are recommended for mild hypothermia, when core temperatures are greater than 32°C (93°F). Covering the patient with blankets accomplishes rewarming by containing and reflecting heat generated by the patient’s own metabolism. Active external rewarming can supplement this approach by using radiant warmers or heated air. These techniques can raise body temperature 0.5–0.8°C (0.9–1.8°F) per hour.52
Although core temperature “afterdrop” is an anecdotal observation when active external rewarming is used on patients with lower core temperatures, the clinical significance of central temperature, electrolyte, and pH changes – reported when the periphery warms faster than the core – remains elusive. Nonetheless, moderate and severe hypothermia requires more aggressive management using active core-rewarming methods, mostly determined by physician expertise and hospital capabilities.
Active internal rewarming by aerosol inhalation of warm humidified oxygen, gastric lavage, bladder lavage, and warm enemas may be appropriate for moderate hypothermia, but are mostly ineffective for victims with severely depressed core temperatures. Thoracic or peritoneal lavage or both are alternate therapeutic options in the ED. In either technique, two intracavitary tubes are placed into one or both pleural spaces or the peritoneal cavity under aseptic conditions. Sterile water or crystalloid fluid warmed to 40–42°C (104–107°F) is allowed to circulate from one tube to the other and drain by gravity.
Indicators of irreversible hypothermic cardiac arrest include serum concentrations of potassium greater than 10 mmol/L or fibrinogen less than 50 mg/dL.74 When potentially reversible cardiac arrest complicates hypothermia, cardiopulmonary bypass (CPB) is the treatment of choice when available.57 The warming rate for CPB is 1–2°C (1.8–3.6°F) every 3–5 minutes. Use of a mechanical chest compression device might be useful when the availability of CPB is delayed.76 Other indications for CPB are solidly frozen extremities, rhabdomyolysis, or failure of other rewarming techniques.74 An alternate to CPB is hemodialysis, which achieves similar rewarming rates, and offers some advantage when electrolyte abnormalities, metabolic acidosis, or renal failure complicate hypothermia.77 However, most hospitals are not equipped to perform emergency CPB or dialysis.
CO poisoning is another hazard that can be confused with nonspecific viral syndromes and other common complaints, as it most commonly presents with headache, nausea, and dizziness.78 People with other medical conditions such as heart or lung disease might be more susceptible to the effects of CO, and present to the ED for treatment of chest pain or shortness of breath.79 Severe CO poisoning contributes to ischemic myocardial injury, and can double mortality.80 Depression, dementia, and psychosis have been reported between 2–28 days after CO poisoning.
Pulse oximetry is unreliable when CO is present, because artificially high saturation readings are associated with carboxyhemoglobin. The standard measurement for the presence or absence of CO is blood carboxyhemoglobin concentration. However, carboxyhemoglobin concentration does not reliably predict delayed neurological sequelae such as an inability to concentrate, learning impairment, memory loss, and abnormal motor function.81
The treatment for CO poisoning is removal from the source and administration of a high concentration of inspired oxygen. The half-life of CO breathing room air is about 5–6 hours. The half-life decreases to less than 2 hours by maximizing oxygen inspiration via face mask, positive-pressure mask, or ventilator. Employing hyperbaric oxygen therapy further hastens CO elimination.78 Hyperbaric chambers traditionally used to treat CO poisoning are located at fixed facilities, but success has also been reported with portable chambers.82
Generally, hyperbaric oxygen therapy is indicated for any history or persistence of syncope, altered mental status, neurological impairment, or myocardial ischemia, as well as for most pregnant patients.78 Hyperbaric treatment might also be indicated in patients found to be at higher risk for long-term cognitive impairment (i.e., patients > 36 years old, exposure >24 hours duration, or “higher” carboxyhemoglobin levels).83 Hyperbaric facilities may be scarce resources that are difficult to access during the aftermath of a winter storm. How long after exposure hyperbaric oxygen therapy is beneficial remains controversial.
In terms of preparedness for epidemics of CO poisoning, one report of an epidemiological spike during a winter storm found that almost four of every five patients arrived between 6:00 PM and 6:00 AM.26 In this study, the eighty-one patients were distributed over 3 days and thirteen hospitals,26 so the impact on individual EDs in that urban area was minimal. However, even at a large university medical center in North Carolina, an epidemic of 200 cases in 1 week resulted in the need for hyperbaric oxygen therapy outstripping available chambers in the area.29 The chamber at Hôpital du Sacre-Cœur de Montréal was used to treat forty-five patients in the first 9 days after the Ice Storm of 1998.9
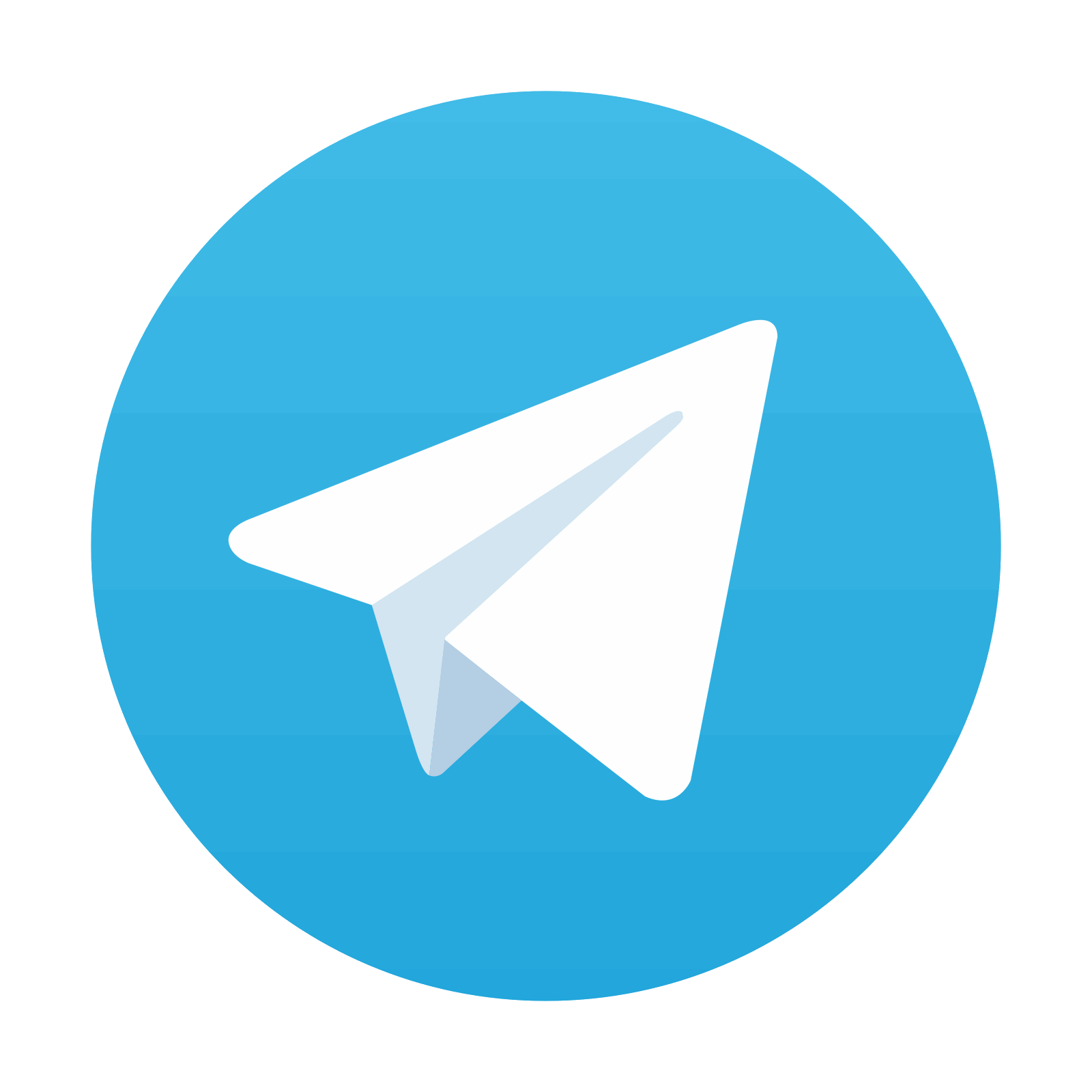
Stay updated, free articles. Join our Telegram channel

Full access? Get Clinical Tree
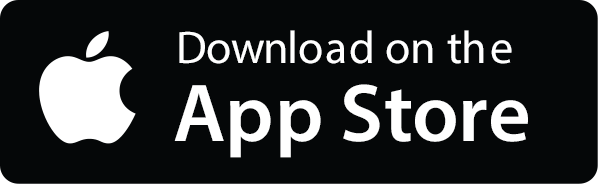
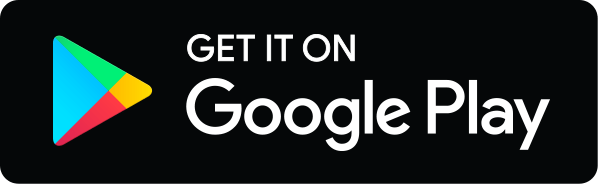