Diverse inciting events lead to acute respiratory distress syndrome (ARDS), including pneumonia, sepsis, pancreatitis, and trauma. These are generally divided into events that directly injure the lung (e.g., pneumonia) or those that indirectly injury the lung (e.g., pancreatitis). Although the method of injury varies, there is a complex and interrelated series of physiologic responses that follow a common pathway to underpin this disease. Inflammatory mediators and direct injury to the alveolar capillary unit lead to increased vascular permeability and protein-rich pulmonary edema, the central finding of ARDS.
Pathophysiologic Changes
Inflammatory Injury
The role of the neutrophil is essential in the pathogenesis of ARDS. The centrality of neutrophils in the pathogenesis of ARDS is suggested in multiple ways. Biopsy and bronchoalveolar lavage (BAL) fluid studies show a significant increase in the volume of neutrophils, and labeled neutrophils transfused into an ARDS patient show a predilection for the lung. The mechanism for neutrophil sequestration in the lung is subject to debate. Only limited evidence supports a significant role for interaction between the neutrophil and the endothelium that is mediated by cell surface adhesion molecules. An alternative theory holds that neutrophil deformability is central. Under normal circumstances, the neutrophil must change shape to transit the pulmonary capillaries and exit the lung vasculature. In ARDS the local inflammatory milieu stiffens the neutrophil, leaving it unable to pass through the vasculature. This physical structural change is thought to be the primary cause of sequestration.
In addition to neutrophil sequestration and inflammatory mediator release in the lung during ARDS, there is evidence that activity is effectively prolonged in ARDS. Normally, the effect of neutrophils is limited by apoptosis. In ARDS, however, BAL fluid studies have demonstrated decreased apoptosis. Further, BAL fluid from ARDS patients can actually inhibit neutrophil apoptosis ex vivo.
The neutrophil acts on both the epithelium and the endothelium, creating a vicious inflammatory cycle. After either direct injury to the lung or systemic inflammation, neutrophils in the pulmonary capillaries are activated. The cells then release proteases, various cytokines, and reactive oxygen species that are cytotoxic. The resultant endothelial damage leads to the breakdown in the capillary wall, allowing adherent platelets to interact directly with neutrophils, further exacerbating cytokine release and recruiting additional neutrophils, thus initiating inflammatory lung injury. Furthermore, platelet activation leads to release of additional inflammatory cytokines and promotes fibrin formation.
It is important to note, however, that while neutrophils play a significant role in ARDS, they are not necessarily essential. The inflammatory cycle of ARDS is complex, with multiple interrelated signalers. Among the hallmarks of ARDS is an early increase in the appearance of immature alveolar macrophages whose influx, in turn, correlates with the severity of ARDS. Stressed fibroblasts, epithelial cells, endothelial cells, and local inflammatory cells release an array of inflammatory products. Both direct and indirect insults to the lung lead monocytes and macrophages to produce tumor necrosis factor α (TNF-α) and interleukin-1 (IL-1) (75). These cytokines act locally and lead to production of IL-8. This leads to both neutrophil recruitment and propagation of a local inflammatory environment. In addition, stressed macrophages, as well as endothelial and epithelial cells, release reactive oxygen and nitrogen species that can directly injure lung tissue. Another precipitant in the cycle of inflammation is the coagulation cascade. In ARDS, activation of this process is enhanced, while the fibrinolytic cascade is downregulated. As a result, an intravascular clot forms, precipitating microthrombosis. Both thrombin and fibrin production independently lead to further exacerbation of the neutrophil-mediated inflammatory cascade.
Alveolar-Capillary Barrier Dysfunction
Injury to both the alveolar epithelium and the capillary endothelium are necessary in ARDS. The result is dysfunction in the alveolar-capillary barrier, leading to movement of intravascular proteins into the alveolar space. This “leakage” is the defining pathophysiologic feature of ARDS.
The alveolar-capillary membrane has the essential role of regulating the volume and movement of proteins and fluid into and out of the alveolar space and thus maintaining surface area for gas transport. Individual type I pulmonary epithelial cells are connected by tight junctions and form a barrier that opposes the movement of proteins into the alveolus. These cells have a characteristic NA,K-ATPase (sodium-potassium adenosine triphosphatase) transporter on their basolateral surface that removes fluid from the alveolus. The inflammatory mediators released by neutrophils damage endothelial cells, disrupt tight junctions, and accelerate apoptosis of both type 1 and type 2 pneumocytes. Reactive oxygen species and elastases are expressed, leading to the formation of paracellular gaps that further impair already tenuous epithelial membranes. In experimental models, these gaps form within minutes to hours of the initiating insult. The damage allows protein-rich fluid to enter the alveolar space. The destruction of types 1 and 2 pneumocytes occurs through both apoptosis and necrosis. The loss of the Na,K-ATPase on type 1 cells, as well as a reduction in surfactant production by type 2 cells, contributes to the progressive damage.
Experimentally, BAL fluid from ARDS patients induces apoptosis in cultured epithelial cells. In addition, radiotracer studies show an increase in the flux of protein across the capillary and in the protein concentration within the alveolar space.
Other inflammatory mediators also damage the alveolar-capillary membrane. Production of reactive oxygen species leads to fatty acid damage on the endothelial and epithelial wall, leading to increased permeability edema.
Permeability Pulmonary Edema
Movement of fluid into and out of the alveolus is primarily determined by Starling’s law. In essence, the net movement of fluid through a semipermeable membrane is dependent on hydrostatic pressure, colloid osmotic pressure (COP), and the permeability of the membrane.
The capillary endothelium is relatively permeable, allowing protein-rich fluid into the interstitium under physiologic conditions. The density of fluid in the interstitium, however, is only two thirds of that normally contained in the capillary. This high COP favors movement of fluid out of the interstitium and into the capillary, an essential mechanism to protect against the pulmonary edema.
Alveolar epithelium, on the other hand, is impermeable to large molecules. Type 1 pneumocytes, which compose 90% of the cells of the epithelium, facilitate gas exchange and are sensitive to damage. They are interconnected via tight junctions that support this impermeable membrane. When fluid does enter the alveolar space, it is removed with a basolateral Na,K-ATPase–dependent pump on the surface of pneumocytes. An osmotic gradient is created, and water flows into the interstitium.
In ARDS, COP is diminished by the increased permeability of the endothelium, allowing egress of plasma proteins from the capillary to the interstitium. The effect of this diminution in COP is dramatic: a 50% decrease in COP increases lymphatic fluid removal fourfold. In addition, for any given hydrostatic pressure gradient change, a similar decrease in COP leads to a doubling in fluid flux away from the capillary. Even with increased permeability of the endothelial membrane, this decreased gradient is central to initiating and maintaining permeability pulmonary edema. This state is exacerbated by the low oncotic pressure common to the critically ill. Experimentally, pulmonary edema is found to develop more rapidly in low protein states and becomes especially pronounced with administration of crystalloid. Under normal conditions, the pulmonary lymphatics drain fluid from the interstitium via the right lymphatic duct, preventing the development of pulmonary edema. In ARDS, the lymphatic flow can increase tenfold but may still remove only 25% of the edema fluid.
The combination of increased permeability and diminution of the protective clearance mechanisms may lead to overwhelming permeability pulmonary edema. At the same time, the ability of the lung to remove this fluid is hampered. When the removal mechanisms of the lung are overwhelmed, clinically apparent pulmonary edema develops.
Iatrogenic Injury
In ARDS, positive pressure ventilation is almost universally employed. Although lifesaving and necessary, this form of support both worsens preexisting lung injury and creates new injury in healthy lungs. The mechanism of injury, in the end, is very similar to the inflammatory cascade induced by any other form of damage causing ARDS. During ARDS, lung aeration is heterogeneous. Some alveoli are collapsed and do not participate in air exchange, whereas other segments are patent and receive more volume than intended. This dichotomy allows multiple mechanisms of lung injury to occur with mechanical ventilation.
Volutrauma is damage caused by ventilation with large tidal volumes that may induce pulmonary edema and hypoxia via alveolar rupture. Several experiments suggest that it is the volume, which was initially thought to be an effect of pressure, and not the pressure that causes these changes. Atelectrauma is an effect of ventilating at low lung volumes or insufficient end expiratory pressure and is caused by the repeated opening of collapsed alveoli. Pathologically, this process leads to epithelial sloughing, hyaline membrane formation, and pulmonary edema. Atelectrauma causes further diminution in surfactant and creates regional hypoxia. This effect is magnified by the regional differences in alveolar collapse.
Both volutrauma and atelectrauma cause biotrauma, the release of inflammatory mediators. Implicated mediators include neutrophils, IL-6 and IL-8, and TNF. In addition, the damage to the epithelial wall by the aforementioned mechanical forces may lead to bacterial translocation into the capillary space and from there into the circulation. It was once thought that translocation was an important precipitant of systemic inflammation and the multiple organ dysfunction syndrome, but this theory is evoked only rarely in current discussions of pathophysiology.
Pathophysiologic Consequences
Clinically, ARDS manifests as refractory hypoxia, difficulty in ventilation caused by pulmonary hypertension (PH), and a significant reduction in pulmonary compliance.
Ventilation-Perfusion Mismatch and Shunt
Hypoxemia results from several important physiologic derangements: ventilation-perfusion ( <SPAN role=presentation tabIndex=0 id=MathJax-Element-1-Frame class=MathJax style="POSITION: relative" data-mathml='V˙/Q˙’>V˙/Q˙V˙/Q˙
V ˙ / Q ˙
) mismatch causing shunt and decreased diffusion of gas across the alveolar membrane. <SPAN role=presentation tabIndex=0 id=MathJax-Element-2-Frame class=MathJax style="POSITION: relative" data-mathml='V˙/Q˙’>V˙/Q˙V˙/Q˙
V ˙ / Q ˙
mismatch occurs because of the deposition of fibrous tissue and the development of infiltrate in the alveolus (discussed later in the pathology section), pulmonary edema, and significant regional atelectasis. Atelectasis is in part due to the destruction of surfactant-producing type 2 pneumocytes in the alveolar epithelium and atelectotrauma. Surfactant is responsible for decreasing surface tension of the alveoli, thus contributing to patent alveoli. BAL studies show that the composition of surfactant changes early in ARDS, contributing to increased surface tension and atelectasis. Evidence implicates the destruction of type 2 pneumocytes, decreased biosynthesis of surfactant and surfactant proteins by injured but intact type 2 cells, and flooding of the alveoli with protein-rich edema fluid. Atelectasis leads to a decrease in compliance, increased hypoxia, and edema that further impairs the ability of type 2 pneumocytes to produce functional surfactant and increases the shunt fraction.
Decreased diffusion develops because of a widened alveolar septum, widened alveolar epithelium, and as a consequence, increased distance between the alveolar and the epithelial membranes.
Decreased Compliance
The alveolar capillary injury with rampant acute inflammation and accumulation of proteinaceous edema fluid in the alveolar spaces results in very stiff, noncompliant lungs. In the early stages of ARDS, pulmonary edema also contributes. As the syndrome progresses, however, fibrosis develops, and compliance may decrease even in the absence of pulmonary edema or active inflammation. Fibrosis will be discussed more fully later.
Dead Space/Pulmonary Hypertension
The development of PH is common in ARDS and contributes to pulmonary insufficiency by increasing dead space ventilation and hypercarbia. In the era preceding lung protective ventilation, 61% of ARDS patients had evidence of cor pulmonale by transthoracic echocardiography, and nearly all patients had elevated pulmonary artery pressures by pulmonary artery catheter measurement.
Early on in ARDS, PH develops due to hypoxic pulmonary vasoconstriction, VTE, and edema. Edema contributes to PH by compressing vessels, thus increasing resistance. Local production of vasoconstrictors, such as endothelin-1 and thromboxane a 2 , has also been implicated. ARDS also leads to subacute arterial, venous, and lymphatic remodeling, with intima being replaced by collagen and fibrin. This process decreases the cross section of the lumen and contributes to PH. As ARDS progresses, fibrous replacement of the microcirculation is seen, and increased muscularization of arterioles develops. As a result, PH worsens and pathologic changes become more pronounced. In addition, both microthrombi and macrothrombi are common during ARDS and have been reported to be present in 95% and 86%, respectively, of autopsy specimens. It is unclear if these thrombi result from local coagulation abnormalities or embolic phenomena. Regardless, they lead to further vascular remodeling and worsening PH.
The resulting PH causes right ventricular dysfunction and thus reduced cardiac output (CO). The development of cor pulmonale and decreased CO leads to end organ hypoperfusion and further hypoxia.
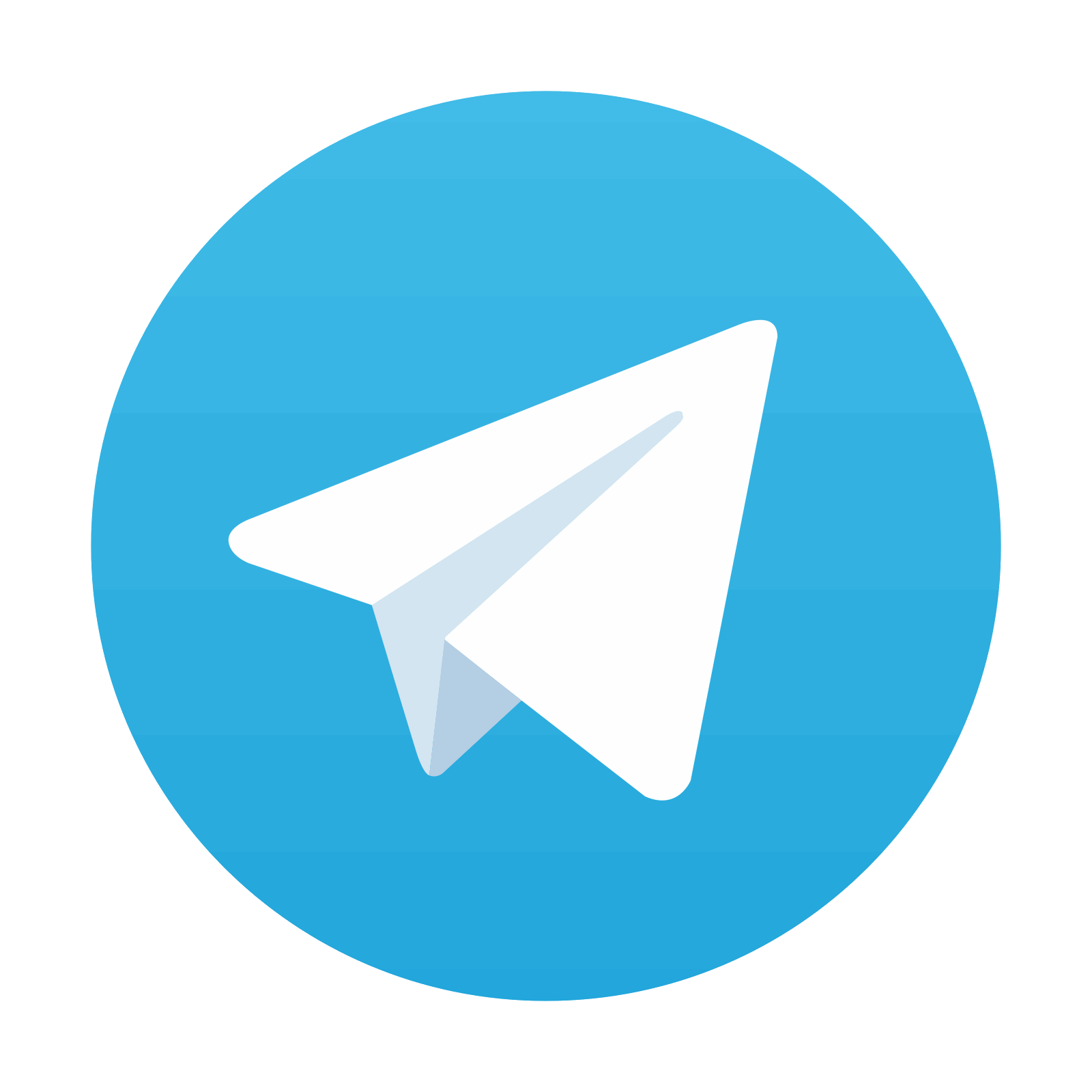
Stay updated, free articles. Join our Telegram channel

Full access? Get Clinical Tree
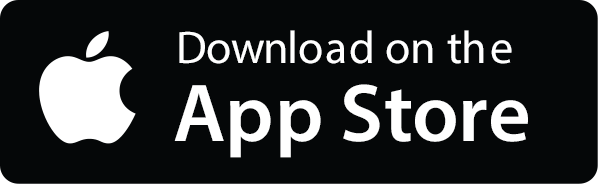
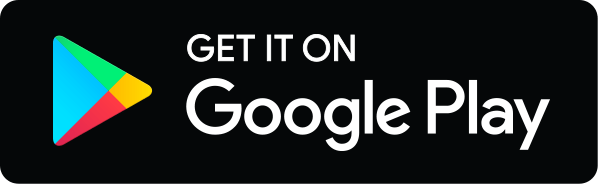