html xmlns=”http://www.w3.org/1999/xhtml” xml:lang=”en”>
Salt, Water, and Brain—What Should the Intensivist Know?
Azra Bihorac, MD, MS, FCCM, FASN
Objectives
- Review the regulatory mechanisms for osmolality and volume homeostasis
- Discuss the epidemiological characteristics of sodium disorders among neurological and neurosurgical critical care patients
- Review the diagnostic approach to hyponatremia and hypernatremia in the ICU
- Identify an appropriate clinical approach for the treatment of hyponatremia and hypernatremia in the ICU
Key words: sodium balance, free water balance, hypernatremia, hyponatremia, neurological and neurosurgical critical care patients, vasopressin, diabetes insipidus
Acknowledgment
Azra Bihorac is supported by grant K23 GM-087709 awarded by National Institute of General Medical Sciences, National Institutes of Health. The content is solely the responsibility of the author and does not necessarily represent the official views of the National Institutes of Health.
Abnormalities of sodium and water homeostasis are common complications encountered in the neurointensive care unit (NICU) that carry severe consequences for patients with neurological problems. The controlling mechanisms of water and sodium balance may become severely disturbed after acute brain injury due to trauma or central nervous system disease and can exacerbate the underlying neurological processes. In this chapter, we discuss the controlling mechanisms for sodium and water imbalance and the clinical approach to diagnosis and treatment of these disorders in the neurological critically ill patient.
Sodium and Water Balance
Water balance is a major determinant of plasma sodium concentration. Total body water (TBW) constitutes between 50% and 60% of total body weight, depending on age and gender, and is divided into 2 major compartments: two-thirds in the intracellular space and one-third in the extracellular space. Water moves between the intracellular and the extracellular fluid (ECF) compartments in response to osmotic forces exerted across the semipermeable cellular membrane. The magnitude of the osmotic force is determined by the concentration of solute particles in the body fluid. Plasma osmolality, defined as the number of osmoles of solute per kilogram of water (mOsm/kg H2O), indicates the osmotic activity of the solutes—the most important being sodium (mmol/L), serum urea nitrogen (SUN) (mg/dL), and glucose (mg/dL). The equation to calculate estimated serum osmolality is
Serum Osmolality (mOsm/kg H2O) = 2(Na) + SUN/2.8 + glucose/18.
Sodium is the major determinant of plasma osmolality because it cannot freely diffuse across the cellular membrane. The total sodium content is the principal determinant of the relative volumes of the intracellular and extracellular compartments. Mechanisms regulating effective circulating volumes are directed at the maintenance of adequate total body sodium content. Mechanisms aimed at regulation of water balance, in contrast, maintain constant plasma osmolality by determining plasma sodium concentration. Hence, water and sodium balance are regulated through 2 systems: baroreceptors regulate multiple vasopressor hormones, the vascular system, and the kidneys to moderate short- and long-term responses to changes in effective circulating volumes through sodium conservation and excretion, whereas osmoreceptors and the hypothalamic–pituitary axis control plasma osmolality. Hypervolemia and hypovolemia reflect disorders of total sodium balance, whereas hypernatremia and hyponatremia reflect disorders of water balance and osmolality.
Regulation of Sodium Balance and Effective Plasma Volume
Because total body sodium content ensures maintenance of the ECF volume and adequate blood pressure, numerous mechanisms are involved in the renal control of sodium excretion.1 Renal Na+ excretion varies depending on the effective circulating volume, from urine Na+ concentration as low as 1 mmol/L in the presence of volume depletion to more than 100 mmol/L with effective volume expansion.2 Low effective circulating volume also (1) activates the renin–angiotensin–aldosterone system, which increases sodium reabsorption at the proximal tubule, distal tubule, and collecting duct, and (2) induces the release of norepinephrine, which decreases the glomerular filtration rate to further stimulate sodium reabsorption at the proximal tubule. These mechanisms all lead to increased sodium and water retention to increase circulating volume. In contrast, expansion of the ECF volume results in an appropriate increase in urinary sodium excretion induced by suppression of the renin–angiotensin–aldosterone system, a small elevation in the glomerular filtration rate, and the effect of natriuretic hormones, like atrial natriuretic peptide.3
Regulation of Water Balance and Plasma Osmolality
Plasma osmolality (Posm) is regulated strictly by water intake and loss primarily by thirst and the ability of renal tubules to conserve or excrete water. In humans, osmolality of the ECF is tightly regulated between 280 and 290 mOsm/ kg. Changes in ECF osmolality can cause water to flow across cell membranes to equilibrate with intracellular osmolality, leading potentially to the alteration of cell volume, intracellular ionic strength, and cell death. A myriad of physiological responses that actively oppose osmotic perturbations in order to restore ECF osmolality to an osmotic set point near 300 mOsm/kg are promptly engaged in response to changes in plasma osmolality.4 An increase in ECF osmolality stimulates the sensation of thirst to promote intake of water and release of the 9-amino acid peptide hormone arginine vasopressin (AVP) to enhance water reabsorption in the kidney. In contrast, ECF hypo-osmolality suppresses basal AVP secretion and stimulates diuresis.5,6 The primary central osmoreceptors that modulate thirst and AVP release are located in regions of the brain that are devoid of a blood–brain barrier, such as the hypothalamus and the circumventricular organs.7,8 Magnocellular neurosecretory cells in the hypothalamic supraoptic nucleus9,10 have been proposed to serve as primary osmoreceptors in humans11,12 through the synthesis of AVP, a major regulator of free water excretion by the kidney. The synthesized peptide is enzymatically cleaved from its prohormone and transported to the posterior pituitary, where it is stored within neurosecretory granules. The increase in plasma osmolality causes secretion of AVP into the bloodstream, leading to the activation of AVP V2 receptors in the kidney and insertion of aquaporin-2 water channels into the apical membranes of collecting tubule principal cells.13 As a result, the increase in water permeability of the collecting duct allows free water conservation and restores the plasma osmolality set point to normal limits.13
Hyponatremia
Hyponatremia is the most common electrolyte disorder encountered in clinical medicine,14 with close to a million patients hospitalized with a principal or secondary diagnosis of hyponatremia every year in the United States. The annual cost of managing patients with hyponatremia has been estimated at $3.6 billion.14 In some disease states, such as congestive heart failure, hyponatremia is an independent risk factor for increased mortality.15,16 The prevalence of hyponatremia in the neurosurgical population has been reported to be as high as 50%.17-19 Given the effect of hyponatremia on intracellular osmolality, neurosurgical patients are at increased risk for cerebral edema, seizures, vasospasm, and death, all of which can result from the inappropriate treatment of hyponatremia.20,21
Evaluation of Hyponatremia
Although in clinical practice most physicians would consider hyponatremia if the serum sodium concentration ([Na+]) is less than 135 mmol/L, the clinically significant level of hyponatremia has not been clearly defined.14,22 The association between hyponatremia and adverse outcomes is proportional to its severity.23,24 In a prospective study of patients hospitalized for medical illnesses other than dysnatremia, [Na+] less than 130 mmol/L was associated with a significant increase in mortality.25 In addition, even after investigators controlled for underlying diagnoses, hyponatremic patients (average [Na+] 125 mmol/L) were more likely to die even after discharge compared with normonatremic patients.26 Appropriate correction of [Na+] as low as 2 mmol/L improved survival among patients with hyponatremia.27 Additional morbidity is associated with [Na+] less than 130 mmol/L,14,22,28 including increased risk for falls and seizures.29,30 In patients with subarachnoid hemorrhage (SAH), hyponatremia has a clear association with increased morbidity including cerebral ischemia,31,32 Glasgow Outcome Scale, vegetative state, and severe disability.33
Diagnostic Workup for Hyponatremia
No single standard reference test exists for the evaluation of hyponatremia; rather, a systematic clinical algorithm that combines physical examination and laboratory measurements is warranted.18,20,22,34-36 In the NICU, this approach is especially important to distinguish between the syndrome of inappropriate secretion of antidiuretic hormone (SIADH) and cerebral salt wasting (CSW). Criteria for SIADH proposed by Janicic and Verbalis22 include Posm less than 275 mOsm/kg, inappropriate urinary concentration (urine osmolality [Uosm] >100 mOsm/kg), clinical euvolemia (absence of orthostasis, tachycardia, decreased skin turgor, dry mucous membranes, edema, and ascites), elevated urinary sodium excretion with normal salt and water intake, and absence of other causes of euvolemic hypo-osmolality (hypothyroidism or adrenal insufficiency). However, these criteria are insufficient in distinguishing between SIADH and CSW in the NICU. In a prospective study, 10 of 12 neurosurgical patients who met the criteria for SIADH ([Na+] <135 mmol/L, Posm <280 mOsm/kg, urine sodium >25 mmol/L, and Uosm >Posm) had low blood cell mass, low plasma volume, and low total blood volume compared with controls.37 Because appropriate assessment of extracellular volume status is the key to distinguishing between SIADH and CWS in the NICU34,37-40 and given that determination using physical examination alone with sensitivity of approximately 40% may be inadequate, more advanced hemodynamic monitoring in the ICU may be warranted.39 Although uric acid has been used to distinguish SIADH from other causes of hyponatremia by using a level of less than 4 mg/dL (positive predictive value for SIADH of 73%), the definition for SIADH used in these studies was broad and most likely included patients with CSW.41
To distinguish between SIADH and CSW, central venous pressure (CVP) can be used to determine volume status. Damaraju et al39 treated hyponatremia based on CVP values: Hypovolemic patients with CVP less than 5 cm H2O were given normal saline (50 mL/kg/d) and salt (12 g/d), and normovolemic patients with CVP of 6 to 10 cm H2O were given normal saline with 12 g of salt per day. In addition, patients with anemia (hematocrit <27%) were administered whole blood. No patients were found to be hypervolemic (CVP >10 cm H2O). Seventy-three percent of patients corrected their [Na+] within 72 hours and an additional 12% within the following 24 to 48 hours. Two of the 3 nonresponders were found to have severe dehydration on blood volume measurements.39 Another prospective study used CVP to categorize 7 hyponatremic patients as SIADH (CVP 6-10 cm H2O) and 4 patients as CSW (CVP <6 cm H2O).42
Patients with hyponatremia and SAH present a special challenge, given that several studies have demonstrated the association between fluid restriction or dehydration with symptomatic vasospasm.32,43 Traditionally, hyponatremia in SAH patients has been attributed to SIADH.18 However, recent studies have demonstrated that hyponatremic SAH patients often have a negative sodium balance (loss of sodium in urine greater than intake of sodium per day) and hypovolemia.31
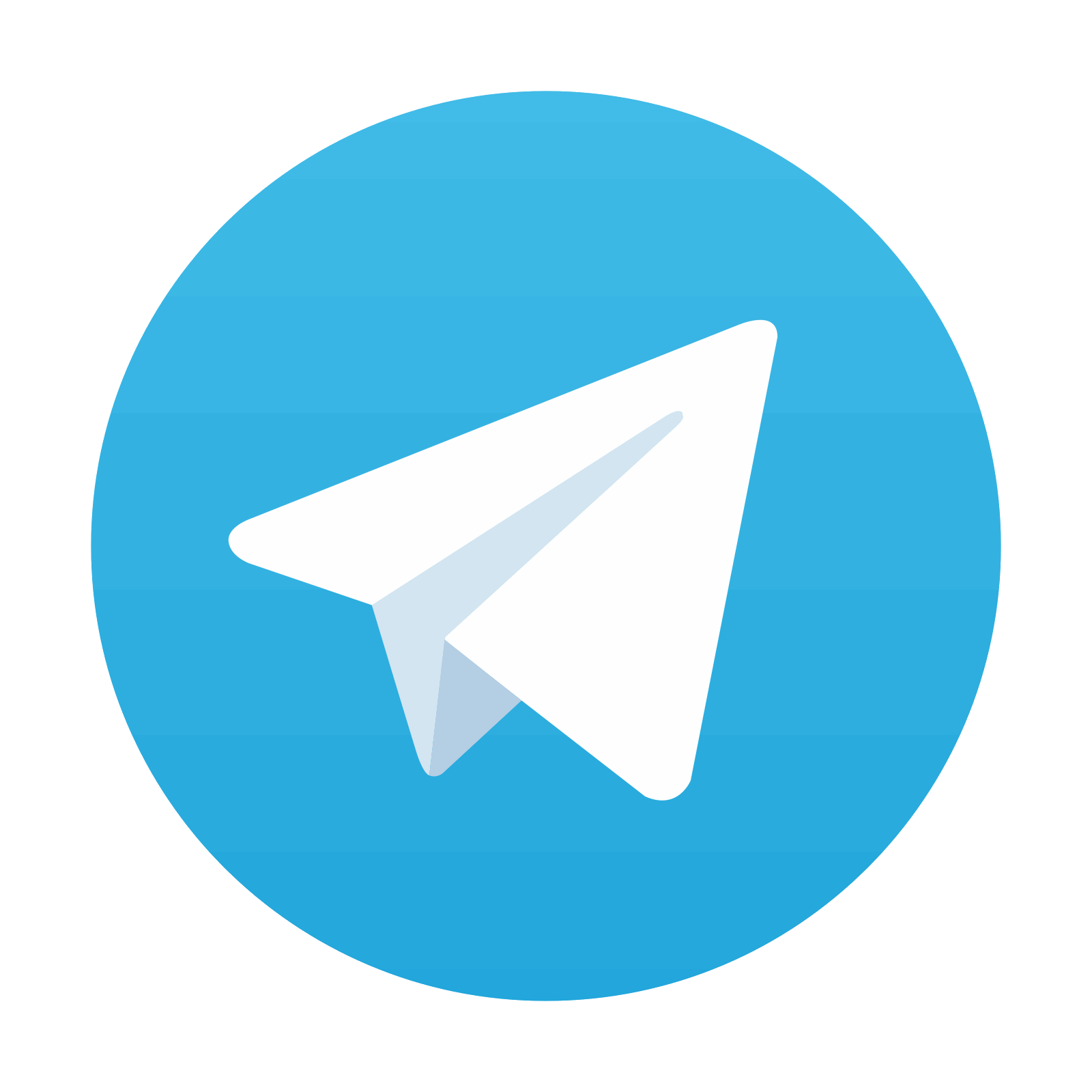
Stay updated, free articles. Join our Telegram channel

Full access? Get Clinical Tree
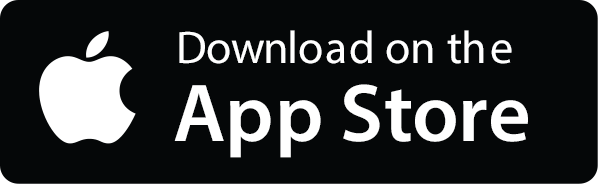
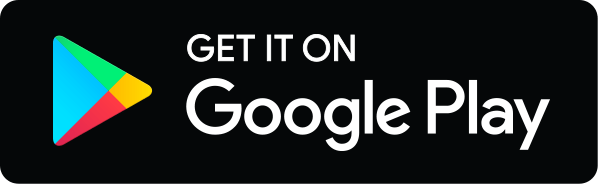