KEY POINTS
-
Ventilator-induced lung injury (VILI) may occur with both lung volumes that lead to overdistention of lung units (volutrauma) or with low distending pressures that allow the lung to be recruited and derecruited (atelectrauma).
-
VILI may cause injury in previously healthy regions of lung, and may also lead to multiorgan dysfunction.
-
To reduce the risk of VILI, limitation of end-inspiratory stretch using low tidal volumes ∼6 mL/kg and limiting plateau pressure (Pplat) <30 cm H2O should be used in treating most patients with acute lung injury (ALI) or acute respiratory distress syndrome (ARDS). Higher Pplat may be used in patients with poorly compliant chest walls.
-
The appropriate level of positive end-expiratory pressure remains to be determined, but levels of PEEP that minimize atelectasis may be beneficial.
-
Permissive hypoventilation (hypercapnia) may be a necessary component of a lung-protective ventilator strategy.
-
The penetrance of lung-protective ventilation strategies into clinical practice is improving.
There is consistent and convincing evidence that mechanical ventilation, particularly in the setting of lung injury, can contribute to functional and structural alterations in the lung. The experimental evidence has also led to the notion that mechanical ventilation not only perpetuates lung injury, but also contributes to both the morbidity and mortality of the acute respiratory distress syndrome (ARDS). Concern surrounding ventilator-induced lung injury (VILI) culminated in a consensus conference in 1993 that recommended (based solely on studies in animal models of ARDS) tidal volumes be limited to the range of 5 to 7 mL/kg and plateau pressures less than 35 cm H2O.1 It would be 8 years until the recommendations of the consensus group were affirmed by a randomized controlled trial demonstrating that a lung-protective strategy led to a decrease in mortality in patients with acute lung injury.2 After initial hesitations about the incorporation of these concepts into widespread clinical practice,3 lower tidal volumes and higher levels of positive end-expiratory pressure (PEEP) are being used widely to minimize VILI.4,5
The objectives of this chapter are to review current concepts of VILI and provide the rationale for lung-protective ventilation strategies. Since most studies evaluating VILI have focused on ARDS, the relevant features of ARDS as it pertains to VILI will be reviewed first. Then, the concept of lung-protective ventilation strategies will be discussed, and pertinent studies evaluating these newer strategies in patients with ARDS will be presented. Recommendations based on current clinical evidence, and when this is lacking best experimental evidence, will also be presented (Table 51-1).
Goals of Mechanical Ventilation Modified to Reduce the Risk of Ventilator-Induced Lung Injury
Oxygenation |
Maintain PaO2 between 60 and 80 mm Hg or maintain oxygen saturation between 88% and 95%. |
Ensure adequate oxygen delivery |
Avoid overdistention |
Limit tidal volumes to 6 mL/kg PBW |
Limit plateau pressure to <30 cm H2O. Consider higher plateau pressure in presence of high chest wall elastance (ie, pleural effusion, obesity, increased abdominal pressure) |
Recruitment maneuvers |
Recruitment maneuver after each disconnection from the ventilator in patients with severe ARDS. |
Positive end-expiratory pressure |
Use higher PEEP levels (ie >10 cm H2O) only in ARDS; these may be more effective in patients who have potential for recruitment. |
Ventilation |
Allow increased PaCO2 if required to minimize VT and Pplat |
Accept pH as low as ∼7.2, if not contraindicated (eg, traumatic brain injury) |
ACUTE RESPIRATORY DISTRESS SYNDROME
ARDS is characterized by endothelial and epithelial cellular injury. This loss of integrity of the alveolar-capillary membrane results in high-permeability pulmonary edema and formation of hyaline membranes. Injury to type II pneumocytes also occurs, along with alterations in surfactant function. Although plain chest radiographs suggest that lung damage is uniformly distributed, thoracic computed tomographic (CT) examination of the thorax of patients with ARDS has demonstrated that the airspace disease is patchy,6,7 with marked heterogeneity and regional differences in lung injury. Regions of lung with airspace disease are juxtaposed to adjacent areas with normal-appearing alveoli. In addition, an exaggerated vertical gradient of lung inflation has been demonstrated in ARDS, with compression of alveoli and a decrease in aerated lung as one progresses from nondependent to more dependent lung regions.8 As emphasized by Mead and coworkers in the early 1970s, it is likely this degree of heterogeneity of the lung injury that makes the lung particularly susceptible to the effects of ventilator-induced injury.9 The heterogeneity of the injury is also responsible for the decrease in lung compliance that characterizes ARDS. It is worth emphasizing that this loss of compliance is due to a functional reduction in alveolar units and not due to the development of “stiff” lungs. Indeed the recognition that ARDS is characterized by a loss of functional lung units with preservation of other alveoli resulting in normal lung specific lung compliance is central to the current notion of lung-protection strategies.10 In many respects owing to the reduction in effective lung volume, the 70-kg adult patient with ARDS must be treated, from the pulmonary point of view, as a 30-kg pediatric patient. Consequently the use of traditional tidal volumes of 10 to 15 mL/kg (700-900 mL in our 70-kg patient) would be inappropriate and will result in overdistention of lung units with relatively normal compliance.
The need to modify the approach to mechanical ventilation in ARDS is further emphasized by three decades of investigations that demonstrate that overdistention of lung units may itself lead to lung injury identical to that seen in ARDS. ARDS is also a syndrome characterized by inflammation of the lung with various cytokines and other mediators thought to play a major role. In recent years, there has been a large body of evidence indicating that mechanical ventilation may have an impact on this aspect of the pathophysiology of ARDS, and indeed there is the suggestion that the improvement in mortality with lung-protective strategies may be partly due to a reduction in release of various mediators by these strategies.
VENTILATOR-INDUCED LUNG INJURY
-
Macroscopic injury caused by mechanical ventilation is termed barotrauma. The severity of lung injury and excessive inflation pressures associated with high transpulmonary pressures appear to be risk factors. In clinical practice, plateau pressure (Pplat) is often used as a surrogate of transpulmonary pressure (Ptp) to assess the propensity for development of VILI. However, Pplat can be very misleading as a surrogate for Ptp in view of range of different chest wall compliances in ventilated patients.
Recent evidence suggests that mechanical ventilation may have both regional and systemic effects. VILI may be broadly classified into macroscopic and microscopic injury (Table 51-2). Macroscopic injury consists of what has been classically described as barotrauma. Pneumothorax, pneumomediastinum, pneumoperitoneum, and subcutaneous emphysema are recognized complications of mechanical ventilation, and are characterized by the presence of extraalveolar air.11 Gattinoni and coworkers have described the appearance of bullae and cystic parenchymal lesions located predominantly in the dependent (dorsal) lung regions.6 These lesions are often occult and are not readily detected on plain chest radiographs.
The Scope of Ventilator-Induced Lung Injury
Oxygen toxicity |
Tracheal and upper airway injury |
Macroscopic |
Pneumothorax |
Pneumomediastinum |
Pneumopericardium |
Pneumoperitoneum |
Subcutaneous emphysema |
Parenchymal emphysema |
Cystic lung spaces |
Microscopic |
Regional |
Epithelial/endothelial activation (inflammatory mediators release) and injury |
Damage to the alveolar-capillary barrier and vascular permeability decreases alveolar fluid clearance |
Surfactant dysfunction |
Bronchiolar injury |
Leukocytes sequestration and activation |
Fibrosis (late phase of ARDS) |
Biotrauma |
Systemic |
Multisystem organ dysfunction |
Macroscopic barotrauma correlates with a variety of factors. In a retrospective study in 139 intubated patients, barotrauma occurred in 34 patients.12 Peak airway pressure, level of PEEP, tidal volume, and minute ventilation correlated with the development of barotrauma. However, in a subsequent prospective study of 168 patients over a 1-year period, only the presence of ARDS was associated with the development of barotrauma.12,13 The relationship of PEEP to the development of extraalveolar air is inconsistent.13,14 Patients with severe underlying lung disease often require higher levels of PEEP to maintain oxygenation, and it is possible that it is the underlying lung disease in such patients that explains the correlation between air leaks and PEEP levels. Eisner and colleagues, using data from the ARDS Network trial, reported that higher PEEP was associated with an increased risk of barotrauma (relative risk = 1.5; 95% confidence interval [CI] 0.98-2.3).15 More recently however, two multicenter clinical trials failed to demonstrate an association between higher PEEP levels and barotrauma.16,17 Whether patient-ventilator interaction may lead to barotrauma is suggested by a recent clinical trial testing the efficacy of the neuromuscular blocking agent (NMBA), cisatracurium in severe ARDS patients. The probability of death at 90 days and incidence of pneumothorax were less in the NMBA group.18 Ideally, these results should to be confirmed in future trials. Of particular importance, sedation and paralysis are risk factors for the development of muscular weakness and prolonged ventilator dependence in ARDS.19-21
There is also evidence that increased blood flow through the lungs can lead to greater VILI manifest by severe hemorrhage, increased filtration coefficient, and heavier lungs.22 Injury to conducting airways could also potentially lead to an increase in regional airways resistance, with resultant gas trapping and progressive downstream regional lung distention. Regions of local superinfection and resultant inflammation may intensify bronchiolar injury. Goldstein and associates used a piglet model and found cystic lung changes and areas of bronchiolectasis in animals that received intrabronchial inoculation with Escherichia coli.23 The importance of bronchiolectasis in the pathogenesis of VILI is further highlighted by observations that dead space (a potential prognostic marker in ARDS24) correlated with the presence and severity of bronchiolar injury and dilation.25 In summary, macroscopic lung injury represents a continuum from airspace enlargement through interstitial emphysema and eventually to radiographically apparent extraalveolar air.
-
Numerous animal and human studies demonstrate that in otherwise healthy lungs mechanical ventilation with large tidal volumes may initiate lung inflammation and may lead to development of acute lung injury (ALI)/ARDS. Moreover, in established ARDS, tidal volume reduction associated with the application of PEEP attenuates these phenomena.
Shortly after the institution of invasive positive pressure ventilation, the development of lung damage was observed in animals ventilated for prolonged periods. The term “respirator lung” was coined to describe the functional and histologic features.26 In 1974, Webb and Tierney graphically illustrated the deleterious effects of mechanical ventilation in rats using varying levels of peak airway pressure and PEEP.27 Animals ventilated using low peak airway pressures (14 cm H2O and no PEEP) had no pathologic or physiologic changes. In contrast, rats ventilated with peak pressures of 30 cm H2O and no PEEP had perivascular edema and alveolar edema. These findings were magnified in rats ventilated with peak pressures of 45 cm H2O. Alveolar and perivascular edema developed, along with severe hypoxemia, decreased dynamic compliance, and obvious gross anatomic changes (Fig. 51-1). Interestingly, rats ventilated using 10 cm H2O of PEEP and peak pressures of 45 cm H2O had no alveolar edema (see Fig. 51-1, center). This latter finding led to the concept of a protective effect of PEEP, which will be discussed later.
FIGURE 51-1
Illustration of recruitable versus nonrecruitable lung using CT imaging of the chest. A. The frequency distribution of the overall study group according to the percentage of potentially recruitable lung (expressed as the percentage of total lung weight). The percentage of potentially recruitable lung was defined as the proportion of lung tissue in which aeration was restored at airway pressures between 5 and 45 cm H2O. B. CT slices obtained 2 cm above the dome of the diaphragm at airway pressures of 5 (left) and 45 (right) cm H2O from a patient with a lower percentage of potentially recruitable lung. The percentage of potentially recruitable lung was 4%, and the proportion of consolidated lung tissue was 33% of the total lung weight. C. CT slices obtained 2 cm above the dome of the diaphragm at airway pressures of 5 (left) and 45 (right) cm H2O from a patient in the group with a higher percentage of potentially recruitable lung. The percentage of potentially recruitable lung was 37%, and the proportion of consolidated lung tissue was 27% of the total lung weight. (Modified with permission from Gattinoni L, et al. Lung recruitment in patients with the acute respiratory distress syndrome. N Engl J Med. April 27, 2006;354(17):1775-1786.)
Subsequent investigations have demonstrated that mechanical ventilation, even at modest airway pressures, is capable of producing functional impairment of the lung with loss of integrity of the alveolar-capillary barrier, surfactant dysfunction, and parenchymal damage that mimics the histologic appearance of ARDS. These observations have led investigators to speculate that mechanical ventilation itself could be contributing to the lung injury, morbidity, and mortality in patients with acute respiratory failure.
Numerous human studies demonstrate that mechanical ventilation with large tidal volumes is capable of inciting lung inflammation and may lead to the development of ALI/ARDS.28,29 Patients with underlying lung disease are particularly prone to the development of macroscopic barotrauma. Asthma, chronic obstructive pulmonary disease (COPD), and pneumonia have all been identified as risk factors.12
-
Transpulmonary pressure—the pressure difference between the alveoli and the pleural space is the effective alveolar distending pressure and is the pressure most closely related to the development of VILI.
-
Plateau pressure is often used as a surrogate for transpulmonary pressure with the requisite that the chest wall elastance be near normal, and respiratory muscles are relaxed.
-
Large tidal volumes inducing overdistension of aerated lung regions causes VILI.
-
Tidal hyperinflation could occur in a subset of ARDS patients even with tidal volumes less than 6 mL/kg.
High airway pressures in and of themselves do not produce alveolar disruption. Trumpet players repetitively generate large airway pressure, up to 150 cm H2O, without pulmonary sequelae.30 The critical factor causing injury is lung overdistention, and hence the term—volutrauma. Increased lung stretch is best assessed in the pressure domain by the transpulmonary pressure (alveolar minus pleural pressure). Trumpet players generate such high airway pressures by generating high pleural pressures and alveolar pressures such that the transpulmonary pressure is not elevated. In critically ill patients, undergoing volume control mechanical ventilation, peak inspiratory pressure (PIP) is often used at the bedside as a surrogate for the degree of lung inflation. However, PIP is dependent on the resistive pressure drop arising from flow across the endotracheal tube and conducting airways, and is also dependent on the compliance of the respiratory system. Consequently, increased PIP may not be indicative of overdistension; plateau pressure obtained after occlusion of the airway following inspiration reflects alveolar pressure, but does not directly reflect pleural pressure due to the pressure dissipated in distending the chest wall and thus is not an accurate surrogate of lung distension, becoming less accurate in patients with stiff chest walls (eg, patients with ascites). Patients with hypoxic respiratory failure are commonly ventilated with very high inspiratory pressures, which may produce transpulmonary pressures >20 to 25 cm H2O, values occurring at TLC,11 and hence lead to overdistention of lung units and lung injury.
Direct evidence for volutrauma stems from several observations. Open-chest animals whose lungs were ventilated with peak pressures of 15 cm H2O for 15 minutes had an 850% increase in their filtration coefficient. In contrast, the filtration coefficients of closed-chest animals increased by 31% with a PIP of 30 cm H2O, and by 450% with a PIP of 45 cm H2O. Animals in which lung expansion was limited did not develop lung edema during ventilation, even with peak airway pressures up to 45 cm H2O. The concept of volutrauma as opposed to a pure airway pressure-related effect was further supported in a study that compared the development of edema using negative-pressure ventilation and high tidal volumes, to that using PPV to achieve similar tidal volumes.31 The amount of edema obtained using negative-pressure ventilation was comparable to that in the animals ventilated with PPV at the same tidal volumes. These studies first established the importance of lung inflation, and second they demonstrated a dose-response relationship, with larger transpulmonary pressures and tidal volumes producing an increased lung injury and edema.32 In a very illustrative histologic study using electron microscopy, overdistention of the lung increased the number of endothelial and epithelial breaks and contributed to rupture of the blood-gas barrier and separation of the epithelium from its underlying basement membrane (Fig. 51-2).139
FIGURE 51-2
Scanning electron micrographs illustrating disruptions of the blood-gas barrier in rabbit lungs perfused at 20 cm H2O transpulmonary pressure and 52.5 cm H2O capillary transmural pressure. Examples of both endothelial (A) and epithelial stress failure (B) are shown. A. Adjacent capillaries with several areas of complete rupture of the blood-gas barrier (arrows) can be seen at various angles relative to the capillary axis; they have resulted in red blood cells and proteinaceous material accumulating on the alveolar surface. B. Round rupture involving only the epithelial layer (arrow). (Reproduced with permission from Fu Z, et al. High lung volume increases stress failure in pulmonary capillaries. J Appl Physiol. Jul y 1992;73(1):123-133.)
In humans, the risk of lung overdistention may vary from patient to patient. CT scan studies classified ARDS into focal (36% of patients), diffuse (23%), and patchy (41%), based on the pattern of distribution of loss of aeration.33-35 A different response to recruitment and overdistension was seen across the different lung CT morphologies. Focal disease as compared to diffuse loss of aeration morphology was associated with a greater risk of overdistension.36 Recent studies of ARDS patients showed that limiting tidal volume to <6 mL/kg predicted body weight (PBW) and plateau pressure to <30 cm H2O may not be sufficient to limit VILI in patients who have a larger nonaerated compartment, as demonstrated by lung CT.37 Finally although a relationship between decreasing mortality as Pplat declines from high to low levels appears to exist, a safe Pplat threshold value has not been established.
A mechanical ventilation strategy that incorporates decreased tidal volumes and minute ventilation is often accompanied by increased PaCO2 and in this context is termed permissive hypercapnia. Permissive hypercapnia does not represent a method of mechanical ventilation per se; rather, it is the consequence of a strategy that limits lung volume excursions to minimize alveolar overdistention and hence VILI. Hickling and associates described the use of a pressure-limited strategy and permissive hypercapnia in an uncontrolled study in which they showed that ARDS patients treated with permissive hypercapnia had a lower mortality than would have been predicted from the APACHE II score.38,39 These were landmark studies, but used historical controls, the method of ventilation was not well defined, and efforts were made concurrently to limit oxygen toxicity. Therefore, these studies suggested, but did not conclusively prove, that a pressure-limited strategy and disregard for the partial arterial pressure of carbon dioxide (PaCO2) improved outcome. Importantly, serious side effects of an elevation of PaCO2 were not observed in either study.
The physiologic consequences of hypercapnia and respiratory acidosis have been reviewed extensively.40,41 At present, the only absolute contraindication to a rise in PaCO2 is increased intracranial pressure, although acute hypercarbia may have adverse effects on the fetus. Indeed it has been postulated that hypercapnia may attenuate the severity of ALI.41-44 Hypercapnic acidosis has been shown to attenuate protein leakage, lung edema, lung lavage inflammatory mediators, and lung injury score, and preserve oxygenation and lung compliance in several models of lung injury.1,44-46
In summary, there is persuasive experimental evidence to support the concept of lung overdistention as a major component of VILI. Studies have demonstrated a dose-response relationship, in which both higher inflation pressures and tidal volumes administered over progressively longer periods of time produce a graded severity of lung injury. Second, VILI has been demonstrated in many animal models, negating the possibility that these observations were due to a species-specific effect.
-
Ventilation of lungs with atelectatic regions can induce damage in nonatelectatic regions.
-
Atelectasis is associated with ultrastructural cellular damage and activation of the intracellular signaling pathway associated with lung injury.
-
PEEP has a number of effects: (1) increases the functional residual capacity (FRC) or end-expiratory lung volume (EELV) after lung injury (alveolar recruitment). (2) Partially aerated lung units or those that collapse at the end of a tidal breath may be kept patent during the entire respiratory cycle (prevents derecruitment). (3) lung units that are already aerated may be overdistended (overinflation). Both derecruitment and overinflation may contribute to VILI and should be minimized.
-
PEEP-induced alveolar recruitment is quite variable among ALI/ARDS patients.
-
PEEP may induce hyperinflation in a subset of ARDS patient despite the use of tidal volumes under 6 mL/kg.
-
Application of adequate levels of PEEP may be beneficial in patients who have a demonstrable high percentage of recruitable lung.
The precise impact of PEEP on VILI is controversial and likely multifactorial. The application of PEEP is known to have effects on distribution of lung water, pulmonary hemodynamics, and (pulmonary) respiratory system compliance.35,47,48 In addition to lung overdistention, ventilation at low lung volumes may also lead to lung injury.
In patients with ARDS, providing PEEP may have one of four effects on the state of lung inflation. First, lung units that are already aerated may become overdistended, which could contribute to VILI (overdistension). Second, partially aerated lung units or those that collapse at the end of a tidal breath may be kept patent during the entire respiratory cycle (prevents derecruitment). Third, previously closed alveoli may be recruited, leading to an increase in functional lung and resulting in an increase in total lung compliance (alveolar recruitment). Fourth, in the absence of adequate levels of PEEP, VILI is associated with distal airway injury in both atelectatic and nonatelectatic lung regions (prevents distal airways injury). The latter two points deserve further emphasis.
To understand the physiological effects of PEEP on the lung and respiratory system, it is useful to consider the respiratory system pressure-volume (P-V) curve, which plots changes in volume versus changes in pressure. At the beginning of the lung inflation, a lower inflection point (LIP) has been described in patients with ARDS, reflecting the point where there is a rapid increase in volume in response to the incremental change in pressure.49 However, using this point to set PEEP has problems, as a unique value of PEEP corresponding the pressure at which all alveoli will be opened does not exist. Rather there is a progressive increase in alveolar patency until the upper inflection point (UIP) is reached. At this point overinflation of alveoli predominates. Examination of the pressure-volume curve, however, does not inform the observer about regional differences in lung inflation where regional overinflation of alveoli in some regions may occur even if the UIP is not reached.
This variability in PEEP-mediated lung recruitment was demonstrated by Gattinoni et al. Patients with ALI/ARDS underwent CT scans after random application of different levels of PEEP (5, 15 cm H2O random)50 (Fig. 51-2). The percentage of potentially recruitable lung varied widely among these patients corresponding to an absolute weight of 217 ± 232 g of recruitable lung tissue.50 In addition to the observed variability in recruitable lung volume, PEEP may recruit new lung units and/or merely overdistend those already open. Therefore, a sophisticated and tailored ventilation strategy to limit the deleterious consequences of excessive PEEP is required. Grasso et al demonstrated that ARDS patients with CT-scan evidence of focal loss of aeration developed alveolar overdistension and release of inflammatory mediators when they were ventilated using the PEEP/FiO2 table from the ARDSNet study.51 The extent to which this influenced the failure of the trials of higher PEEP and lung recruitment in ARDS remains speculative.52 Low PEEP levels associated with low-tidal-volume ventilation have been recognized to be deleterious as well. In ARDS patients, a high percentage of potentially recruitable lung seems to be an independent risk factor for mortality. Therefore in this subgroup of patients the beneficial impact of reducing atelectasis by increasing PEEP prevails over the effects of increasing alveolar strain and overinflation.53
The relative importance of maintaining airway patency and using relatively high levels of PEEP is emphasized by several studies suggesting lung underdistension may be as injurious as lung overdistension and may contribute to the development of VILI. In animal studies, ventilation with zero PEEP or at levels of PEEP that did not produce adequate lung recruitment has been shown to cause respiratory and membranous bronchiolar injury, a reduction in lung compliance, and hyaline membrane formation.54,55 In theory, ventilation at low lung volumes causes repetitive opening and closure of alveoli. This in turn may lead to the development of shear stress along the bronchial and alveolar walls. Repetitive stress is known to disrupt surfactant and may disrupt epithelial structures contributing to stress failure of the alveolar-capillary barrier. Several recent in vivo and ex vivo animal studies have attempted to clarify the deleterious effects of atelectatic regions, which could be associated with insufficient levels of PEEP.56,57 The presence of atelectatic regions is associated with damage to distal airways of atelectatic and predominantly nonatelectatic alveoli as demonstrated by higher histological damage, myeloperoxidase protein expression, and localization of inflammatory cytokines mRNA expression.56 Moreover, in an isolated lung injury model it has been demonstrated that lungs ventilated with 6 mL/kg and low levels of PEEP had more ultrastructural evidence of cell damage possibly through mitogen-activated protein kinase (MAPK)–mediated pathway compared to those ventilated with higher PEEP levels.57 In addition to serving as a purely mechanical stent, PEEP may keep alveoli patent by preserving surfactant function, and in so doing may reduce surface tension and in turn reduce the tendency of alveoli to close.58,59
PEEP may also potentially improve gas exchange and lung mechanics by redistributing lung water from the alveolar to the extraalveolar interstitial space.60 Finally, PEEP has also significant hemodynamic effects and typically results in a reduction in ventricular preload and a reduction in cardiac output. Dreyfuss and Saumon postulated that the benefits of PEEP in ARDS stem from its effect on pulmonary perfusion, and demonstrated that the reduction of lung edema produced by PEEP was negated when dopamine was administered to keep arterial blood pressure constant.31
In summary, in patients with ARDS, PEEP may improve lung compliance and oxygenation by recruiting alveoli and maintaining patency throughout the respiratory cycle (Fig. 51-3). Furthermore, a ventilation strategy that fails to optimize end-expiratory volume with PEEP may contribute to VILI through the development of shear stress during repetitive opening and closing of lung units. It is also clear that atelectasis and inhomogeneity of alveolar patency can have adverse effects on lung and cardiac function. Consequently, the notion of best PEEP needs to be extended to include an EELV that will minimize lung injury and optimize cardiac performance. Finally as ARDS is not a static process, the optimum level of PEEP will vary over time, demanding that the level of PEEP be regularly reviewed in a given patient.
FIGURE 51-3
The sigmoidal shape of the pressure-volume curve of the respiratory system in a patient with ARDS. The outlines across the top of the graph indicate the relative state of inflation of alveoli. At airway pressures above the upper inflection point C (30 cm H2O), the curve flattens as the limits of lung compliance are reached, and there is progressive overdistention of alveoli. Airway pressures below the lower inflection point B are also associated with lower compliance and result in alveolar collapse. A typical ventilation strategy using 15 cm H2O of PEEP and a PIP of 40 cm H2
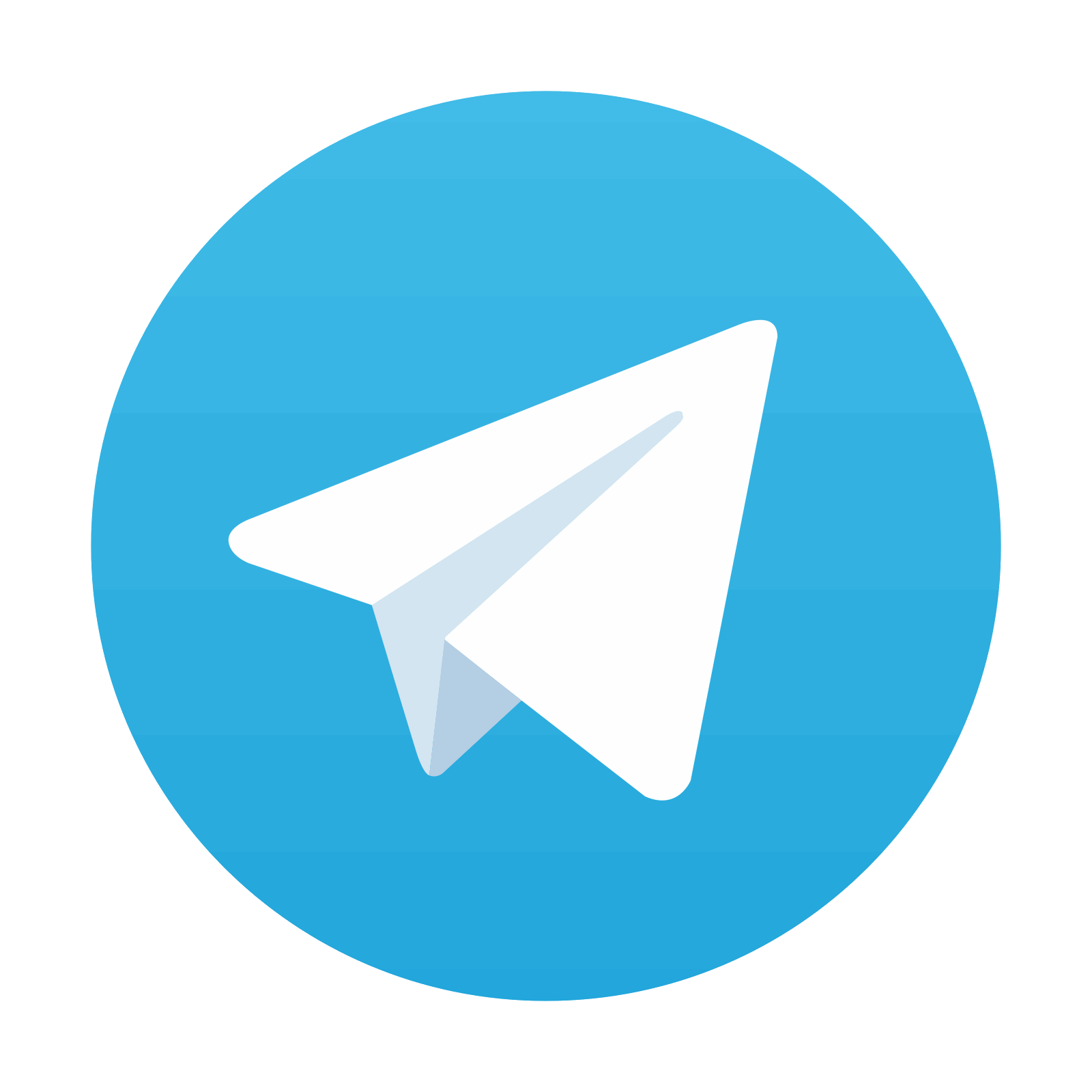
Stay updated, free articles. Join our Telegram channel

Full access? Get Clinical Tree
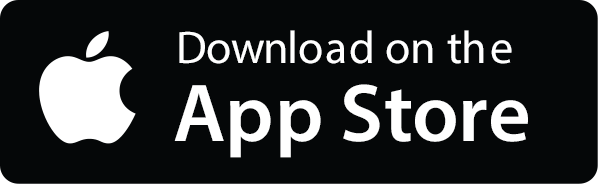
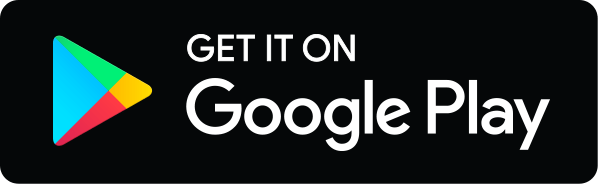