Abstract
The use of ultrasound (US) in the perioperative clinical management should be goal-directed, rapid, and reproducible. Thoracic US enables detailed examinations of vital structures, such as the airway, lung, pleural space, diaphragm, and heart. This chapter focuses on the value of US as a bedside tool to assess anatomy, guide procedures, and monitor vital organ function in patients undergoing thoracic surgery.
1
Introduction
Medical pioneers have long emphasized the vast potential of US-based imaging in anesthesiology to enhance the safety and efficacy of various invasive procedures and to noninvasively monitor vital organ function and anatomy [ ]. The mounting clinical evidence for the value of point-of-care US (POCUS) in the perioperative setting, its growing affordability, and its low associated risks have triggered worldwide movement in anesthesia aimed at increasing the focus on and use of perioperative US in daily clinical practice [ ]. Ideally, the use of POCUS in the clinical management of the patient should be goal-directed, rapid, and reproducible. Thoracic US is now an integral part of perioperative and critical care because it enables detailed examinations of vital structures, such as the airway, lung, pleural space, diaphragm, and heart.
This chapter focuses on the value of US as a bedside tool to assess anatomy, guide procedures, and monitor vital organ function in patients undergoing thoracic surgery. The three primary applications of US for this purpose are airway, lung US, and echocardiography [ ].
2
Airway US
Numerous studies and reports have described the role of US imaging in airway management. The use of upper airway US is operator-dependent and requires proper training to become proficient in its use. In the hands of the well trained anesthesiologist, it enables rapid assessment of airway anatomy and confirmation of correct endotracheal tube (ETT) placement.
2.1
A/airway assessment
2.1.1
Airway sono-anatomy
The following views can be used to assess the upper airway: suprahyoid, thyrohyoid, thyroid, cricothyroid, and suprasternal [ , ]. Depending on the indication for upper airway evaluation, fewer views may suffice to answer a focused question. Fig. 1 .

The hyoid view is the US image acquired through the hyoid bone and appears as a horse-shoe. The thyrohyoid view is located just below the hyoid bone and is used to visualize the epiglottis through the thyrohyoid membrane. The strap muscles, also known as the infrahyoid muscles, appear as 2 hypoechoic oval areas in the pre-epigottic area. The epiglottis appears as a thin hypoechoic stripe lying just anterior to a hyperechoic air-mucosa. The entire image appears as a “monkey face sign” where the strap muscles appear as the eyes and the epiglottis appears as the mouth [ , ].
The thyroid cartilage appears just below the strap muscles and forms a triangular shape. Sometimes, the vocal cords and arytenoid cartilages can be identified somewhat deeper to the thyroïd cartilage with central shadowing.
The thyroid cartilage will plunge from superficial to deep as it runs caudally until its border with the cricothyroid membrane. The cricoid cartilage will appear inferior to the cricothyroid membrane as a hypoechoic ovoid structure, and the tracheal rings will appear as smaller, evenly spaced intermittent hypodensities inferior to the cricoid cartilage. This appearance in sagittal view has been described as “beads on a string” [ ].
The suprasternal view is located just above the suprasternal notch and is the best location to both visualize ETT location and placement and to measure the tracheal diameter. On the transverse view, the trachea appears as a curvilinear convexity (“bullet sign”) with a hyperechoic air–mucosa interface and a posterior reverberation artifact. Reverberation artifacts occur when the US beam reflects back and forth between the two strong parallel reflectors (“reverberates”). The US transducer interprets the sound waves returning as deeper structures since it took longer for the US wave to return to the transducer, causing ‘repetitive line-artifacts’.
The esophagus is usually to the anatomical left of the trachea and appears as a bull’s eye on the left lateral posterior border of the trachea. Visualization of both trachea and esophagus at this level is important as the presence of a “double tract sign or g Oo se sign” indicates improper placement of the ETT into the esophagus during intubation [ , ].
2.1.2
US to predict difficult intubation
Predicting a difficult airway is crucial for saving lives but remains an area of research because the currently available clinical screening tests used to predict a difficult airway have only poor to moderate discriminative power when used alone. Combining individual tests or risk factors adds some incremental diagnostic value compared to the use of an individual test alone [ ]. Measuring the thicknesses of anterior neck soft tissue at the hyoid bone or thyrohyoid membrane—obtained transversely across the neck’s anterior surface using a linear array US probe—could serve as an additional predictor of difficult laryngoscopy [ , ]. Currently, there is still debate about the best parameter and the respective cut-off values [ ]. A measurement from skin to hyoid bone of more than 1,24 cm correlated with difficult laryngoscopy. At the thyrohyoid membrane level, a measurement between the skin and epiglottis of more than 2–2,5 cm could differentiate between difficult and easy laryngoscopy, respectively [ , ]. Fig. 2 .

Although these parameters seem promising as predictors of a difficult airway, more systematic studies and level-one evidence are needed before this modality can be recommended for routine screening.
2.1.3
US to predict airway size in children
Growing evidence supports the clinical use of US to estimate the appropriate ETT size, especially in children [ ]. In the pediatric population, a correctly sized ETT can help minimize the risk of intraoperative inadequate ventilation, postoperative stridor and subglottic stenosis because the narrowest part of the airway lies in the subglottic region at the level of the cricoid ring.
The upper airway’s subglottic diameter can be measured effectively by US. The outer diameter of the ETT correlated strongly with the subglottic tracheal diameter, which is measured just below the cricoid cartilage. The subglottic diameter is measured between the bilateral margins of the mid-cricoid cartilage. Importantly, this method outperforms age-based and height-based formulas in estimating the ETT size [ ]. Notably, while the ETT’s internal diameter remains invariant across manufacturers, its outer tube diameter varies. The outer diameter of the “best-fit” ETT is about 1 mm (0.1–1.7 mm) less than the subglottic diameter [ ]. Fig. 3 .

2.1.4
US to predict double-lumen tubes in adults
US is also useful to estimate the size of a double lumen tube (DLT) used to manage the airway in cases requiring one-lung ventilation. Inserting an inappropriate size can lead to the inability in complete lung isolation, severe injury to the airway, or the need to exchange the tube for a different size. A key step in avoiding complications associated with its use is choosing an appropriate-sized left DLT (LDLT). However, limited data exist on how to select the proper size of LDLT for the individual patient. Most anesthesiologists select LDLT based on patient height and gender. Various methods have been proposed to determine the proper LDLT size.
Although direct measurement of the tracheal diameter at the interclavicular plane from the preoperative conventional anterior-posterior chest radiograph may guide the prediction of correct LDLT placement [ ], measuring the tracheal diameter from a chest radiograph seems less reliable in patients of small stature [ ].
Therefore, a computerized tomography (CT) scan was proposed to directly measure the left main bronchus diameter, which constitutes an exact and accurate “gold-standard” method, it is not very practical, as experienced radiologists are needed [ , ].
Several researchers have found a direct correlation between tracheal diameter and left main-stem bronchial width measured by CT. If the diameter of the left bronchus cannot be measured directly, the tracheal diameter can be used to predict bronchial diameter [ ]. This is corroborated by the use of chest radiograph measurments to select the appropriate DLT size [ , ]. US also is an effective tool to measure tracheal diameter, is safe and is widely available in the operating room. US measurement of the trachea’s outer diameter is performed just above the sternoclavicular joint using a linear transducer and may guide DLT size determination [ ]. Fig. 4 .

The data contained in Table 1 can be used as a guide to estimate the left-sided DLT size [ ].
LDLT size (F) | TW US (mm) |
---|---|
41 | ⩾ 21,2 |
39 | ⩾ 19,3 |
37 | ⩾ 18,3 |
35 | ⩾ 17,4 |
32 | ⩾ 15,9 |
2.2
B/US to confirm endotracheal intubation
The use of US as an adjunct to capnography to confirm ETT placement has gained increasing popularity. A linear US probe is placed above the suprasternal notch in a transverse direction. At this point, caution should be exercised not to apply excessive pressure in order not to distort the airway anatomy. The tracheal rings are visualized as C-shaped hypoechoic (cartilage) and hyperechoic structures (the tissue–air border) with shadowing. The esophagus is usually seen on one side of the trachea as an oval structure with a hyperechoic wall and hypoechoic center (esophageal bull’s eye). Fig. 5 Tracheal US can be performed in real time as the ETT is being inserted, which can be observed as a “sliding” of the air-mucosal interface next to a single tracheal bullet sign (single air-mucosal interface with increased posterior artifact shadowing). Insufflation of the tracheal cuff with air causes a more lateral displacement of the tracheal walls and as a result a broader artifact shadowing at the level of the ETT cuff ( Fig. 5 ). After intubation, the presence of lung sliding confirms correct intubation and ventilation. An esophageal intubation reveals an adjacent hyperechoic structure with shadowing posterolateral to the trachea, consistent with the ETT location within the esophagus. This strategy has been referred to as the ““double tract sign or g Oo se sign” ( Fig. 6 ). Although, US enables rapid confirmation of tracheal or esophageal placement of ETT with a high sensitivity and specificity (respectively 0.98 and > 0.97) [ ], capnography still remains the standard to confirm endotracheal intubation.


3
Echocardiography in thoracic surgery
Perioperative echocardiography is considered an essential part of cardiac anesthesia, where it plays a crucial role in intraoperative decision-making and evaluation of surgical results. Outside the cardiac theater, however, the place of perioperative echocardiography is less well defined. According to relevant practice guidelines, echocardiography should be used when 1) the nature of the planned surgery or the patient’s cardiovascular pathology might result in severe hemodynamic, pulmonary, or neurologic compromise or 2) when unexplained life-threatening circulatory instability persists despite corrective therapy [ ].
Even though, such conditions are frequently encountered in thoracic anesthesia, practice still varies widely today. In fact, the decision to use echocardiography during noncardiac surgery depends on the individual anesthesiologist’s level of competence and experience with the technique. This indicates a need for structured echocardiography training programs if the potential benefits of this monitoring tool are to be extended beyond cardiac anesthesia practice [ ]. In a consensus paper from the American Society of Anesthesiologists and the Society of Cardiovascular Anesthesiologists, both emphasize the significant role of basic perioperative echocardiography and defined its role as an “intraoperative monitoring technique focusing on cardiac causes of hemodynamic or ventilatory instability, including ventricular size and function, valvular anatomy and function, volume status, pericardial abnormalities and complications from invasive procedures, as well as the clinical impact or etiology of pulmonary dysfunction” [ ]. The authors clearly delineate the knowledge and training requirements, as well as the indications and scope of a basic echocardiographic exam, which is distinct from the advanced (cardiac-diagnostic) level, but has value in its own right. POCUS incorporation into daily clinical care has decreased the “skill-gap” for utilizing US among anesthesiologists, but the transition toward standard of care will still need more time and training [ ].
In thoracic anesthesia, the unique ability of echocardiography to monitor right ventricular (RV) function is of particular interest. Lung surgery exposes patients to conditions associated with increased RV afterload, such as hypoxia, hypercapnia, one lung ventilation, and pulmonary artery clamping [ ]. As RV pump function is very sensitive to increases in afterload, RV dysfunction is a frequent cause of hemodynamic instability in this setting. A rapid differential diagnosis is important to enable specific and rapid treatment. Routine use of transesophageal echocardiography (TEE) in nontransplant thoracic surgery for this purpose is not recommended, but lung transplantation is a clear indication of its intraoperative use [ ].
Pneumonectomy is also associated with profound changes in RV volume and pressure load. For subjects with reduced cardiovascular reserve, pneumonectomy imposes a significant risk, and continuous intraoperative echocardiography could benefit such patients. Finally, patients with known or suspected pulmonary arterial hypertension are at an increased risk for perioperative RV failure. This subgroup should be monitored with echocardiography during and after any type of intermediate-risk surgery.
The quantification of RV function has become a topic of intense research. Although several new indices have been proposed, the majority are sensitive to error or require advanced interpretation [ ].
We recommend a pragmatic, fast, and rather simple approach that can easily be integrated in a basic echo exam and comprises only two consecutive measurements: 1) a comparison of left and right chamber dimensions and 2) assessment of RV function ( Fig. 7 .). This can be performed by measuring the long-axis displacement of the tricuspid annulus during systole in 2D using Tricuspid Annular Plane Systolic Excursion (TAPSE) or tissue Doppler imaging (TDI) [ ]. Both can be obtained in the standard 4-chamber view (mid-esophageal for TEE and apical for transthoracic echocardiography (TTE) and allow a semiquantitative appreciation of RV function, provided that the imaging plane is optimally aligned with the heart’s long axis (no foreshortening) and positioned midway the anteroposterior diameter.

Primary RV dysfunction is always associated with RV cavity enlargement. Because the pericardial space is restricted, RV dilation goes at the expense of LV size. The most reliable sign of RV enlargement is therefore an increase in the size ratio of the RV versus LV. Under normal conditions, the RV size is smaller than the LV size, and its dimensions never exceed 2/3 of the corresponding LV dimension. The semiquantitative appreciation of relative proportions is simple and shows high interobserver agreement. For a quantitative assessment, the short axis diameter of the RV is measured as the distance from the RV free wall to the RV septal site and compared to the short axis diameter of the LV, i.e., the distance from the LV free wall to the LV septal site at a corresponding level midway the apex and basis of the heart on a line perpendicular to the long axis.
The second measurement of RV function is based on the fact that RV systolic deformation occurs primarily along its long axis, producing a piston-like displacement of the tricuspid annulus toward a nearly immobile apex. The absolute distance traveled by the tricuspid annulus during systolic ejection is termed “TAPSE” and is considered a very reliable, though load sensitive, measurement of RV ejection. Normal TAPSE should exceed 16 mm, and lower values apply to mechanically ventilated patients. A decrease below 16 mm when combined with an increase in RV/LV size ratio above 2/3 indicates a reduction in RV–arterial coupling—a functional decline in RV performance. During surgery, this is almost invariably associated with an acute increase in the RV afterload. Next to TAPSE, TDI can be applied to measure the peak systolic velocity of the tricuspid annulus. A value above 9.5 cm/s indicates preserved RV function. Both TAPSE and TDI-S’ myocardial velocities reflect the longitudinal systolic function of the RV.
Apart from monitoring RV function, other specific advantages of echocardiography during noncardiac thoracic surgery include the ability to detect lesions that can predict adverse outcomes (aortic atheromata), to provide guidance in the placement of extracorporeal membrane oxygenation cannulas [ ] and for hemodynamic management in ECMO patients, and to diagnose patent foramen ovale in unexplained hypoxia. The use of echocardiography for such purposes requires significant experience, but TEE and TTE are considered feasible for this purpose [ ].
In the postoperative setting, basic echocardiography offers the same value as discussed in the intraoperative scenario. The ability to use the transthoracic modality lowers the threshold for using it in the post-anesthesia and intensive care units even more. The information obtained is noncontinuous, and its value is determined primarily by user proficiency.
4
Perioperative lung US
Lung US is the use of US to examine the chest, based on real-time dynamic 2D imaging and artifacts from the interplay between ultrasonic waves and air, lung tissue, pleura, fluids, and bone. It has gained much attention in recent years for its value in clinical perioperative practice for screening, diagnosis, and as a monitoring tool [ ]. Lung US is noninvasive, readily available in most operating rooms and intensive care settings and has been shown to reduce the need for X-rays [ , ].
4.1
Equipment
Although a micro-convex probe with an emission frequency of 5–7 MHz is preferred for optimal US visualization of the lung, virtually all probe types can be used. As lung US relies on the visualization of natural images with many artifacts, all filters on the echo device, such as harmonics or dynamic noise filters designed to attenuate artifacts and optimize image quality, should be inactivated. Most vendors have created default settings for lung US, which turn off these filters automatically.
4.2
Recommended scanning regions
The examination should be performed in a standardized and comprehensive way, with the patient in a supine or semi-recumbent position. Scanning the anterior, anterolateral, and posterior zones of the lung is recommended [ ]. The anterior points are referred to as upper BLUE, the anterolateral points as lower BLUE, and the posterior points as the “posterolateral alveolar and/or pleural syndrome point,” or PLAPS point. The number of scan points can be extended as needed in order to identify the exact location of the lung point in the pneumothorax ( Fig. 8 ).

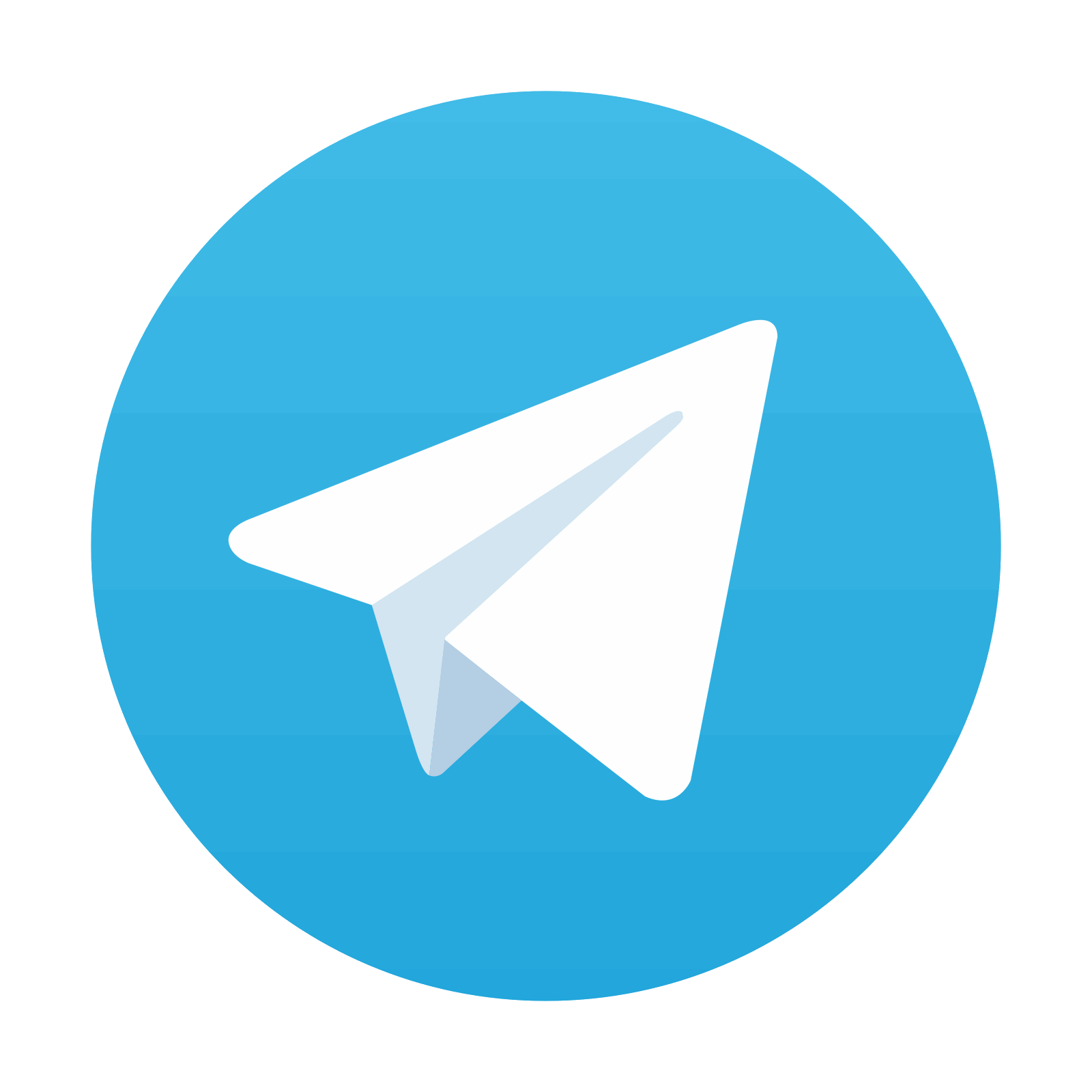
Stay updated, free articles. Join our Telegram channel

Full access? Get Clinical Tree
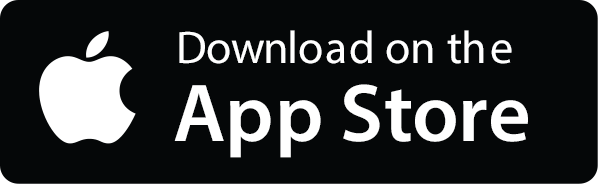
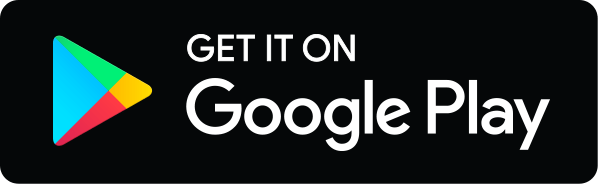
