# Cesarean delivery
Probability (%) of accreta
1
3
2
>10
3
40
4 or above
>60
Imaging tests can aid in the diagnosis of placenta accreta. Ultrasound has both a high specificity and sensitivity for detecting abnormal placentation, although studies have yielded conflicting results. Sonographic findings in the second and third trimesters suggestive of accretism include irregular vascular spaces within the placenta, the loss of a hypoechoic retroplacental zone, and vascular invasion into the uterine wall, as detected with three-dimensional Doppler studies. MRI may help to confirm or exclude the diagnosis, as well as provide additional information about posteriorly located placentas, the depth of invasion, and the extent of adjacent organ involvement [39]. Cystoscopy and sigmoidoscopy are not routinely recommended, but may help occasionally in the evaluation of select patients. Of note, cystoscopy with or without bladder biopsy in patients with placenta percreta may result in uncontrollable hemorrhage. Unfortunately, maternal serum alpha-fetoprotein, maternal serum creatinine kinase, and other laboratory markers have not been evaluated sufficiently to become a routine component of the workup of placenta accreta.
Patients with known or suspected placenta accreta should be scheduled for delivery at an institution with adequate facilities, such as interventional radiology (IR) suites, ample blood bank supplies, and available ancillary personnel, including urologic, gynecologic-oncologic, general, and vascular surgeons. At some such centers, interventional radiologists routinely place prophylactic balloon devices in the common iliac arteries or selectively embolize collateral pelvic vessels in all patients with abnormally adherent placentas [40]. However, the evidence in support of prophylactic endovascular procedures is lacking, and several complications, such as nerve injury, hematoma, ineffective balloon deployment, leg ischemia, and iliac artery rupture during balloon inflation, have been reported [38]. Another management option involves scheduled cesarean delivery in the IR or angiography suites at institutions with appropriate capabilities. Advantages of this strategy are readily available, high-quality imaging equipment, minimal risk of migration of intra-arterial catheters during patient transport, and the ability to perform cesarean delivery emergently if complications arise during the endovascular procedure [41]. Still other institutions perform embolization of persistent bleeding vessels after cesarean delivery in patients at high risk for hemorrhage, transporting stable patients to the IR suite postoperatively.
Early mobilization of blood bank resources and several other perioperative measures may help minimize the morbidity and mortality associated with placenta accreta. Although blood product requirements are difficult to predict, arrangements should be made in advance to ensure that platelets, fresh frozen plasma (FFP), packed red blood cells (PRBCs), and cryoprecipitate are immediately available. Because blood loss can be life-threatening, many institutions have implemented massive transfusion protocols on the labor and delivery unit to facilitate access to blood products. The so-called damage control resuscitation strategy, which emerged from relatively recent experience at military and civilian trauma centers, calls for a 1:1:1 ratio of PRBCs, FFP, and platelets [42]. Early administration of cryoprecipitate and the timely administration of recombinant Factor VIIa and/or anti-fibrinolytic agents for ongoing massive obstetric hemorrhage should be considered. Other related considerations include placement of an arterial line for both improved blood pressure monitoring and frequent blood draws, the establishment of additional large-bore venous access (central or peripheral), availability of high-flow infusion devices for rapid administration of intravenous fluids and blood products, and avoiding maternal hypothermia.
Additional perioperative planning may include ureteric stent placement to facilitate palpation of the ureters and identify intraoperative ureteral injury. This procedure has not been proven to reduce the incidence of ureteral complications, but is occasionally performed preoperatively when the accreta involves the lower uterine segment or intraoperatively if complications arise. When bladder involvement is suspected intraoperatively, cystoscopy can help delineate the extent of invasion and identify treatment options. Dissection of the bladder from the uterus can precipitate life-threatening hemorrhage; partial cystectomy is recommended in the setting of extensive bladder invasion. Additional management options for placenta accreta include intrauterine balloon tamponade, complete or subtotal hysterectomy, intraoperative bilateral hypogastric arterial ligation, and, rarely, leaving the placenta and uterus in situ, followed by methotrexate therapy to expedite absorption of placental tissue [43].
Anesthetic management of parturients with placenta accreta should be individualized. In general, neuraxial techniques are associated with a decreased incidence of failed intubation, intraoperative recall, aspiration of gastric contents, and maternal mortality compared to general anesthesia [44]. However, anticipated uncontrollable hemorrhage, coagulopathy, or maternal refusal may prohibit regional techniques. When maternal hemodynamic instability or lengthy surgical interventions are expected, a single-shot spinal anesthetic may not be appropriate. Further, practitioners may prefer to secure a known or anticipated difficult airway at the beginning of a potentially complicated surgical procedure. That said, continuous catheter techniques (e.g., epidurals, combined spinal-epidurals, and spinal catheters) are considered safe and appropriate for the management of patients with placenta accreta [45]. In particular, neuraxial catheters are suitable for cases involving perioperative endovascular intervention and cesarean delivery, as the catheter can be dosed to provide adequate anesthesia for both procedures and the risk of life-threatening hemorrhage with in situ balloon occlusion devices or after selective arterial embolization is presumably decreased. Alternatively, regional anesthesia can be used for the IR procedure and for delivery of the newborn, with planned conversion to general anesthesia for the remainder of the procedure. Of note, once the femoral sheaths are in place, the patient can no longer position herself for an epidural placement due to the risk of dislodgement; if a continuous catheter technique is planned for the cesarean delivery, it must be placed prior to the IR procedure. As in all cases performed under regional anesthesia, practitioners must be prepared to convert to general anesthesia when circumstances dictate.
Trauma During Delivery
Although uncommon, the bladder, ureters, and urethra are vulnerable to trauma during vaginal deliveries, including those with forceps and vacuum assistance, and cesarean sections. Periurethral lacerations, urethral detachment, and damage to the urethral sphincter during labor and delivery are among the lower urinary tract injuries that can contribute to postpartum stress incontinence. The bladder is also vulnerable to injury during vaginal delivery, particularly in the vicinity of the trigone. The incidence of bladder injury during cesarean delivery is estimated at 0.28%, but is higher in the setting of prior cesarean deliveries [46], cesarean hysterectomy, emergency cesarean deliveries, previous abdominal surgeries, and extensive abdominal scarring [47]. The area most commonly at risk during operative deliveries is the dome, but injury may extend into the trigone. Diagnosis is usually immediate, but can be facilitated with the intravenous administration of indigo carmine or methylene blue or via retrograde bladder filling with sterile milk or dye. Injury of the ureters, however, is more difficult to detect and may present in the postpartum period with unilateral hydronephrosis and flank pain. The diagnosis can be confirmed postpartum with an IVP. In cases where there is a high index of suspicion for ureteral injury intraoperatively, cystoscopy is performed to ensure the integrity of the urinary tract. Repair of this rare complication, which occurs at an estimated rate of between 0.02–0.1%, may require ureteric reimplantation or stenting [48].
Maternal Safety
In general, optimizing care of pregnant patients receiving either general or regional anesthesia ensures optimal care of the fetus, regardless of gestational age, type or location of surgery, and underlying maternal coexisting disease. Yet pregnancy produces profound physiologic and anatomic changes due to hormonal alterations, physical adaptations to accommodate the developing fetus, and increasing metabolic demands. Potential far-reaching anesthetic implications of these changes require familiarity with the physiology of normal pregnancy.
Respiratory Changes
Alterations in respiratory function during pregnancy can be attributed to anatomic and hormonal changes, as well as to changing metabolic demands. With upward uterine displacement of the diaphragm, beginning in mid-gestation, expiratory reserve volume (ERV) and residual volume (RV) fall by 25% and 15%, respectively. As a result, functional residual capacity (FRC) drops markedly after 5 months’ gestation, reaching 80% of prepregnancy values by term. Supine positioning, obesity, and the induction of general anesthesia exacerbate this decrease. Tidal volume (TV) increases by 45% early in pregnancy, yet vital capacity (VC) remains unchanged due to the increase in inspiratory reserve volume (IRV). Total lung capacity shows little to no change (see Table 11.2).
Table 11.2
Respiratory physiology in term patients versus nonpregnant patients
Lung volumes | Percentage change |
IRV | ↑5 |
TV | ↑45 |
ERV | ↓25 |
RV | ↓15 |
Lung capacities | Percentage change |
IC | ↑15 |
FRC | ↓20 |
VC | 0 |
TLC | ↓5 |
Ventilation | Percentage change |
MV | ↑45 |
AV | ↑45 |
RR | 0 |
Respiratory mechanics | Percentage change |
FEV1 | 0 |
FEV1/FVC | 0 |
Flow volume loop | 0 |
There are several clinical implications of the changes in respiratory mechanics during pregnancy. Minute ventilation increases primarily as a result of increased TV and in part due to the increased respiratory rate. The arterial pressure of carbon dioxide (PaCO2) decreases, reaching 30 mmHg early in pregnancy. Partially compensating for this respiratory alkalosis, the kidneys excrete bicarbonate, which decreases to 20 mEq/L throughout pregnancy. Overall, the pH remains at roughly 7.44, slightly more alkalotic than the normal state. The arterial pressure of oxygen increases only slightly during pregnancy, ranging from 107 mmHg in the first trimester to 103 mmHg in the third trimester (see Table 11.3). The combination of a decreased FRC and an increased oxygen consumption results in rapid oxygen desaturation during periods of apnea, as during anesthetic induction. Finally, the increased minute ventilation and decreased FRC result in a faster uptake of volatile anesthetics during pregnancy. This, along with the increased levels of plasma endorphins and progesterone, contributes to a faster rate of anesthetic induction, as well as to a reduction in minimal alveolar concentration to up to 40% nonpregnant levels.
Table 11.3
Blood gases during pregnancy
Nonpregnant | Trimester | |||
---|---|---|---|---|
First | Second | Third | ||
pH | 7.40 | 7.41–7.44 | 7.41–7.44 | 7.41–7.44 |
Po2 mmHg | 100 | 107 | 105 | 103 |
Pco2 mmHg | 40 | 30–32 | 30–32 | 30–32 |
[HCO3 −] mEq/L | 24 | 21 | 20 | 20 |
The airway also changes during pregnancy and over the course of labor. Swelling of the oropharyngeal tissue, a decrease in the size of the glottic aperture, and mucosal friability are present from mid-gestation until several days postpartum, but are most pronounced near the end of pregnancy. A deterioration in airway classification, as manifested by an increased incidence of Mallampati classes 3 and 4 and a significant decrease in oropharyngeal area and volume, continues throughout labor and appears to be unrelated to both the duration of labor and the amount of fluid administered [49]. The presence of comorbidities, such as obesity or preeclampsia, can magnify these changes. Access to video laryngoscopes and other airway adjuvants, optimization of maternal positioning, familiarity with the emergency airway algorithm for obstetric patients, and increased use of regional anesthesia, when appropriate, in patients with known or suspected difficult airways may help minimize the complications associated with difficult ventilation and intubation.
Cardiovascular System
Pregnancy is associated with several cardiovascular adaptations. Myocardial contractility remains unchanged, but systemic vascular resistance (SVR) decreases due to the low-resistance placenta and the potent vasodilating effects of progesterone and prostacyclin. This reduction in SVR contributes to an initial increase in cardiac output (CO) beginning as early as 5 weeks’ gestation. CO continues to increase gradually throughout pregnancy, peaking at 28–32 weeks’ gestation and, again, during the second stage of labor. The highest CO, however, is in the immediate postpartum period. Both an increased stroke volume (SV) and heart rate (HR) contribute to the increased CO, but the former contributes to a greater degree (see Table 11.4).
Table 11.4
Hemodynamic changes during pregnancy compared to nonpregnant women
Parameter change | Percentage |
---|---|
CO | ↑50 |
SV | ↑25 |
HR | ↑15 |
SVR | ↑20 |
Maternal positioning influences the pregnancy-induced increase in CO. Sharp reductions in CO are seen in some women in the supine position as early as 18–20 weeks’ gestation, when the enlarging uterus begins to compress the inferior vena cava and, less often, the aorta. At term, aortocaval compression in the supine position (a.k.a. supine hypotensive syndrome) can reduce the CO by 30%. General and neuraxial anesthesia impair sympathetic tone and compromise the normal physiologic response to aortocaval compression, exacerbating maternal hypotension and further reducing cardiac preload and output. LUD with either a wedge placed under the patient’s right side or a 15–20° tilt of the operating table should be maintained during all procedures involving parturients after mid-gestation. Assuming the lateral recumbent position or a knee chest position can also help to maintain CO during procedures, such as spinal or epidural placement.
Cardiovascular changes of pregnancy can be attributed in part to several hematologic changes. Plasma volume expands gradually throughout pregnancy, starting as early as 6 weeks’ gestation and peaking at 30–34 weeks. The red blood cell mass increases also, beginning at 8–10 weeks’ gestation, but a disproportionate increase in plasma volume contributes to a relative anemia of pregnancy. Despite this increased erythropoiesis, hemoglobin, hematocrit, and blood viscosity decrease during pregnancy (see Table 11.5).
Table 11.5
Changes in blood volume and its consequences during pregnancy
Parameter | Percentage change or actual value |
---|---|
Blood volume | ↑45 |
Plasma volume | ↑55 |
RBC volume | ↑30 |
Hemoglobin | 11 |
Hematocrit | 35 |
Urinary System
Kidney, ureteral, and bladder changes accompany different stages of pregnancy. The kidneys elongate by 1–1.5 cm, while kidney volume increases up to 30%. The renal pelvises, calyces, and ureters begin to dilate as early as 7 weeks’ gestation due to both the vasodilating effects of progesterone and the mechanical compression of the ureters at the pelvic brim. Of note, a protective effect of the sigmoid colon and dextrorotation of the uterus contribute to more marked ureteral dilatation on the right [50]. Hormonal changes also affect the bladder and urethral mucosa, which becomes more hyperemic and congested. The bladder itself develops an increased capacity and becomes displaced upwards and anteriorly by the gravid uterus; by the third trimester, it protrudes markedly into the abdomen and undergoes additional anatomic distortions.
Glomerular filtration rate (GFR) increases up to 50% by the beginning of the second trimester. This physiologic increase in GFR, which tapers towards term, leads to a decrease in serum creatinine and blood urea nitrogen values and an increase in protein, amino acid, water-soluble vitamin, and glucose excretion. Additional changes in kidney function during pregnancy include a decrease in the renal bicarbonate threshold and a related decrease in serum bicarbonate as well as osmoregulation alterations, manifested by decreased serum osmolality.
Gastrointestinal System
Multiple changes in the gastrointestinal system may predispose pregnant women to aspiration, although it remains unclear when this risk becomes significant. Lower esophageal sphincter (LES) tone is impaired early in pregnancy as a direct result of progesterone, predisposing gravid women to gastroesophageal reflux disease (GERD). Gastric and pyloric anatomic changes related to the enlarging uterus occur later, increasing the risk of both GERD and, possibly, aspiration pneumonitis after roughly 18–20 weeks’ gestation.
Recent studies have cast doubt on the traditional dogma that pregnant women have decreased gastric motility, increased gastric acidity, and increased gastric volume, prompting a more liberal approach to full stomach precautions in pregnancy [51]. Although GERD is common in pregnant patients, gastric emptying does not appear to be delayed during pregnancy until the onset of labor and/or the administration of systemic or neuraxial opioids [52], nor does there appear to be a difference in the acidity of gastric contents in pregnant and nonpregnant patients [53]. Consequently, in the absence of GERD, motility disorders, or other common risk factors for aspiration, it may be appropriate to reserve full stomach precautions for patients after 20 weeks’ gestation.
Fetal Safety
In pregnant women undergoing nonobstetric surgical procedures, health-care providers must take into consideration both maternal and fetal well-being. In general, optimizing maternal care optimizes fetal well-being. Yet despite careful attention to maternal well-being, women exposed to surgery and anesthesia during pregnancy have a higher incidence of fetal loss, preterm labor, growth restriction, and low birth weight infants, all of which are most likely attributable to the underlying maternal condition requiring surgery [54]. This section explores issues that affect fetal outcomes during nonobstetric surgery, with emphasis on mechanisms to minimize the risk of preterm labor and optimize uteroplacental perfusion. Although there does not appear to be an increased risk of congenital anomalies in women who have nonobstetric surgery during pregnancy, this section opens with a review of common concerns about teratogenicity of anesthetic agents.
Teratogenicity
Teratogenesis manifests as structural or functional abnormalities, such as growth restriction, malformations, and fetal death, arising from dysgenesis of fetal organs. A limited number of agents are known teratogens in human beings (see Tables 11.6 and 11.7). To date, no commonly used anesthetic agent or adjuvant has been proven to be teratogenic in clinically relevant doses. However, studies of human teratogenicity are limited by ethical concerns, and newly marketed drugs are rarely, if ever, tested in pregnant patients [55]. As a result, most information currently available is gleaned from animal research and epidemiologic studies, and the majority of anesthetic drugs remain classified as Category C (see Table 11.8).
Table 11.6
Known teratogenic agents in human beings
Captopril | Methimazole |
Carbamazepine | Phenobarbital |
Cocaine | 1,3-cis-Retinoic acid |
Enalapril | Tetracyclines |
Fluconazole, high doses | Thalidomide |
Glucocorticoids | Valproic acid |
Lithium |
Table 11.7
Possible and unlikely teratogens in humans
Possible teratogens | Unlikely teratogens |
---|---|
Azathioprine | |
Colchicine | |
Disulfiram | Anesthetics |
Ergotamine | Aspirin |
Paroxetine | Illicit drugs (marijuana, LSD) |
Pseudoepinephrine | Metronidazole |
Streptomycin | Oral contraceptives |
Zidovudine (AZT) |
Table 11.8
Pregnancy categories of common anesthetic drugs and their adjuvants
Atropine sulfate: Category C | Morphine: Category C |
Dexamethasone (Decadron): Category C | Nalbuphine (Nubain): Category B |
Diphenhydramine (Benadryl): Category B | Naloxone (Narcan): Category C |
Dilaudid: Category C | Neostigmine bromide: Category C |
Ephedrine: Category C | Ondansetron (Zofran): Category B |
Esmolol (Brevibloc): Category C | Pepcid (Famotidine): Category B |
Fentanyl: Category C | Phenylephrine: Category C |
Glycopyrrolate (Robinul): Category B | Rocuronium (Zemuron): Category C |
Labetalol: Category C | Succinylcholine (Anectine): Category C |
Metoclopramide: Category B | Toradol (Ketorolac): Category C |
Methadone: Category C | Zantac (Ranitidine): Category B |
Midazolam: Category D | |
Category A: Controlled studies show no risk. Controlled studies show no risk (examples include levothyroxine, prenatal vitamins, potassium supplementation) | |
Category B: No evidence of risk in humans. Animal studies have revealed no evidence of impaired fertility or harm to fetus OR Animal studies have shown an adverse effect, but adequate and well-controlled studies in pregnant women have failed to demonstrate a risk to the fetus (examples include penicillins, macrolides, and most cephalosporins) | |
Category C: Risk cannot be ruled out. Animal studies have shown a risk, but there are no adequate, well-controlled studies in pregnant women OR No animal studies have been conducted and there are no adequate, well-controlled studies in humans (examples include albuterol, B-blockers, calcium-channel blockers, zidovudine) | |
Category D: Positive evidence of risk. Studies have demonstrated that this medication can cause fetal harm when administered to a pregnant woman. However, potential benefits of therapy may outweigh the potential risk (examples include lithium, valproic acid, carbamazepine, phenytoin, azathioprine, and systemic corticosteroids) | |
Class X: Contraindicated in pregnancy. Studies in animals or pregnant women have demonstrated positive evidence of fetal abnormalities or risks |
For a functional or structural abnormality to develop as a result of exposure to a teratogenic agent, the embryo or fetus must be genetically susceptible to the adverse effects and be exposed to a sufficiently large dose during a particular gestational period. Historically, diazepam and nitrous oxide have raised the greatest concerns among the commonly used anesthetic agents about risks of teratogenicity. Diazepam use during pregnancy has been associated with cleft lip in the newborn, generating a great deal of research over the past several decades. The evidence to date does not support this association [56]. Rather, judicious use of preoperative anxiolysis may serve to reduce circulating catecholamines, which compromise uteroplacental perfusion [57]. Nitrous oxide is known to inactivate methionine synthase, thereby inhibiting thymidine and DNA synthesis, and has been shown to be weakly teratogenic to rodents after prolonged administration [58]. However, the coadministration of volatile agents with nitrous oxide counters the teratogenic effects of nitrous on rodents without interfering in the vitamin B12-dependent enzymes, casting doubt on the mechanism of the teratogenicity of nitrous oxide [59]. Further, the inactivation of methionine synthase in rodents is far more rapid than in humans, and it is unlikely that findings from rodent studies can be extrapolated to humans [60]. Concerns about an increased incidence of spontaneous abortions among operating room personnel with chronic trace exposure to nitrous oxide also appear to be unfounded [61].
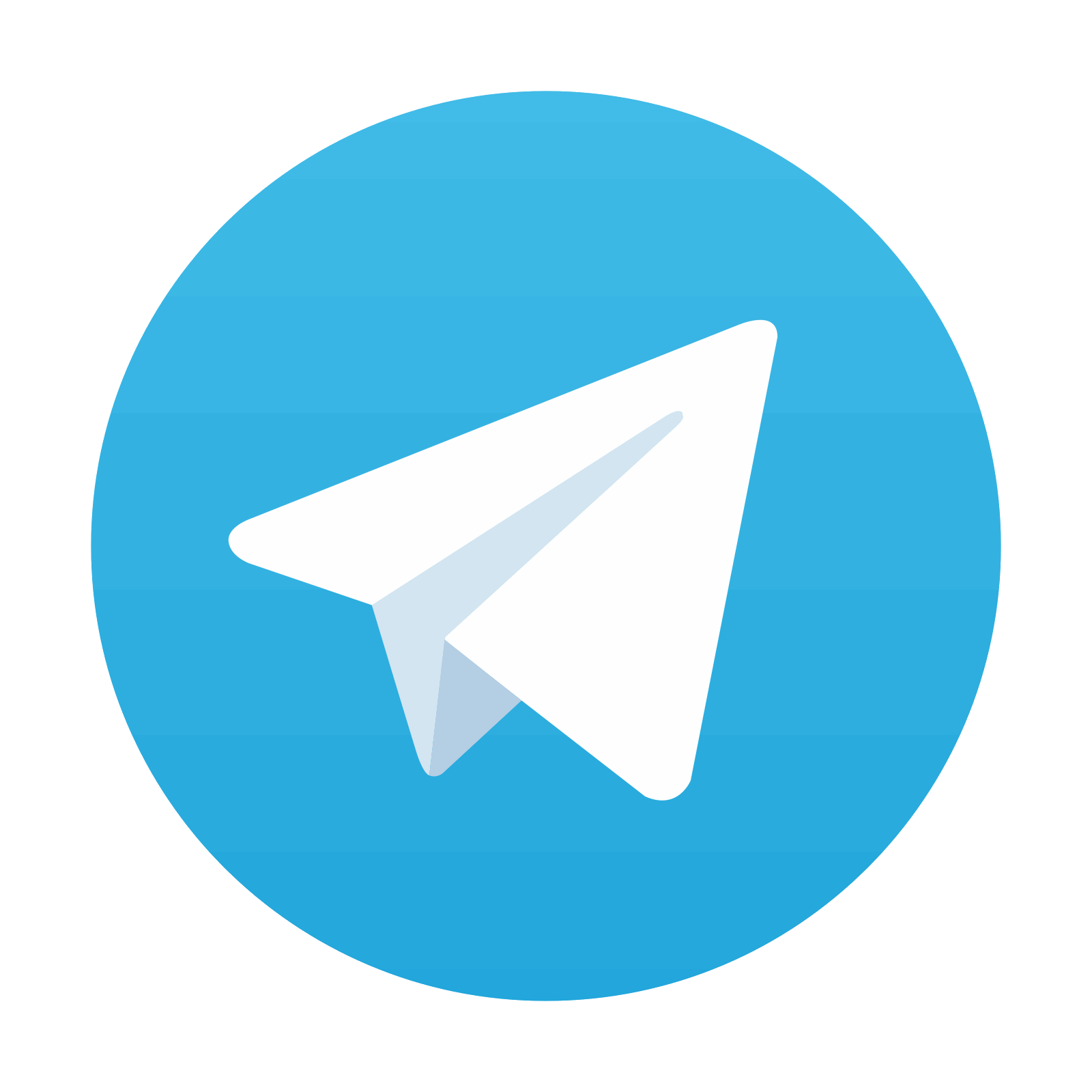
Stay updated, free articles. Join our Telegram channel

Full access? Get Clinical Tree
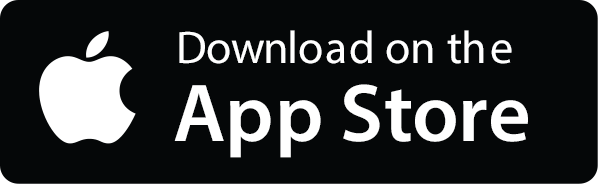
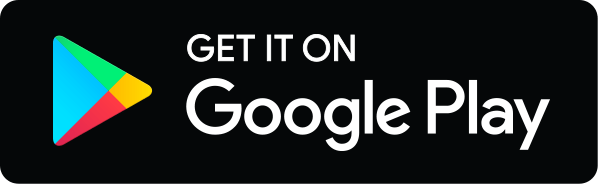