Key Clinical Questions
What useful clinical information can urinalysis provide?
What is the diagnostic significance of different urinary casts?
What are the appropriate clinical settings in which to measure the fractional excretion of sodium and the fractional excretion of urea?
What are the limitations of the different urinary tests?
How can the different pieces of information provided by various urinary tests be integrated to assist in making an accurate diagnosis?
Introduction
Urine tests can be a valuable diagnostic tool, especially since they are easily obtained and can provide a wide range of information. When interpreted in the context of a thorough history and physical examination, the clinician can use the information obtained from urinalysis to narrow the differential diagnosis and guide treatment. For example, in a patient presenting with kidney disease, the presence of significant proteinuria and red blood cell casts in the urine strongly suggests glomerulonephritis as a diagnosis. Unlike most blood tests, basic urinalysis can be performed rapidly by the examining physician.
This chapter will review how important clinical information can be obtained from various attributes of the urine—such as the appearance of the urine, urine specific gravity and osmolality, urine electrolytes, and urine microscopy. Especially when various urine tests are considered together, these tests can shed significant light on the patient’s clinical status. However, all urine tests have significant limitations. Moreover, certain urine tests, such as urine osmolality, have wide reference ranges, so a urine osmolality value that may be normal in one patient could be abnormal in another patient. For example, a relatively low urine osmolality of 100 mOsmol/kg may be normal in a patient who takes in a large volume of water, but could suggest diabetes insipidus in a patient who is hypernatremic with polyruia.
Urine Color and Appearance
Even before any official urinalysis results are available, inspection of the urine can be revealing (see Table 104-1). For instance, urine may be pale yellow or clear in a patient who is well hydrated, whereas dark yellow urine can suggest a patient who is volume depleted. Urine may have a reddish hue in cases of significant hematuria, though a vaginal source or other perineal source needs to be considered. Myoglobinuria and hemoglobinuria, from rhabdomyolysis and hemolysis, respectively, can also cause red urine. Red urine can also result from the ingestion of certain foods, such as beets and rhubarb, and medications, such as senna and doxorubicin. Centrifugation of the urine can help distinguish hematuria from myoglobinuria and hemoglobinuria. Upon urine centrifugation, with hematuria the intact red blood cells (RBCs) should form a red-colored sediment only, whereas if the supernatant is red, that suggests the presence of myoglobinuria or hemoglobinuria. Foamy urine may be seen in patients with significant proteinuria.
Appearance of Urine | Associated Medications | Associated Conditions |
---|---|---|
Pale yellow | Volume replete | |
Dark yellow |
| |
Orange |
| |
Red |
|
|
Brown |
| Povidone-iodine contamination |
Blue-green |
| Pseudomonas aeruginosa urinary tract infection |
Milky | Propofol (depending on pH) | Lipiduria Chyluria |
Foamy | Proteinuria |
Medications can account for other color changes in urine, including milky urine with propofol; dark brown urine with metronidazole (or povidone-iodine contamination); orange with rifampin; and blue-green with indomethacin, amitriptyline, triamterene, and propofol (depending on pH). Patients who are jaundiced can have dark yellow or brown urine, and patients with porphyria can have urine that is red or brown. The odor of the urine can also be diagnostically useful, with a foul-smelling urine suggesting infection and sweet-smelling urine raising the possibility of ketonuria.
Urinalysis and Urine Dipstick Testing
Urinalysis provides information about a number of different properties of the urine. Two related properties of the urine are urine osmolality and urine specific gravity. Clinical settings in which measuring urine osmolality can be useful include diagnosing primary polydipsia as the cause of hyponatremia and evaluating a patient with polyuria. Urine osmolality is the concentration of particles per kilogram of solution. The usual physiologic range of urine osmolality is 50 to 1200 mOsmol/kg. Specific gravity is the density of the urine relative to the density of water. Unlike osmolality, which measures the solute concentration and is not a function of the size of the particles in solution, specific gravity is affected by the mass of the particles in the urine. Therefore, large particles that are excreted in the urine, such as radiocontrast material, can cause an increase in the specific gravity. Nonetheless, under most conditions, urine specific gravity usually correlates fairly well with osmolality. Urine specific gravities of 1.001, 1.010, and 1.030 are approximately equal to a urine osmolality of 40, 320, and 1200 mOsmol/Kg, respectively. In addition to the presence of radiocontrast in the urine, glucose and protein in the urine can also elevate the specific gravity. Urine osmolality is determined by a laboratory, whereas specific gravity is usually determined using a urine reagent strip (urine “dipstick”).
Clinically, urine osmolality—and so also urine specific gravity—will be elevated in cases in which antidiuretic hormone (ADH) is elevated. ADH can be elevated appropriately in cases of hypovolemia, or inappropriately, as in cases of the syndrome of inappropriate antidiuretic hormone secretion (SIADH). Of note, in SIADH, the urine osmolality may not be absolutely high, but it is inappropriately high relatively speaking, given that the adaptive response to hyponatremia is to excrete free water. A urine osmolality > 100 mOsm/kg is inappropriate in SIADH, although the urine osmolality frequently will be higher than this, typically higher than the serum osmolality. A urine osmolality <100 mOsm/kg in a hyponatremic patient suggests primary polydipsia. Patients with acute kidney injury (AKI) from acute tubular necrosis (ATN) will usually have a urine osmolality <400 mOsm/kg due to compromise of the kidney’s urinary concentrating ability. In contrast, patients with prerenal azotemia will usually have a urine osmolality that is >500 mOsm/kg. In cases of polyuria, the urine osmolality can help distinguish a water diuresis from a solute diuresis. A urine osmolality >300 mOsm/kg is consistent with a solute diuresis, whereas a urine osmolality <150 mOsm/kg is suggestive of a water diuresis. In cases of a higher urine osmolality (>300 mOsm/kg) suggestive of a solute diuresis, possible causes include high salt intake, glucosuria, or mannitol. In cases of low urine osmolality (<150 mOsm/kg) suggesting a water diuresis, possible causes include diabetes insipidus or excessive water intake, as with primary polydipsia.
The initial test commonly used to evaluate for hematuria is the urine dipstick. Urine dipsticks have sensitivities of 91% to 100%, using 2 to > 5 RBCs per high power field (HPF) on sediment microscopy as the reference standard. Urine dipsticks have specificities in the 65% to 99% range, using microscopy as the reference standard. There are a number of conditions that can cause false positive results for hematuria on urine dipsticks. Myoglobinuria and hemoglobinuria, from rhabdomyolysis and hemolysis, respectively, cause urine dipsticks to read positive for heme. The absence of RBCs on urine microscopy in the setting of a urine dipstick positive for heme suggests the possibility of rhabdomyolysis or hemolysis. In one series by Grover et al examining patients with rhabdomyolysis, a urine dipstick showing moderate or large urine heme in the absence of hematuria had a sensitivity of 81% for detecting creatine kinase levels greater than 10,000 U/l. As noted above, centrifugation of the urine can be useful in this setting, because with myoglobinuria and hemoglobinuria the supernatant only should be red, whereas in true hematuria the sediment should consist of a pellet of RBCs. Another condition that can lead to false positives for heme on urine dipsticks includes some bacteria with pseudoperoxidase activity, such as certain species of staphylococci and streptococci. Ascorbic acid can result in false negative results for hematuria (and glucosuria).
Urine microscopy is important because a heme-positive dipstick result can occur in the absence of any RBCs in the urine. The conventional definition of hematuria on urine microscopy is greater than 3 RBCs/HPF or 1000 RBCs/ml. When reported, the morphology of any RBCs seen on microscopy can be helpful, as the presence of RBC casts and dysmorphic RBCs—particularly acanthocytes (ringform RBCs with blebs protruding off of it)—suggest the hematuria is glomerular in origin.
It is important to consider that urine dipsticks and microscopy signal hematuria, not necessarily disease. Epidemiological studies looking at the proportion of patients with hematuria who have serious disease have yielded inconsistent results, with rates of significant urologic disease among patients with microscopic hematuria varying from 17% to 56% in different studies. Mariani et al evaluated 1000 consecutive adults with asymptomatic hematuria (microscopic or macroscopic). Of these patients, 9.1% had what was deemed a life-threatening condition, most commonly bladder transitional cell cancer or renal adenocarcinoma. Significant findings, such as nephrolithiasis or cystitis, occurred in 22.8% of patients. In 56.4% of patients, only insignificant findings resulted from the evaluation of their hematuria, and in the remaining 11.7% of patients, no diagnosis to explain the hematuria was obtained. The authors of this study assert that there is no lower limit to the number of RBCs in the urine that rules out serious underlying disease, given that 18.6% of patients with life-threatening conditions produced a urinalysis with fewer than 3 RBCs/HPF within 6 months of the cancer diagnosis.
Unexplained hematuria requires further evaluation. The most important goal of this further evaluation is to assess for the presence of cancer affecting the kidney or urinary tract. In general, patients with unexplained hematuria should undergo imaging, such as a CT scan, urine cytology testing, and also be referred to a urologist for cystoscopy.
Measuring proteinuria is an important part of evaluating any patient with known or suspected renal disease, as heavy proteinuria can signal significant glomerular disease, or can be a consequence of renal involvement in a systemic disease, such as multiple myeloma. Proteinuria > 150 mg/day is considered abnormal. Microalbuminuria refers to urinary albumin excretion of 30–300 mg/day. Microalbuminuria is commonly used to detect early renal disease in diabetic patients, and so is mainly used in the primary care setting rather than the inpatient setting. When proteinuria exceeds 3.5 g/day, it is in the nephrotic range, and when accompanied by hypoalbuminemia, edema, and hyperlipidemia, full-blown nephrotic syndrome is present. Not only is the level of proteinuria diagnostically useful, but it is also a risk factor for progression of chronic kidney disease in both diabetic and nondiabetic patients.
There are 3 different methods of assessing for proteinuria: (1) a 24-hour urine collection for protein excretion; (2) a spot (ie, untimed) urine protein-to-creatinine ratio; and (3) a urine dipstick. The 24-hour urine collection, while often used as a reference standard, has a limited role in routine inpatient clinical practice. The 24-hour urine collection is inconvenient. Incomplete collections—which compromise the accuracy of this method—are common, and other methods will often provide the necessary clinical information. Under most circumstances, a spot urine can be obtained for a protein-to-creatinine ratio, which gives an estimate of the 24-hour protein excretion in the urine.
One study by Lane et al, which looked at 103 patients in a renal and hypertension clinic, concluded that as the level of proteinuria increased, the accuracy of the spot urine protein-to-creatinine ratio decreased, and that at levels of proteinuria above 1 g/24 h, the spot measurement was inadequately accurate, and a 24-hour collection should be used instead. The authors argue that the spot measurement is helpful in answering whether the patient has significant proteinuria, but if the exact amount of that significant proteinuria needs to be known (such as for following the effect of treatment), then a 24-hour urine collection should be used. Nonetheless, in the inpatient setting, a spot urine protein-to-creatinine ratio will in most cases provide the necessary information.
Most urine dipsticks can detect albumin concentrations of 200–300 mg/L. There are semiquantitative assays, usually reporting the amount of proteinuria as 1+ to 3+ or 4+. Importantly, most dipsticks are insensitive for nonalbumin proteinuria, such as the light chains that may occur in the setting of myeloma kidney (Bence Jones proteins). Therefore, when there is a suspicion of nonalbumin proteinuria, other methods of assessing for proteinuria besides a dipstick should be used, such as a spot urine protein-to-creatinine ratio performed by the clinical laboratory. Sulfosalicylic acid, when added to a urine sample, will cause turbidity in the presence of protein in the urine (both albumin and nonalbumin protein) and so can be used as a point-of-care test to detect nonalbumin proteinuria (with a reported sensitivity of 76.7% and specificity of 75.4%). Another limitation of urine dipstick testing for proteinuria is that it is affected by the concentration of the urine sample being used. Using the albumin-to-creatinine ratio as the reference standard, a study of 310 general hospitalized patients by Pugia et al found that dipstick assessment for albuminuria had a positive predictive value of 82% and a negative predictive value of 99%. The authors concluded dipstick testing had good agreement with the quantitative albumin-to-creatinine ratio.
The urine dipstick features used to diagnose a urinary tract infection (UTI) include the presence of leukocyte esterase and nitrites. White blood cells (WBCs) on urine microscopy and an organism isolated on urine culture help confirm the diagnosis. Leukocyte esterase is an enzyme contained in WBCs, while nitrites are a product resulting from the metabolism of nitrates by certain bacteria. Bacteria that can convert nitrates to nitrites include E. coli and other bacteria in the family Enterobacteriaceae. However, a number of important urinary pathogens do not produce nitrites, including S. saprophyticus, Pseudomonas, and enterococci. Certain factors can cause a false negative nitrite test on dipstick testing, including insufficient nitrates in the diet to be converted by the bacteria and a dipstick that has been exposed to the air for a prolonged period. It takes at least 4 hours for bacteria to convert nitrates to detectable levels of nitrite, and so this test may be negative in patients with UTIs whose urinary frequency does not provide adequate time for bacterial conversion of nitrates to nitrites. The presence of urine eosinophils or Trichomonas in the urine can lead to false positive results. Factors that can lead to false negative results include the presence of ascorbic acid, high levels of glucose or protein, and certain antibiotics, including cephalexin and tetracycline.
A systematic review by Hurlbut et al of the test characteristics of dipstick leukocyte esterase and nitrite to detect UTI, which used > 100,000 cfu/ml on the urine culture as the reference standard, found that considering either positive leukocyte esterase or positive nitrite to denote a positive test for UTI yielded the greatest area under the ROC curve. Considering either leukocyte esterase positive or nitrite positive to be a positive result for UTI yielded a sensitivity of 75% and a specificity of 82%. This review and other reviews concluded that in the setting of a high clinical suspicion, a dipstick negative for both leukocyte esterase and nitrite is not sufficient to exclude a UTI. A recent study of 408 women by Little et al found similar results. Using either a dipstick positive for nitrite or positive for both leucocytes and blood to be a positive test yielded a sensitivity of 77% and a specificity of 70%, using culture data as the reference standard.
In assessing the relationship between the degree of pyuria and likelihood of bacteriuria, measuring the leukocyte excretion rate is the most diagnostically useful. However, this method is cumbersome, and so the method employed in most clinical laboratories to measure pyuria is counting the number of WBCs per HPF in the centrifuged urine sample. A common break point used by clinical laboratories is ≥5 WBCs/HPF as being abnormal pyuria, though the break points used by clinical laboratories vary. In general, the higher the number of WBCs, the more likely there is to be significant bacteriuria. One older study by Holm et al found that among patients with 0–1 WBCs/HPF, 3% of patients had urine cultures with >103 bacteria/ml, whereas among patients with >9 WBCs/HPF, 87% had >103
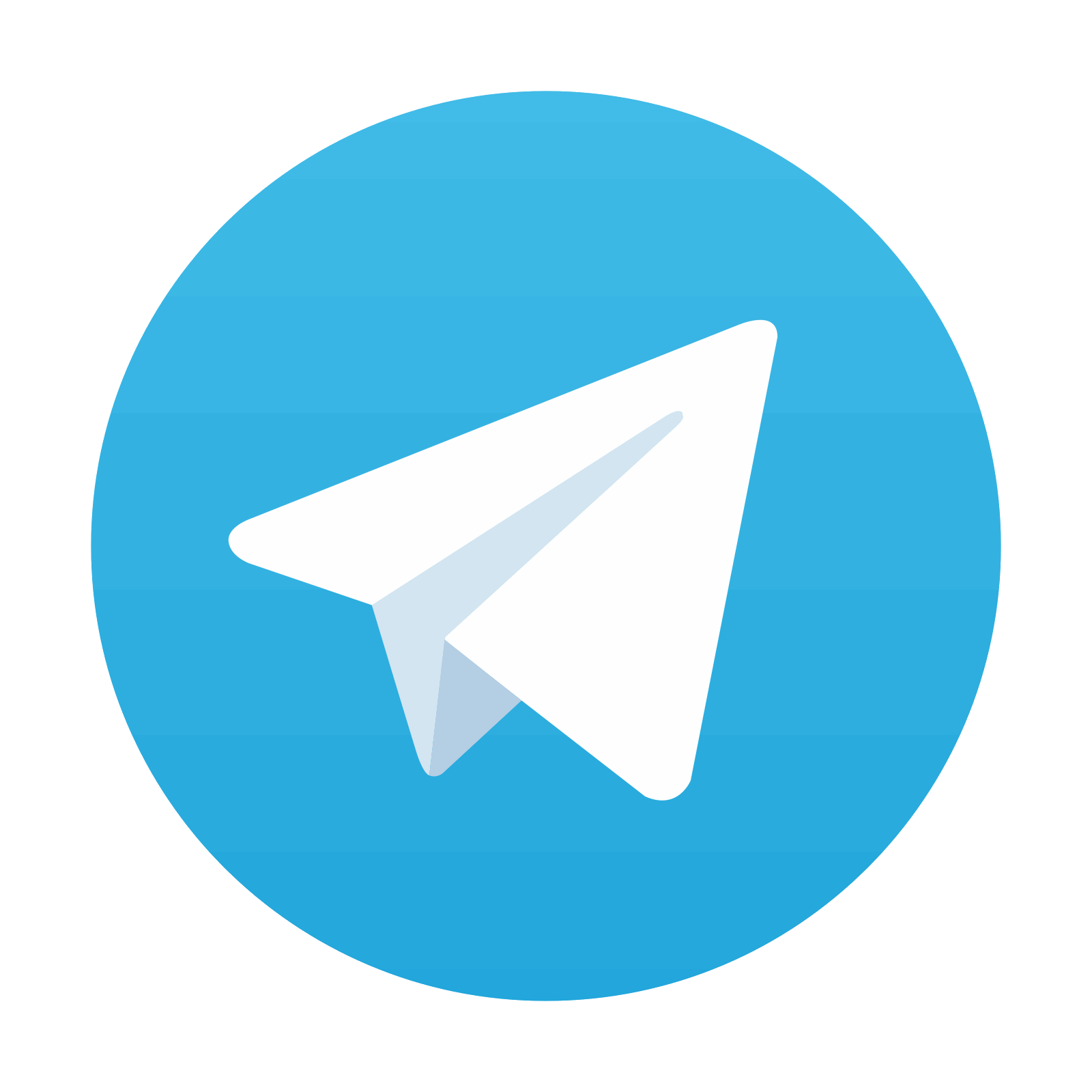
Stay updated, free articles. Join our Telegram channel

Full access? Get Clinical Tree
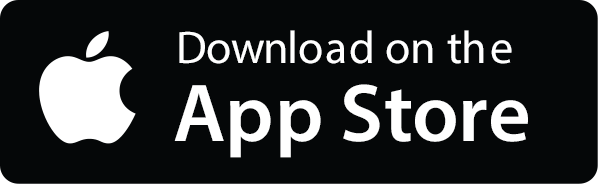
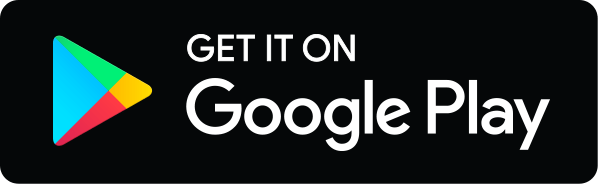
