A full-term baby is born with APGAR scores of 4/6. The neonatologist attempts stimulation of the baby and then proceeds with two failed attempts to intubate the trachea with a Miller 0 and 1 blade, with no clear view of the vocal cords. You are immediately called to the NICU to help with airway management. You notice the baby in obvious signs of respiratory distress including tachypnea and upper airway obstruction. The baby’s oxygen saturation is 93% with an oral airway and face mask. What are your concerns and how would you manage this child’s airway?
The Pediatric Perioperative Cardiac Arrest (POCA) Registry is a subdivision of the ASA Closed Claims Registry specifically dedicated to pediatrics. According to the POCA Registry during the 1970s and 1980s, 50% of all cardiac arrests were due to respiratory causes primarily with hypoxemia quickly leading to bradycardia and cardiac arrest.1 Recently, a multicenter study of 1018 children with difficult airways demonstrated that greater than two direct laryngoscopy attempts in children with difficult tracheal intubation are associated with high failure rates and an increased incidence of severe complications.2 The most common severe complication was cardiac arrest occurring in 2% of these children. The most common complication overall was hypoxemia.
Thanks to the advent of pulse oximetry and better monitoring, and perhaps better medications and equipment, respiratory causes are now the second most common reason for death and brain damage, although inadequate oxygenation continues to account for approximately 25% of all pediatric cardiac arrests. Currently, the number one reason for death and brain damage is cardiovascular causes such as unrecognized hypovolemia.1
Surprisingly, even though children with ASA Physical Status 3 to 5 are at higher individual risk, two-thirds of children suffering perioperative death or permanent brain damage are with ASA Physical Status 1 and 2.
Most pediatric anesthesia practitioners are not pediatric specialists; over 80% of all pediatric anesthesia care in the United States is provided in non-pediatric centers as part of a mixed practice, usually for pediatric patients with ASA Physical Status 1 and 2.
Fortunately, there are more similarities between the pediatric and the adult airway than there are differences. The traditional emphasis on the differences between adults and children is unfortunate, and likely impairs the performance of an anesthesia practitioner that deals mostly or exclusively with adults, when faced with an ill child for fear that they will do something “wrong.” When compared to adults and older children, the biggest differences are found in the child less than 2 years of age. For this reason, this age group will be emphasized. The purpose of this chapter is to provide essential information and recommendations regarding pediatric airway management that is evidence-based, and practical in such settings.
Variability in equipment sizes, drug dosages, and the propensity of children to be both uncooperative and to have rapid oxygen desaturate can make even the most experienced anesthesia practitioner wary. There is also no time to calculate drug doses or discover mis-sized or inadequate equipment in a crisis situation. Calculating medication doses and equipment sizes is not algorithmic, does not translate into a reflexive skill, and will invariably increase cognitive load. Errors happen: the POCA database estimates 18% of children received wrong drug doses and contributed to their morbidity and mortality.3
Immediate access to such dosing and sizing information in any clinical scenario, including planned and unplanned difficult airway situations, will allow the anesthesia practitioner the “mental space” to focus on critical decision-making. The authors enthusiastically support the use of pre-calculated systems for both drug dosages and equipment sizes. This can be done a number of ways, such as based on the actual weight, or the length of the child, using a system such as the Broselow–Luten tape (Armstrong Medical, Lincolnshire, IL).4,5 Other settings have taken the approach of including drug doses in the front of every child’s chart. However, this assumes both the creation and presence of a chart.
Having a system that provides information on drug dosages and equipment sizes during a resuscitation situation decreases cognitive load,6 allowing the anesthesia practitioner to focus on what is essential, clinical decision-making.7
Montreal Children’s Hospital reported an incidence of 6.6% for unexpected difficult bag-mask-ventilation (BMV), requiring additional management techniques to provide adequately ventilation in a sample of 484 healthy children aged 0 to 8 years undergoing elective surgery.8 Decreased age was the only significant risk factor for difficult BMV. Obesity, gender, type of surgery, OSA, and asthma were not associated with difficult BMV. This incidence is similar to rates for non-high-risk adult patients. Interestingly, there was no association between difficult BMV and difficult tracheal intubation.
The Children’s Hospital of Philadelphia reported a difficult laryngoscopic intubation rate of 0.25% or 16/6524 patients over a 5-month period.9 Notably, the majority (14 of the 16) were anticipated. The rate of unanticipated difficult direct laryngoscopic intubation rate was therefore 0.03%, with no patients being unable to be oxygenated with BMV. An extraglottic device (EGD) was inserted in 44% of patients as a bridge to intubation. The most common physical signs in this group were narrow inter-incisor distance (93.8%) and mandibular hypoplasia (87.5%). Akpek et al.10 reported a difficult intubation rate of 1.25% or 16 of 1278 pediatric cardiac patients, again in a pediatric center. Half of the difficult intubation patients were syndromic and the other half had an extreme anterior airway and micrognathia. In adults, the incidence of unanticipated difficult laryngoscopic intubation is approximately 5.8%.11
Heinrich et al.12 reported the overall incidence of difficult laryngoscopy to be 1.35% among 11,219 patients undergoing general anesthesia at pediatric tertiary care center over a 5-year period. The incidence of Cormack–Lehane (C/L) 3/4 was significantly higher in patients younger than 1-year-old (4.7% vs. 0.7%). ASA Physical status III/IV, higher Mallampati score (III/IV), and lower BMI were all associated with difficulty direct laryngoscopy. Oral maxillofacial and cardiac surgery patients had higher rate of C/L 3/4.
Unanticipated difficult intubation in children is less common than in adults, but can occur in up to 20% of children.2 Fortunately, most difficult airway situations are in children with a syndromic diagnosis, rarely unanticipated, and allow for planning prior to induction. A few examples of both congenital and acquired syndromes that can lead to difficult laryngoscopy or intubation in children are listed in Table 45–1.
Examples of Commonly Encountered Pediatric Syndromes with Airway Implications and Possible Management Techniques
Pierre Robin syndrome
Mucopolysaccharidosis
Down’s syndrome
Treacher Collins syndrome
Cystic hygroma
Juvenile rheumatoid arthritis
|
While the exact incidence is unknown, this is a rare event. Children with a potentially difficult tracheal intubation are often easy to mask-ventilate, and difficulty in intubation is usually anticipated. There are always exceptions to this generalization. A child with a difficult airway is often detected prenatally and has an associated craniofacial syndrome13 (Pierre Robin, Goldenhaar, Treacher Collins, cystic hygroma, also see Figures 45–1 and 45–2 and Table 45–1). At birth, if the newborn is unable to maintain their airway, a tracheotomy is placed or an ex-utero intrapartum treatment (EXIT) procedure is performed by an otorhinolaryngologist.14 If obstruction is mild to moderate, they may undergo procedures to advance their mandible or improve mouth opening during the first few days of life. This is the population that may be at higher risk for CICO scenario.
Guidelines have been developed for management of the unanticipated difficult airway in children from 1 to 8 years. These guidelines were developed by an expert panel and provide algorithms for difficult mask-ventilation, unanticipated difficult tracheal intubation, and a “CICO” scenario. The principles of unanticipated airway management are the same in children and adults; oxygenation, not intubation saves lives.15
The pediatric upper airway consists of the nose and mouth leading to the nasopharynx and oropharynx respectively. These structures are contiguous. The ala of the nose leads to two separate nasal compartments separated by the nasal septum. The lateral wall of each nasal compartment has three scroll-like conchae or turbinate bones. The major air passage lies below the inferior turbinate and is the preferred route for nasal intubation.
The posterior nasal aperture leading to the nasopharynx is called the choanae. Choanal atresia occurs in approximately 1:7000 births, however, only approximately 50% of these babies will have respiratory distress. Although it was once thought infants are obligatory nose breathers, it is now thought infants are more accurately described as “preferential nasal breathers.”16 If infants have respiratory distress, they will usually cry, raise their soft palate, and instantly become mouth breathers. This is borne out by the observation that when infants get a stuffy nose, they do not suffer hypoxic brain damage but are more likely to appear uncomfortable because of the energy required to contract their soft tissues in order to breathe through the mouth.17
From infancy until approximately 2 years of age, the tongue is relatively large surrounded by a small jaw. There is simply not enough space to sweep the tongue to the left of the oral cavity as occurs with direct laryngoscopy when employing a curved Macintosh blade in an adult. For this reason, a straight or Miller blade is generally preferred in this age group. Of note, a recent study demonstrated that in children <2 years of age, optimal laryngeal views may be obtained with either the Miller size 1 blade lifting the epiglottis or with the Miller or Macintosh blades lifting the tongue base.18
Traditionally, the airway of a child under 2 years old has been described as “cone shaped” with the narrowest part being at the level of the cricoid cartilage, the only complete cartilaginous ring of the respiratory tract. Recent studies however question whether this is actually true. Cadaveric studies originally described the narrowest part of the pediatric airway being the cricoid ring, but this may be artifactual. Studies using bronchoscopy19 and MRI imaging20 show the narrowest part of the pediatric airway to be at the level of the vocal cords, the same as an adult. These studies were performed on children under general anesthesia without NMB and therefore preserved muscle tone. Studies in paralyzed children are still required. This may help to explain why cuffed endotracheal tubes in children even in infancy are very well tolerated without an increase in complication rates both in the intensive care21 and operative settings.22
As with the anatomy of the airway, the major physiologic differences between adults and children occur in the first 2 years of life.
Young children consume oxygen at two to three times the adult rate (6 mL·kg−1·min−1 vs. 2 mL·kg−1·min−1), and have a smaller functional residual capacity (FRC) under general anesthetic relative to adults (10–15 mL·kg−1 vs. 30 mL·kg−1 respectively). The result is that young children consume their oxygen reserve more quickly than adults and hence oxygen desaturation occurs more rapidly.
Although children have tidal volumes similar to adults (7 mL·kg−1), their dead space to tidal volume ratio is larger, leading to proportionately less alveolar ventilation per breath. In children, an increase in demand in ventilation is primarily achieved by increasing the respiratory rate rather than the depth of each breath. Taken together, this means that respiratory distress in an infant may be subtle and heralded by tachypnea. In turn, because infants and small children have more Type 2 respiratory muscle fibers, they are prone to fatigue. By 2 years of age, children have developed a rich supply of Type 1 respiratory muscle fibers, which do not fatigue as easily.
Additionally, because the rib cage of young children is highly compliant due to its cartilaginous nature, increased work of breathing doesn’t necessary translate into better alveolar ventilation as the chest wall collapses or “indraws,” as seen in the case presented. The diaphragm is also very compliant leading to a cephalad shift in the face of abdominal distension such as with a bowel obstruction and distension (as in this case) or gastric distension due to overzealous noninvasive BMV. This limits reserve and achievable tidal volume while increasing the work of breathing. The ideal maneuver is to decrease the amount of gut distension by decompression with a nasogastric tube if possible.
Unlike metabolic acidosis, respiratory acidosis is exceptionally well tolerated in children. Goldstein et al.23 reported a case series of five young children, aged 1 day to 6 months, in an intensive care setting who underwent permissive hypercapnia for a period of 35 minutes to 2 days. Arterial carbon dioxide values ranged between 155 and 269 mm Hg with accompanying pH values of 6.76 to 7.10. The only acute change was generalized slowing of their EEG tracing. All five infants made a complete neurologic recovery. Although only a small study, it does beg the question whether carbon dioxide values in an acute difficult airway situation really matter.
This may explain why the description of a failed airway has changed from “can’t intubate, can’t ventilate” to the more accurate “can’t intubate, can’t oxygenate” in recent difficult airway literature.24
The mnemonics presented earlier (Chapter 1) with respect to the evaluation of the adult airway for difficulty are: MOANS for difficult BMV; RODS for difficult EGD; LEMON for difficult direct laryngoscopic intubation; CRANE for difficult indirect (video) laryngoscopic intubation; and SHORT for a difficult surgical airway.
These mnemonics do not translate directly to small children. The classification system suggested by Hall25 (below) provides a framework that prepares the anesthesia practitioner with limited pediatric airway management experience to anticipate airway management difficulty and formulate Plans A, B, and C accordingly.
Hall25 described four types of a pediatric difficult airway:
Congenital abnormality resulting in chronic upper airway obstruction (e.g., Pierre Robin [also known as Robin sequence], see Chapter 48).
Congenital or acquired abnormalities resulting in difficult direct laryngoscopy (e.g., retrolingual tonsil or tonsillar tissue in the vallecula).
Infectious, tending to be progressive (rapidly or slowly depending on the type and degree of infection). All fascial layers in the neck are contiguous meaning that infections can spread to adjacent facial planes.
Foreign body, which can suddenly lead to a complete obstruction.
Although this is helpful when thinking of the types of various difficult airways, the classification per se does not immediately lead to an airway plan. Additionally, some children may have overlap categories (e.g., a child with Down’s syndrome with a postoperative tonsillar bleed). Furthermore, difficult direct laryngoscopy in Group 2 does not necessarily mean difficult airway. Given the explosion of available alternatives to direct laryngoscopy such as video-laryngoscopy or flexible bronchoscopic intubation through an EGD, intubation may be relatively straightforward using alternative devices or a combination of devices.
The first question when managing a difficult airway is whether a child should be awake or asleep. If the child will be put to sleep, the second question is whether the child should be paralyzed. As a general rule, if a child is dependent on his or her own intrinsic muscle tone to keep an airway open, don’t paralyze. For example, in infectious disease26 or foreign body scenarios,27 the anesthesia practitioner should seriously consider maintaining spontaneous ventilation.
In a 2005 survey of Canadian pediatric anesthesiologists, the majority favored spontaneous ventilation for all difficult airway scenarios they were given except where the airway was shared with a surgeon. Most preferred an inhalational technique while maintaining spontaneous ventilation.28
How Do I Plan for a Difficult Airway in Children? How Does My Planning Change Depending on Whether It Is an Anticipated or Unanticipated Difficult Airway?
Airway management in children needs exactly what their adult counterparts do: effective oxygenation. A paradigm shift, where oxygenation, not intubation, is the “gold standard” in airway management prevents task fixation (i.e., repeated attempts at intubation in the setting of poor oxygenation). Research performed in an urban EMS system has shown there is no difference in survival or neurologic outcome between BMV and endotracheal intubation by paramedics in the field.29
Planning for the anticipated difficult airway includes communication with all members of the health care team including parents and the child if appropriate. The airway management plan must be clearly documented and followed. In particular, the parents and child (if appropriate for that child) know what the bottom-line plan is, particularly if a surgical airway is a possibility (Table 45–2).
Management of Anticipated versus Unanticipated Pediatric Difficult Airway
Anticipated
Unanticipated
|
Tube sizes are measured in millimeters of internal diameter (ID) with the external diameter (OD) dependent on the material used and the manufacturer. The smallest uncuffed tube is 2.0-mm ID. The smallest cuffed tube is 3.0-mm ID. Sizes increase by 0.5 mm increments and the OD can vary greatly for the same ID.30 Because of the large tracheal diameter of the adult airway (20–25 mm) this factor may not be of significance. However, in children with much smaller tracheal diameters depending on age,31 the size of the endotracheal tube wall may be a significant consideration. Pediatric endotracheal tubes also vary with respect to their beveled end and the presence of a Murphy eye. Some endotracheal tubes have a flexible “beaked” tip that may help in advancing the endotracheal tube over a flexible bronchoscope (e.g., Parker Endotracheal Tube, see Figure 10–22), although it has also been shown that this “beak” potentially partially obstruct the distal opening of the ETT.
The location and material of the cuff on cuffed endotracheal tubes will also vary with the manufacturer. Most cuffs are made from polyvinylchloride (PVC) but can also be made of thinner polyurethane. Polyurethane used in the pediatric Microcuff (Kimberly Clark, Atlanta, GA) has a lower sealing pressure than PVC.32 An excellent review regarding the various types of pediatric tubes has recently been published by Leong and Black.30
The exact size of tube that ought to be selected for a particular patient can be estimated by several means. Perhaps the best method is to use the length of the patient, such as used in the Broselow–Luten tape. Patient age is also commonly used. The Cole formula33 originally published in 1957 based on age has been modified to:
Age/4 plus 4
The modified Cole formula was designed to be used for uncuffed tubes. If anything, it underestimates uncuffed tube size. It is recommended that the formula be modified for cuffed tubes to:
(Age/4 plus 4) minus 0.5
Neither the size of the smallest finger of a child nor the size of the external nares estimates endotracheal tube size accurately.
In any case, the cautious anesthesia practitioner has the size predicted (determined by whatever means) and both a half size larger and smaller immediately available.
Cadaveric studies originally described the narrowest part of the pediatric airway being the cricoid ring. This led to a tradition of using uncuffed tubes in children because the cricoid ring was thought to provide a snug seal for the tube without the need for a cuff. Anesthesia practitioners also preferred uncuffed tubes because older pediatric cuffed endotracheal tubes possessed high-pressure low-volume cuffs that were associated with ischemic airway mucosal damage and subsequent subglottic stenosis.
Recently, a prospective randomized controlled multicenter trial22 studied the use of uncuffed tubes from various manufacturers and compared them to Microcuff tubes in children under 5 years of age. This study randomized over 2000 children and found the incidence of postoperative croup to be similar. The authors of the study, however, emphasized that cuff pressures need to be monitored. The average cuff pressure was 10.6 cm H2O, well under the threshold pressure of 25 cm H2O that had been shown in past studies using uncuffed tubes to increase morbidity.34 It should also be noted that the conclusions drawn from this study might not be valid for cuffed endotracheal tubes from other manufacturers with thicker cuff walls, requiring perhaps higher cuff pressures needed to achieve a similar seal.
A most significant finding of this large study was that uncuffed tubes needed to be switched to an alternative size in 30% of patients. Cuffed tubes were switched out for another tube size only 2% of the time. Estimation of appropriate sizing for all endotracheal tubes was done as per each center’s guidelines.
Preventing the need to switch endotracheal tube sizes in a difficult airway situation obviously offers tremendous benefit; emphasizing the recommendation that cuffed tubes be considered in this setting. This is a pivotal study and, with the accompanying editorial stating “Thus, the ‘uncuffed camp’ will now have to prove, based on prospectively gathered data, that the use of adequately designed cuffed tracheal tubes is in fact harmful to the pediatric airway.”35
There is no question that damage to the airway can occur with both cuffed and uncuffed tubes.31,32,36,37 The anesthesia practitioner needs to reflect on the specifics of the tracheal tubes they use (e.g., different manufacturers have the cuffs in different positions, and some can herniate onto the vocal cords), as well as the sizing. Finally, it has been shown that movement of the child increases the incidence of airway damage.
Over the past 10 years, a number of new devices have been made available in pediatric sizes for routine, difficult, and failed airway management. These devices can be divided into two groups: optical devices that are similar to the traditional laryngoscope and optical stylets.
The video/optical laryngoscopes include the GlideScope ® (Verathon, Bothwell, Washington), the STORZ Videolaryngoscope™ (Karl Storz Endoscopy, Tuttlingen, Germany), and the Truview EVO2™ (Truphatek, Netanya, Israel). These devices combine and incorporate video technology into the tip of the laryngoscope blade. This allows images from beyond the tip of the blade to be displayed on video monitors permitting one to “free hand” the ETT into the trachea. The GlideScope® incorporates a 60-degree angulated distal tip (see section “GlideScope Video-Laryngoscope (GVL, Verathon Inc., Bothell, WA)” and Figures 11–13, 11–14, and 11–15 in Chapter 11). The Truview EVO2™ is a rigid laryngoscope with an angulated tip that uses a series of prisms to transmit the image from the distal tip to an eyepiece. The tip is angulated 46 degrees anteriorly from the direct line of sight, and the device provides a wide-angle magnified view. A new version of this device called the Truview PCD has a video camera and screen that is mounted to a proximal eyepiece converting it to a video-laryngoscope.
The Airtraq™ (Prodol Meditec SA, Vizcaya, Spain) is a novel device that combines a video image with a tube delivery capability. The image provided to the practitioner is a magnified wide-angle view transmitted via a series of mirrors similar to that of a submarine periscope. Once the glottis is viewed, an endotracheal tube that has been preloaded into the ETT delivery channel is advanced into the trachea. This is a single-use device with anti-fogging capability at the distal tip of the blade. There is no need for an external monitor (see section “Airtraq Laryngoscope” and Figure 11–6 in Chapter 11). A randomized, controlled trial of 20 infants and 40 children found longer intubation times for children and better laryngeal views for all patients with the Airtraq compared to direct laryngoscopy.38
The optical stylets combine the optics of a flexible bronchoscope with the features of a lightwand. Those available for pediatric use include the Shikani Optical Stylet™ (Clarus Medical, Minneapolis, MN) a semi-malleable stainless steel sheath housing a fiberoptic bundle connected to a distal light source with a fixed eyepiece (see section “What Are the Characteristics of the Shikani Optical Stylet?” and Figure 11–2 in Chapter 11); and the Bonfils Intubation Fiberscope™ (Karl Storz Endoscopy, Tuttlingen, Germany), a rigid optical stylet with a swiveling eyepiece (see section “What Are the Characteristics of the Bonfils Retromolar Intubation Fiberscope?” and Figure 11–1 in Chapter 11).
All of the above devices have been used to manage the pediatric difficult airway in case series and case reports.39–49 Larger pediatric studies regarding these devices are ongoing. Many of the prospective studies involving the GlideScope®50–52 and video-laryngoscope53,54 compared to direct laryngoscopy show that both these devices provide an improved view of the glottis in children with normal airway anatomy, but require a longer time for intubation. The longer time is probably not significant in clinical practice, and the benefit offered by an improved view probably outweighs the minimal increase in time for tracheal intubation. It is hypothesized that the longer time is due to inexperience with optical-laryngoscopes compared to direct laryngoscopy for experienced anesthesia practitioners.
The GlideScope® Cobalt was designed for use in children and was shown to have similar intubation times and success rates compared to direct laryngoscopy in anatomically normal neonates and infants (best view faster, tube passage longer) in a prospective randomized trial with 60 children.55
Video-laryngoscopes have been demonstrated to improve the view in adults with difficult airways and evaluations are ongoing in children. Two recent small series demonstrate improved laryngoscopic views with the GlideScope® compared to direct laryngoscopy in children with a history of difficult airway or failed intubation.56,57
A pilot comparison between the Airtraq and Storz system found no difference in the success rate of intubation for 10 children less than 2 years old with a normal airway assessment.58
Optical-assisted laryngoscopes may be valuable for inexperienced anesthesia practitioners who encounter an infant with a difficult airway. A study of 30 anesthesia residents attempting to intubate the trachea of an infant model of difficult airway with airway obstruction and neck immobilization found higher success rates and shorter intubation times with optical-assisted laryngoscopy compared to direct laryngoscopy.59
With the exception of bronchoscope diameter, there is no material difference between a flexible “pediatric bronchoscope” and an “adult bronchoscope.” Generally, a bronchoscope (50–60 cm in length) that is less than 4.0 mm in tip diameter is called a “pediatric bronchoscope.” Those larger than 4.0 mm in tip diameter tend to be called adult bronchoscopes.
The flexible bronchoscope scope remains the cornerstone of pediatric difficult airway management. Fiberoptic image transmission is being replaced by CMOS camera technology providing exceptionally high-quality images. These high-definition scopes with a “working channel” are limited in size, the smallest being approximately 2.8 mm in tip diameter. Ultra-thin bronchoscopes without a suction channel have a tip diameter of 2.2 to 2.5 mm and are generally used as nasoendoscopes used by our ENT colleagues. Bronchoscopes with a suction channel have an outer diameter of at least 2.8 mm. The smallest flexible bronchoscope with a suction channel (2.8 mm) has poorer optical quality than the 2.5-mm scope without the suction channel because the channel takes up considerable space at the expense of light and optical fibers.
EGDs that are available for use in the pediatric population (below age 2 and <30 kg) include the following (see Chapter 13):
the LMA family of devices including the LMA-Classic™, the LMA-Unique™, the LMA-Proseal™, and LMA-Supreme (Teleflex, Triangle Park, NC)
the air-Q™ (air-Q™) (Mercury Medical, Clearwater, FL)
i-gel (Wockingham, UK)
The Combitube and the Easy Tube are available in small adult sizes making them potentially useful for children older than 10 to 12 years, as rescue devices.
Which Rescue Devices Are Available for Pediatric Patients? Is There an Intubating EGD for Pediatric Patients?
The above named EGDs constitute the mainstay of nonsurgical rescue airway devices for infants and children <10 years of age. The most commonly used EGD in children is the LMA-Classic™. The LMA-Proseal™ provides a better laryngeal seal pressure than the LMA-Classic™,60,61 and incorporates an esophageal drain tube (second-generation EGD) and a bite block. This device has been used with greater success when positive pressure ventilation is desired.62 Other devices used include the King LT/Laryngeal Tube™, and the i-gel®. The i-gel® is another second-generation EGD which has been studied extensively and is a suitable conduit for positive pressure ventilation.63,64 In theory, second-generation EGDs may reduce the risk of aspiration of gastric contents, and allow for ventilation of the lungs at higher peak inspiratory pressures.
EGDs may be helpful in patients that are difficult to mask-ventilate.15 They can be utilized effectively in children with difficult laryngoscopy, and can be used as an alternative to tracheal intubation.65
There is no LMA-Fastrach™ (Intubating LMA) for pediatric patients. The smallest of these devices is a size three which has been used successfully in children >12.
The air-Q™ can be utilized as a conduit for tracheal intubation, even in smaller children and overcomes some of the limitations that have been associated with LMA-Classic™ guided intubation.66 There is a high incidence of epiglottic downfolding, even with adequate ventilation through the EGD. Therefore, flexible bronchoscopy is recommended to assist with tracheal intubation. The air-Q has been shown to be an effective conduit for tracheal intubation in children.67–69
A summary of the devices available along with the clinical advantages and limitations for the management of the difficult pediatric airway is shown in Table 45–3.
Devices Available Along with Clinical Advantages and Limitations for the Management of the Difficult Pediatric Airway
Device | Advantages | Limitation |
---|---|---|
GlideScope® |
|
|
Video-laryngoscope |
|
|
Airtraq™ |
|
|
Flexible bronchoscope |
|
|
|
|
|
LMA-Classic™ |
|
|
|
|
|
air-Q™ |
|
|
i-gel® |
|
|
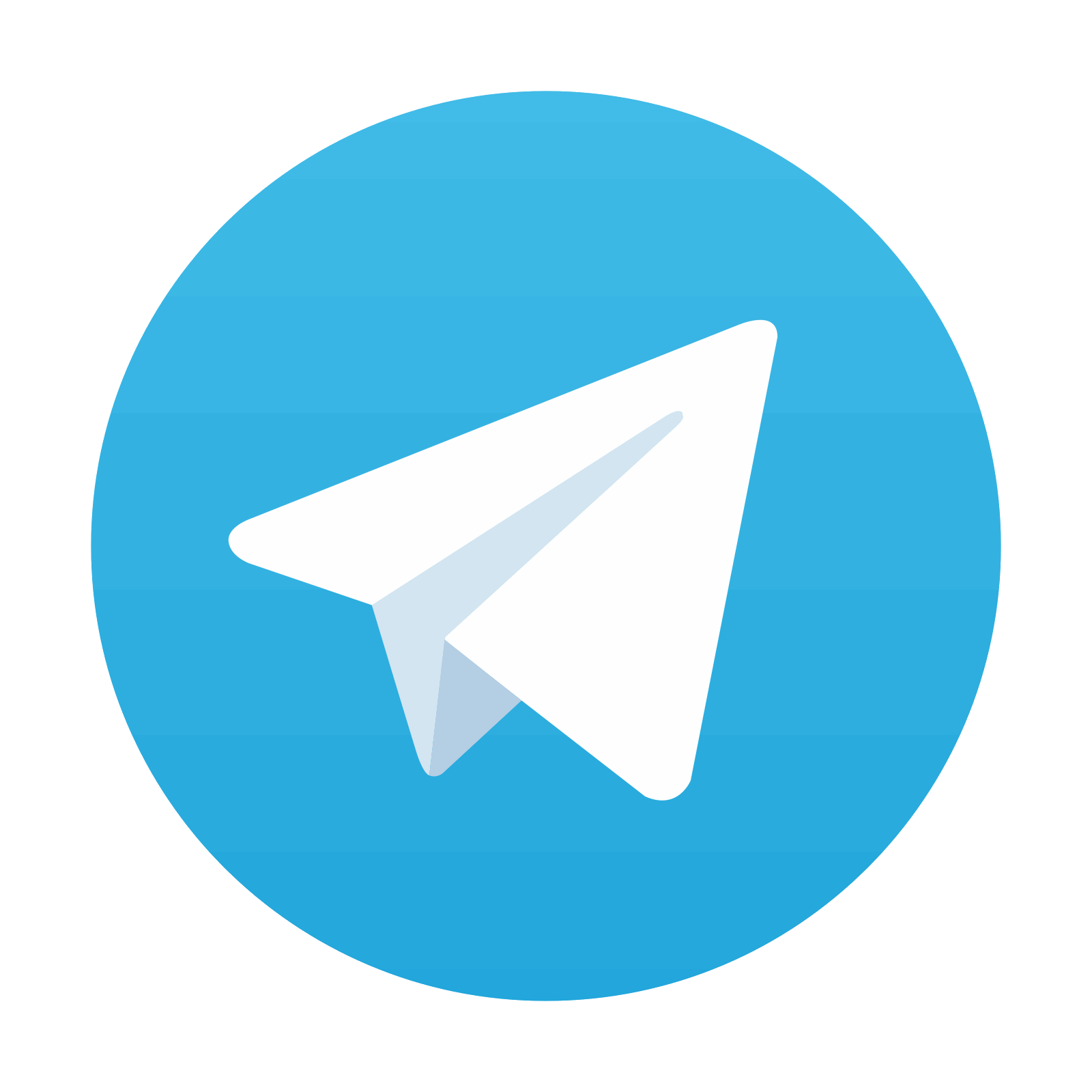
Stay updated, free articles. Join our Telegram channel

Full access? Get Clinical Tree
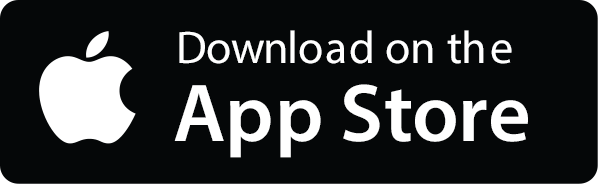
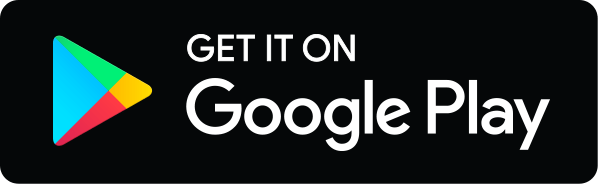
