It is crucial for clinicians performing transesophageal (TEE) examinations to understand how the controls on an ultrasound machine alter the display. Without this knowledge, it is impossible to consistently optimize images, and unskilled manipulations may misrepresent diagnostic information and result in missed diagnoses. This chapter describes the controls found on most ultrasound machines, how they affect the image, and how they are used to optimize the ultrasound image. Table 2–1 presents the most commonly used controls for two-dimensional (2D) imaging.
2D Variable | Knob(s) | Function |
---|---|---|
Gain | Gain | Amplifies returning signals before display |
TGC/DGC | TGC/DGC toggles | Selectively amplifies returning signals before display (horizontally) |
LGC | LGC toggles | Selectively amplifies returning signals before display (vertically) |
Compression | Compression | Changes the difference between the highest and lowest received amplitudes (shades of gray) |
Power | Power (dB) | Controls rate at which energy is propagated into an imaged medium |
Frequency | Dependent on probe | Determines number of times/second a sound wave completes a cycle |
Focal zone | Focal zone | Alters the placement of the narrowed region that designates an area of improved resolution |
Depth | Depth | Selects how shallow or deep an area is imaged |
Sector size | Size, trackball | Narrows or widens the image sector |
Zoom | Zoom | Magnifies a particular area of interest within the sector |
Freeze | Freeze | Stops or starts live imaging |
Measurement | Freeze, caliper, trace, enter, erase | Quantifies features of a 2D image |
Harmonics | Harmonics | Uses frequencies created by the tissues, rather than the fundamental frequency, to create an image |
Annotation | Annotation | Adds text or picture to image |
After providing power to the machine itself, a TEE probe must be connected to the machine, register as compatible with the machine, and be selected from other possible transducer options. The basic parameters for the ultrasound examination may be defined by choosing an appropriate TEE preset. The preset provides a starting point for basic machine settings such as depth, gain, and image processing settings. The operator can adjust all the machine’s variables from the initially fixed settings, as needed. Adjustments to the preset can be saved permanently under a different preset name when desired. Patient identification (name and medical record number) and any other relevant information should be entered into the machine before beginning an exam. This includes date of birth, sex, videotape number, name of person performing the examination, location, and a number of other qualifiers.
The five most common modes used during TEE examinations are 2D gray-scale imaging, color Doppler, pulsed-wave (PW) Doppler, continuous-wave (CW) Doppler, and three-dimensional (3D) imaging. The usual buttons to enable these modes are 2D, Color, PW, CW, and 3D. Other scanning modes, such as M-mode and angio, are often available but are minimally important in comparison. Figure 2–1 is an example of four common ultrasound control panels. While the number and layout of buttons and controls are different, there are many similarities. This chapter focuses on controls that affect 2D imaging, color Doppler, pulsed-wave Doppler, and continuous-wave Doppler. Three-dimensional imaging is a new and exciting addition to TEE, especially for the evaluation of the mitral valve, and will be briefly discussed at the end of this chapter.
Two-dimensional gray-scale imaging is a type of B-mode imaging (B is for brightness) in which the various amplitudes of returning ultrasound signals are displayed in multiple shades of gray. Higher amplitude signals are closer to white, whereas lower amplitude signals are displayed as closer to black. The many different shades of gray form an image or representative picture of the patient’s cardiac anatomy. TEE probes generate a sector or pie-shaped display of gray-scale images, with the top portion of the sector showing the tissue closest to the transducer. Of the five modes, the 2D display mode is most commonly used and manipulated during a TEE examination. Two-dimensional imaging also provides a reference point from which to activate all three forms of Doppler (color, PW, and CW).
Overall gain or amplification is the first postprocessing function performed by the receiver and is the most important variable to adjust during a study. Overall gain controls the degree of amplification that returning signals undergo before display. By increasing gain, small voltages are changed into larger voltages by an operator-specified level of amplification. Gain is also the one control that is misused most often, with the most common mistake being the addition of too much gain to an image. Although additional gain can make the picture brighter and structures more obvious, using too much gain, or over gaining, will destroy image resolution. The appropriate amount of gain for any given image becomes apparent when reflectors and tissue interfaces can be seen, but fluid and blood appear as totally black and echo-free. Myocardium should be adequately dispersed with reflectors by setting it at a medium shade of gray, but the muscle should not approach the look of a solid white band. Only the pericardium, certain states of abnormal thickening, calcification, tissue infiltration, and surgically altered valves should be hyperechoic (very bright). Gain should be added incrementally if the picture is entirely black or if the only structures seen with clarity are normally hyperechoic. Figure 2–2 shows a TEE image with varying gain settings. Figure 2–2A is an example of overall gain setting being too low, while Figure 2–2B is proper gain setting. Figure 2–2C shows the same image with a gain setting that is too high.
The second postprocessing function of the receiver is compensation, commonly known as time gain compensation (TGC) or depth gain compensation (DGC). Compensation makes up for energy loss from the sound beam due to attenuation. Attenuation is the loss of intensity and amplitude of the ultrasound beam as it travels deeper into the body. Strong returning signals from the near field (close to the transducer) need to be suppressed, whereas signals from the far field (deeper depths) require higher amplification.
TGC/DGC is seen on the machine as a column of toggles that can be manipulated along a horizontal plane. By sliding a toggle to the right, the operator increases the gain at that given depth. The TGC/DGC is normally placed at a diagonal slope of variable steepness in which the upper, or near field, toggles are often set at a lower degree of compensation, whereas the lower, or far field, toggles are often set at a higher degree of compensation. A pattern of gradual change from one toggle to the next avoids a “striped” appearance to the ultrasound picture.
Lateral gain compensation (LGC) is present on only some ultrasound machines. LGC toggles are similar to TGC/DGC toggles but act selectively on the y-axis of the picture to change the gain in vertical portions. LGCs help to bring out myocardial walls that may be hypoechoic (lacking in brightness) due to technique or positioning. However, the operator must be selective in using compensation of the gray scale because some sections of the image may not require any compensation.
Compression is another postprocessing function that is just as important as gain. Compression alters the difference between the highest and lowest echo amplitudes received by taking the received amplitudes and fitting them into a gray-scale range that the machine can display. Dynamic range (how many shades of gray appear within the image) is determined by the degree of compression applied to returning signals. Compression changes the dynamic range of the ultrasound signal with an inverse relationship. Increasing the compression produces an image with more shades of gray, whereas decreasing the compression provides a highly contrasted image with strong white and black components. Sharply decreasing the compression may be a tempting method to improve structure delineation, but it will sacrifice low-amplitude targets. Most presets will start the operator with midlevel value of compression and gain. Figure 2–3 shows the effect of compression on an image of a mitral valve. Figure 2–3A shows a high compression setting so the image has too many low amplitude targets. Figure 2–3B presents a medium compression setting. The image has the optimal gray-scale information. Figure 2–3C depicts a low compression setting, so the image has little gray-scale information.
Power can be indirectly assessed by decibel (dB) settings, mechanical index (MI), and thermal index (TI). Power, expressed in watts (W) or milliwatts (mW), describes the rate at which energy is propagated into the imaged medium. Intensity, power per unit area (mW/cm2 or some unit variation), is a concept that closely relates to power. Intensity may not be entirely uniform throughout tissues, so intensity levels predict more accurately the risk of bioeffects than do power levels. When looking at an image, changing the output power from the transducer appears to have an effect similar to that of changing the overall gain; however, alteration of power is less preferable than increasing the overall gain. Any increase in output power can raise the amount of energy that transfers into the biologic tissue, increasing risk of bioeffects. Adjustment of gain settings do not change the amount of energy transferred into the tissue. Changes in overall gain setting adjust signals that have already traveled through tissues. Thus, gain variables should always be adjusted before ever attempting to change the power.
Most machines do not describe the power level in watts or milliwatts. Instead, they indirectly describe power in terms of decibels. The 3-dB rule, intended to help clinicians understand alterations in power, states that an increase of 3 dB doubles the power or intensity from an original value, whereas a decrease of 3 dB halves the power or intensity from an original value. The exact power or intensity levels are not presented on the display, so manufacturers have placed two more variables on the display screen to help clinicians estimate power and intensity levels. The two variables shown on the display are the mechanical index and the thermal index. The MI conveys the likelihood of cavitation resulting from the ultrasonic energy during the examination. Cavitation refers to activity (oscillation or bursting) of microscopic gas bubbles within the tissues due to exposure to ultrasound energy. The TI is the ratio of output power emitted by the transducer to the power needed to raise tissue temperature by 1° Celsius.
Frequency is defined as the number of cycles per second. The frequency of most TEE probes is between 5 and 7 MHz (5,000,000 to 7,000,000 cycles/s). The frequency of the ultrasound probe can have a dramatic effect on image quality of the TEE. Lower transmitted frequencies will create very different images than higher transmitted frequencies. Resolution is the ability to detect two targets positioned closely to one another. When the wavelength is shorter (higher frequencies), the axial resolution is better. Improved resolution generates a more accurate anatomic rendering of structures displayed by ultrasound. However, the lower the sound source frequency, the more effective it is in penetrating tissue and not falling subject to attenuation. Unfortunately, low frequencies yield poorer resolution in their resulting images when compared with high-frequency images. A TEE can be performed at a higher frequency than a chest wall echocardiogram. This is largely due to the fact that the heart is closer to the probe and there is less interference from bone, lung, and other tissue.
The imaging frequency is dependent on the transducer used; however, each probe has a frequency bandwidth. Frequency bandwidth is the range of frequencies any selected probe is able to transmit. Broadband transducers are commonly used because of their ability to transmit over a wide range of frequencies. Some ultrasound systems offer a wide bandwidth and allow an operator to select a part of the bandwidth spectrum that is most appropriate to make the images. During a TEE, the highest possible frequency should be used to distinguish targets on the display. Such a strategy takes advantage of the close proximity of the heart to the TEE probe and will produce more highly resolved images.
Standard transducers transmit and receive frequencies that are the same or within a very close range. The frequency from such a transducer is known as the fundamental frequency. As the beam propagates through tissues, it distorts and creates additional frequencies that are multiples of the fundamental frequency. These additional frequencies are the harmonic frequencies and are created by the tissues themselves. Whereas the fundamental frequency may undergo a large amount of distortion, the harmonic frequencies do not. In patients with poor sound transmission due to obesity or dense muscle tissue, the harmonic frequencies may produce a better image than the fundamental frequency. In general, it should be unnecessary to use the harmonics during a TEE because of the proximity of the transducer to the heart. In fact, when using harmonics, the image likely will have poorer resolution than the image created with the fundamental frequency. During contrast studies, harmonics will improve image quality whether using agitated saline solution or one of the transpulmonary contrast agents. Figure 2–4 demonstrates the differences between a fundamental low frequency (Figure 2–4A), a fundamental high frequency (Figure 2–4B), and a harmonic image (Figure 2–4C). Notice how harmonic imaging makes the aortic valve appear thicker in the harmonic image compared to the fundamental images.
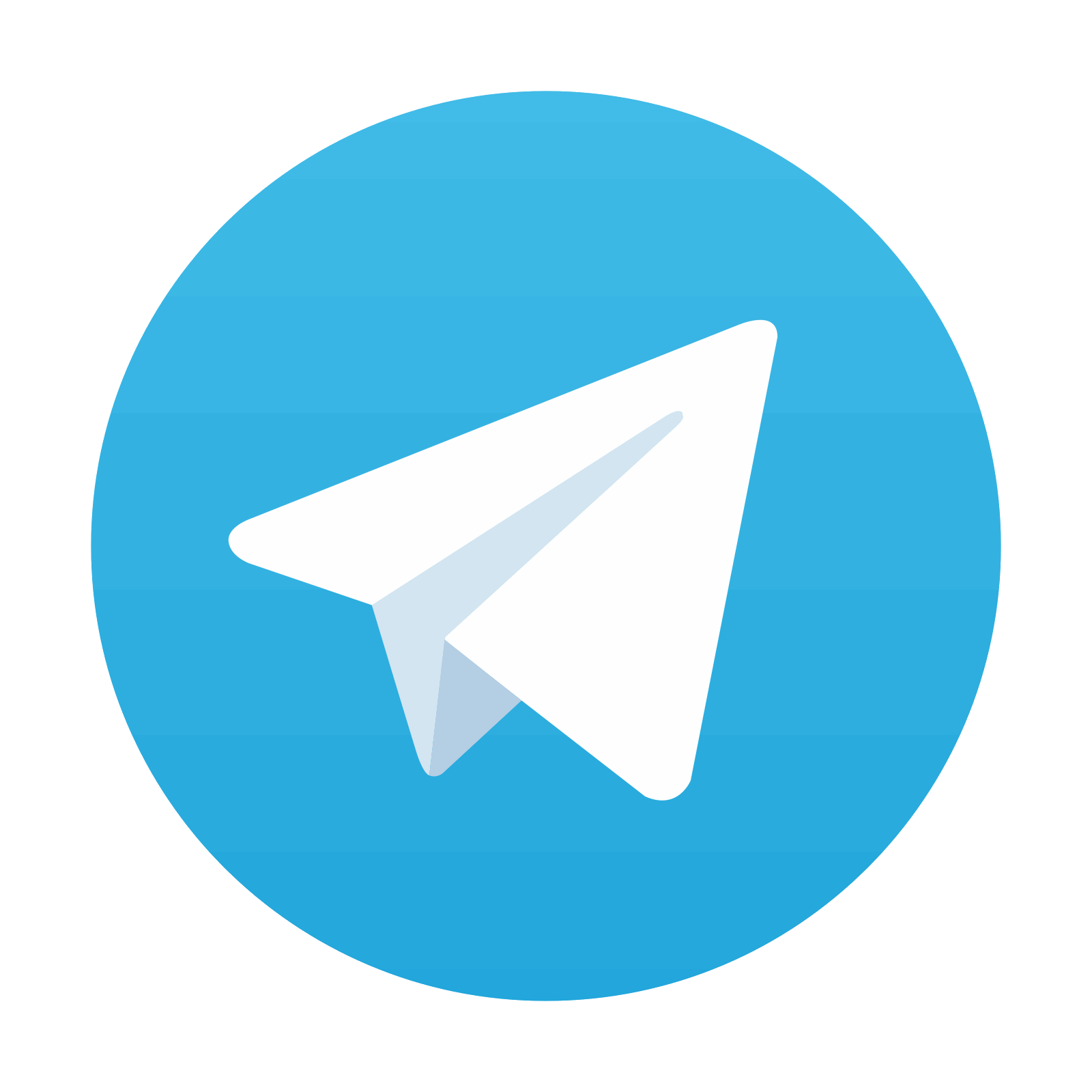
Stay updated, free articles. Join our Telegram channel

Full access? Get Clinical Tree
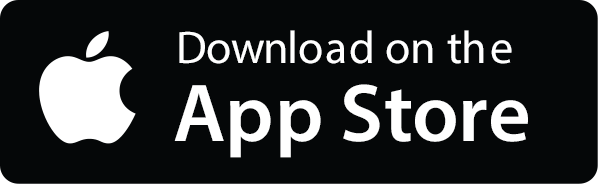
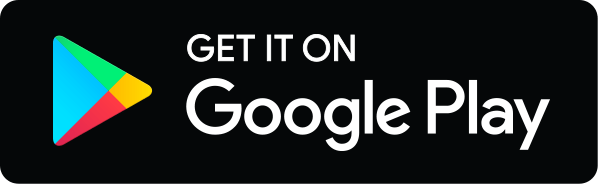