Ultrasound has revolutionized the practice of regional anesthesia, particularly peripheral nerve blockade, and it has also been used for central neuraxial blocks (spinal and epidural injections). 1–3 However, the use of ultrasound for central neuraxial blocks is still in its infancy and not as popular 4 as that for peripheral nerve blocks. The reasons for this are not clear, but may be related to the high success rate of landmark-based techniques, limited data on ultrasound for neuraxial blocks, perceived difficulty in performing spinal sonography, limited acoustic window for ultrasound imaging, and poor understanding of spinal sonoanatomy. However, recently published data suggest that ultrasound is beneficial for central neuraxial blocks. Identification of a given lumbar intervertebral level for central neuraxial block using surface anatomical landmarks (Tuffier line) is often imprecise, 5 and ultrasound is more accurate than clinical assessment. 6 It can also be used to accurately measure the depth to the epidural space or thecal sac 7–9 and predict the ease of performing a neuraxial block. 10 Ultrasound also offers technical advantage by reducing the number of puncture attempts, 11–14 improves the success rate of epidural access on the first attempt, 12 reduces the need to puncture multiple levels, 12–14 and improves patient comfort during the procedure. 13 Ultrasound may also be beneficial for central neuraxial blocks in patients with difficult (ie, abnormal or variant) spinal anatomy. 15,16 Therefore, it is envisioned that the use of ultrasound for central neuraxial blocks will grow in the near future. A sound knowledge of the anatomy of the spine is a prerequisite for understanding the sonoanatomy of the spine. In this chapter, we describe general details of spine anatomy and basic considerations relevant for spinal sonography and central neuraxial blocks.
The human spine or vertebral column is made up of 33 vertebrae—7 cervical, 12 thoracic, 5 lumbar, 5 sacral, and 4 coccygeal—that are stacked on top of each other (Fig. 5–1). A typical vertebra has unique features (Fig. 5–2), and they differ at different levels (Figs. 5–3 to 5–5). The number of spinal nerves in the thoracic, lumbar, and sacral region corresponds to the number of vertebra, each lying below the corresponding vertebra. In the cervical region there are eight spinal nerves. The first seven spinal nerves lie above the corresponding vertebra, but the eighth cervical nerve lies below the seventh cervical vertebra. In the coccygeal region there is only one coccygeal nerve.
The spine has two primary curves (ie, the thoracic and sacral curve) that are concave anteriorly, present at birth, and due to the shape of the vertebral bodies (Fig. 5–1). There are also two secondary curves—the cervical and lumbar curves (Fig. 5–1)—that are convex anteriorly and develop after birth. The cervical curvature develops after the infant starts to support the weight of the head (usually between 4 and 9 months of age), and the lumbar curvature develops between 12 and 18 months of age once the child assumes the upright posture.
A typical vertebra is made up of two components: the vertebral body and the vertebral arch (Fig. 5–6). The latter is formed by the supporting pedicles and laminae (Fig. 5–6). Seven processes arise from the vertebral arch: one spinous process, two transverse processes, two superior articular processes, and two inferior articular processes (Fig. 5–6). Adjacent vertebra articulate with each other at the facet joints between the superior and inferior articular processes and the intervertebral disc between the vertebral bodies (Fig. 5–7). This produces two gaps between the lamina and the spinous processes (ie, the “interlaminar space” and “interspinous space”). It is through these spaces that the ultrasound energy enters the spinal canal and is therefore relevant for spinal sonography and central neuraxial blocks. The three major ligaments of the spine are the ligamentum flavum, anterior longitudinal ligament, and posterior longitudinal ligament (Fig. 5–7). The posterior longitudinal ligament is attached along the length of the anterior wall of the vertebral canal (Figs. 5–7 and 5–8). The ligamentum flavum, also referred to as the “yellow ligament,” is a dense layer of connective tissue that bridges the interlaminar spaces and connects the lamina of adjacent vertebra (Figs. 5-7 to 5–9). It is archlike on cross-section and widest posteriorly in the midline and in the lumbar region. The ligamentum flavum is attached to the anterior surface of the inferior margin of the lamina above, but it splits inferiorly to attach to both the posterior surface (superficial component) and anterior surface (deep component) of the lamina below. The spinous processes are attached at their tips by the supraspinous ligament (Fig. 5–7), which is thick and cordlike, and along their length by the interspinous ligament (Fig. 5–9), which is thin and membranous.
The spinal canal (vertebral canal) is formed by the vertebral arch and the posterior surface of the vertebral body (Fig. 5–6). The openings into the spinal canal are through the intervertebral foramen along its lateral wall and the interlaminar space on its posterolateral wall. Within the spinal canal lies the thecal sac (formed by the dura mater and arachnoid mater) and its contents (spinal cord, cauda equina, and cerebrospinal fluid, Fig. 5–9). The spinal cord extends from the foramen magnum to the conus medullaris, near the lower border of the first lumbar vertebra (Fig. 5–9), finally terminating as the filum terminale. However, there are normal variations in the position of the conus medullaris, and it can extend from T12 to upper third of L3. 17 The cauda equina, named after its resemblance to a “horse’s tail,” is made up of lumbar, sacral, and coccygeal nerves that originate in the conus medullaris and descend caudally to exit the spinal canal through their respective intervertebral foramen. The dural sac ends at the level of the second sacral vertebra (S2) (Fig. 5–9), but can vary from the upper border of S1 to the lower border of S4. 18 The epidural space is an anatomical space within the spinal canal, but outside the dura mater (extradural). It extends from the level of the foramen magnum cranially to the tip of the sacrum at the sacrococcygeal ligament (Fig. 5–9). The posterior epidural space is of importance for central neuraxial blocks. The only structure of importance in the anterior epidural space for neuraxial blocks is the internal vertebral venous plexus.
Spinal sonography typically requires the use of low-frequency ultrasound (5-2 MHz) and a curved array transducer because the spine is located at a depth. Low-frequency ultrasound provides good tissue penetration, but it lacks spatial resolution at the depths at which neuraxial structures are imaged (approximately 5–8 cm). The osseous framework of the spine, which envelopes the neuraxial structures, also reflects much of the incident ultrasound signal. Furthermore, the acoustic window for ultrasound imaging (interlaminar and interspinous space) is relatively narrow, and this poses an additional challenge in obtaining high-quality images of the neuraxis. Recent improvements in ultrasound technology, image processing capabilities of ultrasound machines, availability of advanced imaging modalities (tissue harmonic imaging [THI], tissue aberration correction, color B-mode imaging), and the development of new ultrasound scan protocols 1 have all contributed to improving our ability to image the neuraxis. Currently it is possible to accurately delineate the neuraxial anatomy relevant for central neuraxial blocks using ultrasound. 1,3 Also of note is technology that was once only available in the high-end cart-based ultrasound systems are now available in portable ultrasound devices, making them practical for spinal sonography and ultrasound-guided neuraxial blocks.
There are basically three anatomical planes: median, transverse, and coronal plane (Fig. 5–10). The median plane is a longitudinal plane that passes through the midline and bisects the body into equal right and left halves. The sagittal plane is also a longitudinal plane but is parallel to the median plane and perpendicular to the ground. Therefore, the median plane can also be defined as the sagittal plane that is exactly in the middle of the body (median sagittal plane). The transverse plane, also known as the axial or horizontal plane, is parallel to the ground. The coronal plane, also known as the frontal plane, is perpendicular to the ground.
The spine can be imaged using ultrasound in the transverse (transverse scan, Fig. 5–11) or longitudinal (sagittal scan, Fig. 5–12) plane and with the patient in the sitting, lateral decubitus, or prone position. The latter is useful in patients presenting for chronic pain interventions when fluoroscopy may also be used in conjunction with ultrasound. The transverse and sagittal scan planes complement each other during an ultrasound examination of the spine. Coronal plane images are displayed exclusively during multiplanar three-dimensional (3-D) ultrasound imaging, and they are rendered images from the acquired 3-D volume. During a transverse scan of the lumbar spine, the ultrasound beam can be insonated at the level of the spinous process (transverse spinous process view, TSPV, Fig. 5–11A) or through the interspinous space (transverse interspinous view, TISV, Fig. 5–11B). A sagittal scan can be performed through the midline (median sagittal scan) or through a paramedian (paramedian sagittal scan, PMSS) plane. The latter is more frequently used (less bone), and during a paramedian sagittal scan (PMSS) the ultrasound beam is insonated lateral to the midline (paramedian), and ultrasound images are acquired from the level of the lamina (paramedian sagittal lamina view, Fig. 5–12A), articular process (paramedian sagittal articular process view, Fig. 5–12B), or transverse process (paramedian sagittal transverse process view, Fig. 5–12C). The neuraxial structures are better visualized through a paramedian sagittal plane than through the median sagittal or median transverse plane. 19 The ultrasound visibility of neuraxial structures is further improved when the spine is imaged in the paramedian sagittal oblique axis (Fig. 5–13). During a paramedian sagittal oblique scan (PMSOS), the transducer is positioned 2 to 3 cm lateral to the midline (paramedian) in the sagittal plane, and it is also tilted slightly medially, that is, towards the midline (Fig. 5–14). The purpose of the medial tilt is to ensure that the majority of the ultrasound energy (signal) enters the spinal canal through the widest part of the interlaminar space. The same applies, and is probably more important, during a paramedian sagittal scan of the thoracic spine (Fig. 5–15).
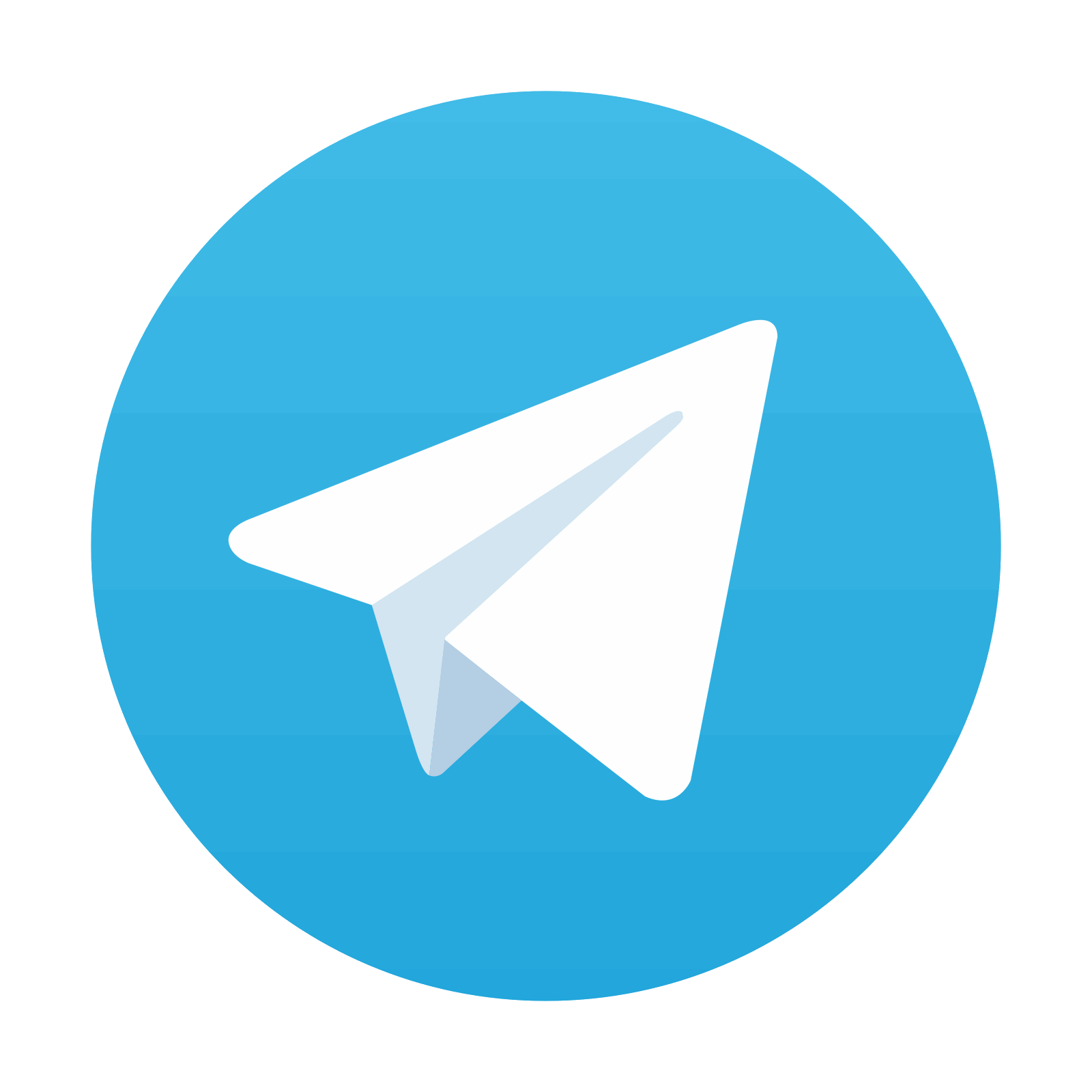
Stay updated, free articles. Join our Telegram channel

Full access? Get Clinical Tree
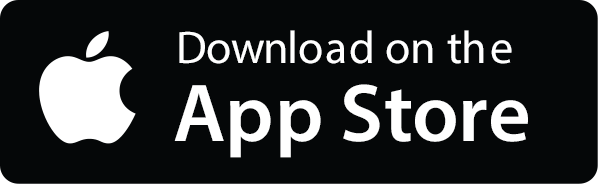
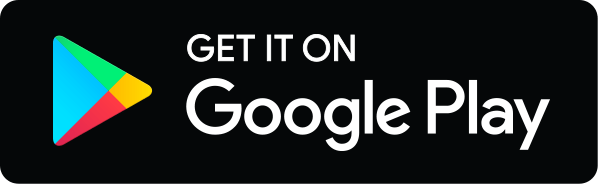