Chapter Outline
Technology 207
An Overview of Ultrasound 207
Ultrasound and Its Interaction With Tissue 207
Ultrasound in Tissue 207
Creating an Ultrasound Picture 209
Limitations of Ultrasound 211
Ultrasound for Regional Anesthesia and Vascular Access 212
Technological advances over the last decade, including improvements in image resolution, and the development of smaller, more manageable, laptop-sized systems have made ultrasound techniques more accessible for all medical practitioners.
Ultrasound allows immediate and dynamic visualization of anatomy, and it is an invaluable diagnostic used in prenatal screening, assessment of cardiac function, and assessment of intraabdominal and vascular pathology.
Ultrasound also has a role in facilitating performance of invasive procedures. In the field of anesthesiology, this predominantly means insertion of central venous lines and placement of neural blockade. Adopting ultrasound to guide procedures can improve safety and success rate and decrease performance time.
Technology
An Overview of Ultrasound
Sound is transferred by mechanical waves of compression and rarefaction through a medium. Audible sound has frequencies between 12 Hz and 20,000 Hz, sound with frequencies greater than 20,000 Hz is known as ultrasound. Ultrasound used in medical imaging applications typically has a frequency in the megahertz range.
Ultrasound is produced by piezoelectric crystals. Piezoelectricity is the ability of a material to generate an electric potential after application of a mechanical stress. The piezoelectric effect also occurs in reverse; application of an electric potential to a crystal will cause mechanical deformation. Both the direct and reverse piezoelectric effects are used in medical ultrasonography.
The basic premise of ultrasonography is that sound waves travel at different speeds in tissues of different density and that sound waves are reflected at tissue boundaries. A short pulse of ultrasound created by a piezoelectric crystal can be conducted through tissues of interest ( Figure 15-1 ). Ultrasound will reflect back from boundaries between tissues and cause deformation of the piezoelectric crystal, which via the direct piezoelectric effect will cause a potential difference to occur in the crystal. The potential difference in the crystal induces a current that is measured. The time gap between impulse generation and returning echoes is carefully measured. Making assumptions about the speed of ultrasound in tissue (on average 1540 m/sec) allows time gaps to be interpreted as distances. Complex postprocessing allows these data to be converted to images.

Ultrasound and Its Interaction with Tissue
To produce a single scan line an ultrasound machine passes a defined current through a piezoelectric crystal to produce a defined pulse of ultrasound. A pulse of ultrasound will typically be 1 to 2 cycles with a frequency of 2 to 15 MHz. The physical length of the pulse is determined by multiplying the number of cycles by the wavelength and is known as the spatial pulse length (SPL).
Once the pulse of ultrasound has been created and conducted into the tissue of interest, the transducer will wait a defined period of time to receive echoes. The amount of time the transducer waits is determined by the depth of field required to visualise the anatomical structure of interest and the average speed of sound in tissue. After the specified period of time, the next pulse of ultrasound is produced.
The time between pulses is known as the pulse repetition period (PRP), and its inverse is the pulse repetition frequency (PRf). This is distinct from the frequency of the ultrasound itself.
Ultrasound in Tissue
The speed at which ultrasound travels through tissue is determined by the compressibility and density of the tissue. It averages 1540 m/sec ( Table 15-1 ).
Speed of sound in tissue ( c ) = 1 / compressibility × density
Material | Speed of Transmission (m/s) | Acoustic Impedance (kg/m 2 /s × 10 6) | Attenuation Coefficient (dB/cm at 1 MHz) |
---|---|---|---|
Water | 1484 | 1.5 | 0.002 |
Blood | 4080 | 1.62 | 0.18 |
Fat | 1450 | 1.35 | 0.63 |
Lung | 550 | 0.26 | 41 |
Muscle | 1580 | 1.65-1.74 | 1.3-3.3 |
Bone | 4080 | 7.8 | 20.0 |
Air | 330 | 0.0004 | 3.3 × 10 6 |
The property of a tissue that determines the speed of ultrasound is known as acoustic impedance (z).
Acoustic Impedance ( z ) = Density ( ϱ ) × Acoustic Velocity ( V )
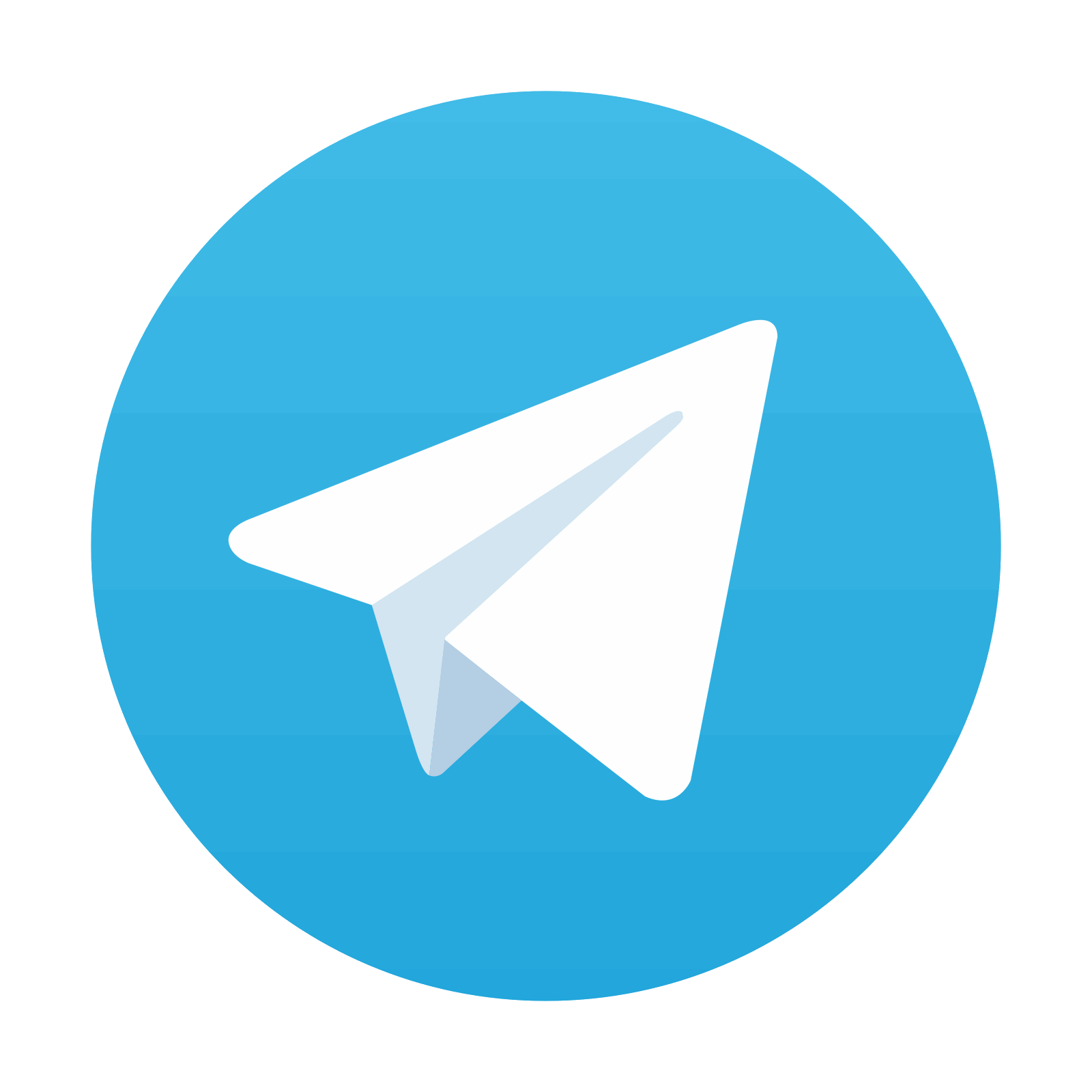
Stay updated, free articles. Join our Telegram channel

Full access? Get Clinical Tree
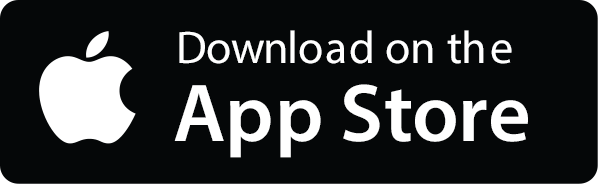
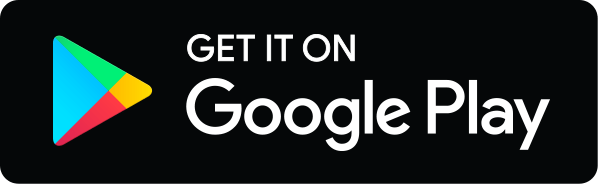