Fig. 2.1
Intravenous recombinant tissue plasminogen activator (rtPA) for acute ischemic stroke. This 58-year-old man presented to the emergency department 3.5 h after the acute onset left hemiplegia. (a) Initial computed tomography (CT) scan was interpreted as having no hemorrhage and evidence of early right middle cerebral artery (MCA) distribution ischemic changes. Intravenous rtPA was administered without neurologic improvement. (b) Eight hours after administration of rtPA the patient had a decreased level of consciousness and underwent repeat CT scan demonstrating early hemorrhage in the putamen ipsilateral to the stroke. (c) CT angiography obtained at that revealed a patent right M2 branch with absent flow in the lenticulostriate arteries on the right side (asterisk). (d) Twenty-four hours after presentation, a plain CT was obtained that demonstrated extension of hemorrhage and increased stroke and peri-hematoma edema resulting in midline shift
Given the short window in which rtPA therapy is initiated, there are a limited number of cases that meet the inclusion criteria for thrombolytic therapy. The number of cases that are eligible for rtPA therapy is further diminished by contraindications as rtPA cannot be used in cases with evidence of intracranial hemorrhage, clinical evidence of subarachnoid hemorrhage (SAH), active internal bleeding, known bleeding diathesis with elevated prothrombin time or international normalized ratio (INR), intracranial surgery within 3 months of presentation, uncontrolled hypertension (systolic >185 mmHg, diastolic >110 mmHg), history of intracranial hemorrhage, and/or known aneurysm or arteriovenous malformation.
The use of rtPA between 3 and 4.5 h after stroke onset was studied by the European Cooperative Acute Stroke Study using the same dosing regimen as in the NINDS trial [22]. This trial demonstrated a modest but significant improvement in functional outcomes with a significant associated increase in symptomatic intracranial hemorrhage in rtPA-treated patients; however, there was no difference in mortality between treatment groups. This evidence supports the extension of the therapeutic window to initiate intravenous thrombolytic therapy to 4.5 h following stroke onset.
Other promising pharmacological thrombolytic therapies are currently being explored for stroke thombolysis. Desmoteplase is a fibrin-specific thrombolytic agent based on the anti-hemostatic component of the saliva of the vampire bat. The Desmoteplase in Acute Stroke trial part 2 (DIAS-2) utilized this thrombolytic agent in patients between 3 and 9 h following stroke onset with a demonstrated ischemic penumbra depicted by a diffusion–perfusion-weighted imaging mismatch on MRI [23]. This study demonstrated neither an improvement in functional status nor an increase in treatment-related hemorrhage or mortality; although there was an increase in delayed and non-neurological deaths in the treatment arm. Desmoteplase will be further studied in two subsequent trials (DIAS-3 and DIAS-4) initiated in 2009. Tenecteplase is a promising thrombolytic agent based on rtPA with modifications in the rtPA amino acid sequence that increase fibrin selectivity, increase half-life, and decrease the risk of hemorrhage [24]. Tenecteplase has undergone early investigation in safety and dose finding studies and is presently being evaluated in a Phase II trial comparing it against alteplase (rtPA) for acute ischemic stroke administered within 4.5 h of stroke onset (ATTEST trial, NCT01472926) [25].
Intra-arterial Thrombolysis and Mechanical Thrombolysis/Thrombectomy
Intra-arterial thrombolysis is delivered through an endovascular catheter proximal to the site of arterial occlusion or from within the thrombus (Fig. 2.2). The main advantage of intra-arterial thrombolysis is that an elevated dose of thrombolytic agent can be delivered at the site of occlusion while reducing the systemic dose of drug compared to intravenous therapy. Intra-arterial therapy was effective when delivered up to 6 h following stroke onset, hence it may be utilized in patients who present outside of the 4.5-h window for intravenous rtPA therapy. However, this technique requires an interventional neuroradiology team to place the catheter in the occluded arterial branch prior to thrombolysis, potentially delaying therapy onset. No trial has directly compared intravenous and intra-arterial therapy to date.
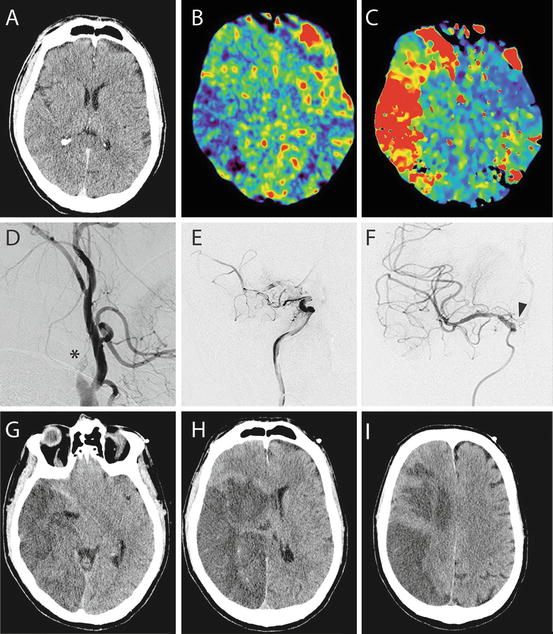
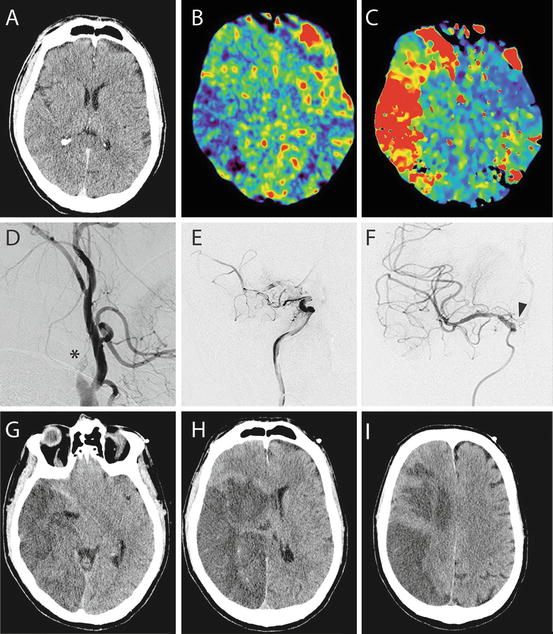
Fig. 2.2
Intra-arterial and mechanical thrombolysis for acute stroke. This 36-year-old female presented 4 h after acute onset arm and facial weakness with dysarthria. (a) Initial CT scan demonstrated early ischemic changes in the right MCA distribution. (b, c) CT perfusion demonstrated decreased cerebral blood flow (b) and elevated time to peak concentration (c) in the right MCA distribution. The patient received 0.6 mg/kg of rtPA and was taken to angiography. (d) Angiogram revealed an occlusion of the right internal carotid artery (asterisk). (e) A Penumbra device was used to aspirate clot from the internal carotid artery and achieve reperfusion. (f) Intra-arterial rtPA was infused through a microcatheter (catheter tip at black arrow) to achieve recanalization of the MCA. (g–i) The patient did not have neurological recovery following recanalization and went on to have a completed stroke in the right MCA distribution as demonstrated by hypodensity on plain CT 24 h after rtPA. Early uncal herniation (g) and midline shift (h) were evident
The evidence supporting intra-arterial therapy includes two Phase III randomized controlled trials, the North American Prolyse in Acute Cerebral Thromboembolism study part II (PROACT-II) and the Japanese Middle Cerebral Artery Embolism Local Fibrinolytic Intervention Trial (MELT) [26, 27]. PROACT-II utilized 9 mg of recombinant pro-urokinase or heparin (control group) given as an intra-arterial infusion over 2 h in cases of middle cerebral artery (MCA) occlusion presenting less than 6 h following stroke onset [26]. In this study, 40 % of treatment patients reached a modified Rankin score (mRS) of <2 % vs. 25 % of control patients. Recanalization rates were 66 % in the treatment group vs. 18 % in controls. Symptomatic intracranial hemorrhage was higher in the treatment group at 10 % vs. 2 % for controls; however, mortality was equal in the two groups. The MELT trial utilized urokinase delivered in bolus doses every 5 min until recanalization was achieved in cases of MCA occlusion presenting within 6 h of stroke onset [27]. The control arm of the MELT trial received standard medical therapy that did not include intravenous rtPA as it was not approved in Japan during the trial. The MELT trial was halted early when intravenous rtPA was approved for use in acute ischemic stroke in Japan. The subsequent analysis of the truncated dataset was promising but did not reach significance for the primary endpoint, mRS 0–2 at 90 days, where 49 % of the treatment arm reached this endpoint vs. 39 % in the control group. However, secondary analyses were significantly improved including excellent outcome (mRS 0–1 at 90 days) achieved in 42 % of treatment patients vs. 23 % of controls and a National Institutes of Health Stroke Scale (NIHSS) score of 0–1 at 90 days was 5.3 % in the treatment arm and 3.5 % in the control arm (P = 0.017). Rates of intracerebral hemorrhage (ICH) were 9 % in treatment patients and 2 % in controls.
No thrombolytic therapy has received regulatory approval for intra-arterial use in the United States. Therefore, the use of intra-arterial thrombolytics is considered off label [28]. Furthermore, pro-urokinase is not clinically available in the United States and urokinase has not been approved for use in acute ischemic stroke, leaving rtPA as the thrombolytic of choice for intra-arterial thrombolysis in most centers. The American College of Chest Physicians has included intra-arterial rtPA as an option for acute ischemic stroke <6 h in an MCA distribution or in the basilar distribution in centers with expertise in endovascular therapy [28].
Combination therapy with intravenous rtPA followed by intra-arterial thrombolysis is a strategy that provides the benefit of early intravenous thrombolytic dosing followed by intra-arterial administration of thrombolytic drug directly to the thrombus [29]. This strategy has been studied in the Interventional Management of Stroke (IMS) II trial comparing reduced dose (0.6 mg/kg) rtPA intravenous followed by intra-arterial rtPA (maximum dose 22 mg) to historical controls from the NINDS study [30]. IMS II patients had a mortality of 16 % vs. 24 % in the NINDS placebo arm. Furthermore, all functional measures were improved in IMS II patients compared to NINDS placebo patients and the rate of intracerebral hematoma was not different between the IMS II cohort and the intravenous rtPA arm of NINDS. Combination strategies utilizing various dosing of intravenous and intra-arterial thrombolytics have been reported in noncontrolled studies with promising results [31–34]. A Phase III trial (IMS III) comparing standard intravenous rtPA treatment vs. reduced dose intravenous rtPA followed by intra-arterial rtPA for patients presenting within 3 h of stroke onset is ongoing (NCT00359424, Clinicaltrials.gov).
Mechanical thrombolysis and embolectomy are strategies that employ an intra-arterial device to either physically break down or secure and retrieve a clot from the occluded artery (Figs. 2.2 and 2.3) [35, 36]. These therapies may be combined with stenting to maintain recanalization after embolectomy [37]. Generally, these therapies are combined with intravenous and/or intra-arterial thrombolytics if the stroke presents within 4.5 h of onset or without pharmacologic thrombolysis in later presentations. The techniques employed in mechanical thrombolysis include clot disruption with an endovascular wire or sonothrombolysis where ultrasound pulses are delivered with thrombolytics at the tip of the catheter [38]. Embolectomy can be achieved with wire loops to capture emboli, retrievable stent strategies (Fig. 2.3e) or aspiration catheters that suction clot from the vessel (Fig. 2.2e) [38]. Devices to achieve vessel recanalization have largely been approved based on safety and recanalization results in large animal models and in small human studies, limiting functional outcome measures in support of these strategies. The Mechanical Embolectomy in Acute Ischemic Stroke (MERCI) trial is the main evidence supporting mechanical clot retrieval [39, 40]. The MERCI device is an intra-arterial coiled wire that is “corkscrewed” around or into the clot to trap it and then used to physically retrieve the clot by pulling it from the parent artery. In the MERCI trial patients presenting within 3 h of stroke onset in any vascular distribution were given intravenous rtPA at standard doses. If the occlusion did not resolve on follow-up angiography, then the MERCI device was utilized. The device resulted in a 57 % recanalization rate that increased to 70 % with additional intra-arterial rtPA. An mRS outcome of 0–2 was achieved in 36 % of patients, the mortality rate was 34 % and the risk of procedure-related morbidity was 5.5 %. In summary, mechanical means of recanalization show promise in early clinical trials and warrant further study.
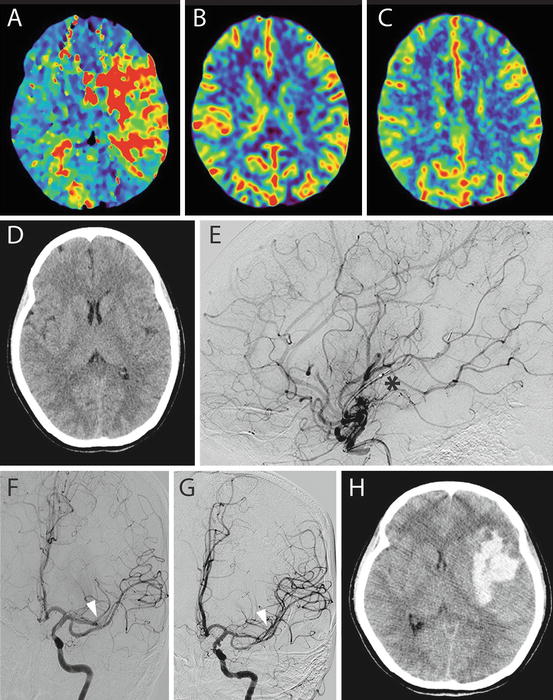
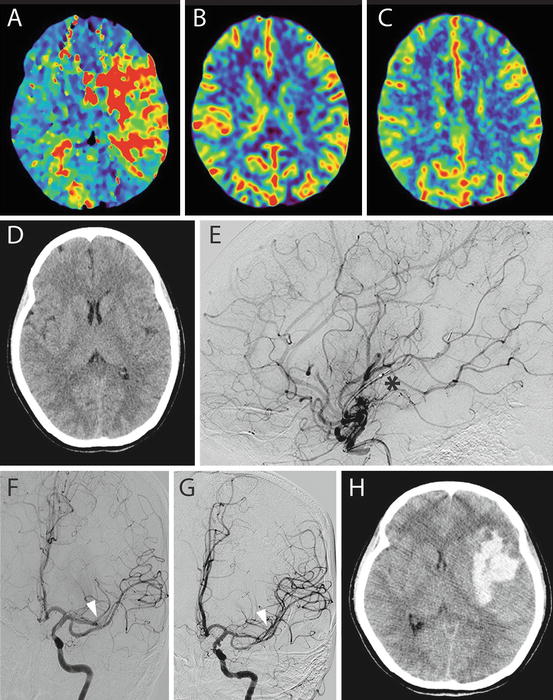
Fig. 2.3
This 42-year-old patient presented 3 h after acute onset aphasia and right arm and leg hemiparesis. (a–c) Early CT perfusion demonstrated an elevated time to peak concentration (a) with minimal deficit in cerebral blood flow (b) or cerebral blood volume (c) suggesting good collateralization in the MCA territory. (d) Plain CT demonstrates early stroke in left insular cortex. The patient received 0.6 mg/kg of rtPA and was taken to the angiography suite. (e) A retrievable stent was deployed in the occluded M2 branch of the MCA (asterisk) and clot was retrieved. (f) Angiogram prior to stent retrieval shows M2 occlusion (arrow). (g) Angiogram following clot retrieval shows recanalization of the occluded M2 branch (arrow). (h) Nine hours after the procedure the patient declined with worsening weakness and decreased level of consciousness and was found to have an intracerebral hematoma in the distribution of the stroke. The patient went on to surgery to evacuate this clot without major clinical improvements postoperatively
In vascular distributions other than the MCA, there is less evidence to guide the use of intra-arterial thrombolytic therapy and/or mechanical thrombolysis. In multiple case series of intra-arterial therapy for basilar artery occlusion, a potentially positive effect has been demonstrated when recanalization is achieved [41–44]. Stent-assisted recanalization of basilar occlusion has been reviewed in case series and has been shown to achieve improved recanalization rates with an acceptable risk profile up to 8 h after stroke onset [45, 46]. Randomized controlled trials for basilar occlusion might clarify the role for these treatments in this indication; however, such a trial would be difficult to execute given the poor outcomes experienced by these patients, the relatively infrequent presentation of these cases, and the lack of a standard therapy to act as a control.
Decompressive Craniectomy
In cases where reperfusion fails to reverse the evolution of stroke or where stroke progresses despite recanalization, there is a risk for malignant edema and elevated intracranial pressure (ICP) that may be life threatening (Fig. 2.4). Mortality in this cohort of patients has been as high as 78 % [47]. In severe cases there is a role for decompressive craniectomy to relieve brain shift, herniation, elevated ICP, and death. For malignant MCA stroke, the procedure generally includes a large craniectomy, durotomy, and subtemporal decompression (Fig. 2.4e, f). Results for decompressive craniectomy in case series and randomized trials demonstrate a significant decrease in mortality after this procedure [48, 49]. Patient age is the only definitive predictive factor of outcome after decompressive hemicraniectomy for stroke, where younger patients have better functional outcomes [50]. Other factors including timing of surgery, side of stroke, and the presence of herniation have not proven to be significant predictors of outcome. A potential downside of decompressive craniectomy for stroke is that the procedure saves lives but leaves patients in severely disabled or persistent vegetative states [51, 52]. Both the benefits and potential harms of this procedure must be considered carefully in the context of a specific case before treatment is undertaken.
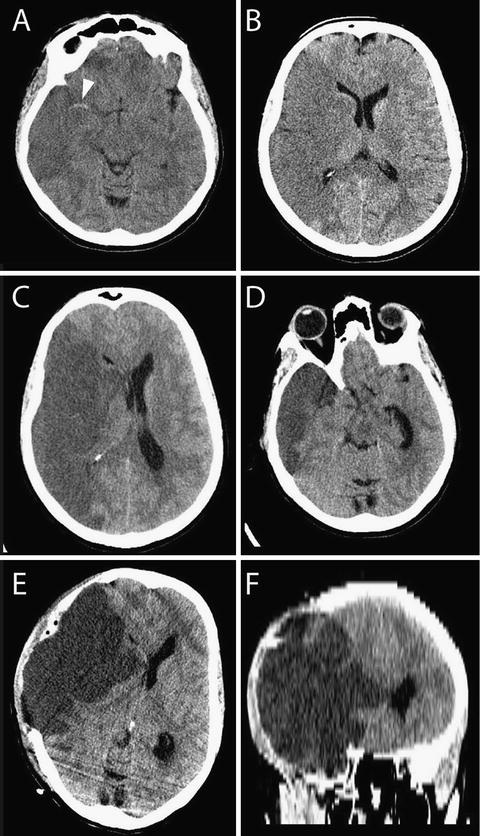
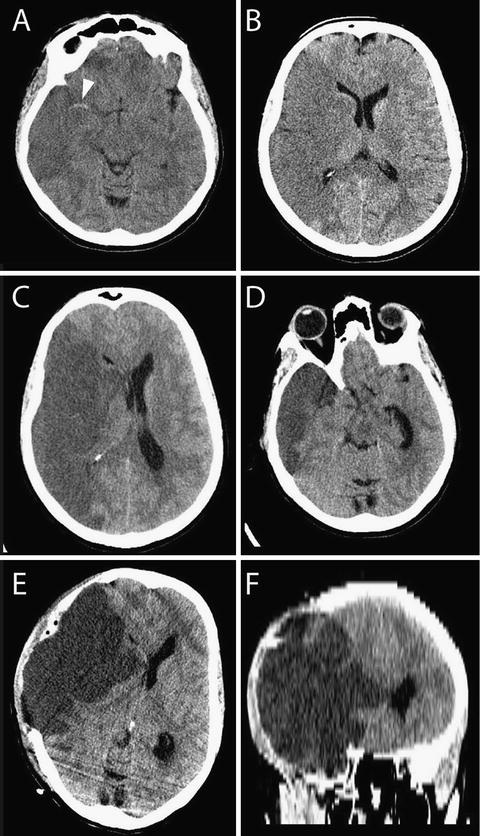
Fig. 2.4
Decompressive craniectomy for malignant MCA stroke. This 48-year-old female experienced left hemiparesis following a cardiac procedure on cardiorespiratory bypass. (a) A hyperdense right MCA sign was observed (arrow) with MCA stroke. (b) The entire right MCA distribution was involved with diffuse hypodensity. The next day the patient had an acute loss of consciousness with a Cushing’s response and dilated right pupil. (c, d) Urgent CT scan revealed malignant edema with midline shift (c) and uncal herniation (d). (e, f) Emergent decompressive craniectomy, subtemporal decompression, and duroplasty were undertaken to allow external herniation of edematous brain
In the case of cerebellar infarction, there is a risk of direct brainstem compression and hydrocephalus if swelling occurs (Fig. 2.5) [53]. Suboccipital craniectomy, in addition to expansion duroplasty, can be used to accommodate cerebellar swelling. In severe cases of cerebellar edema, infarcted tissue can be excised to provide more room for swelling (Fig. 2.5e, f). Early craniectomy in these cases may be justified to prevent impending herniation or brainstem compression.
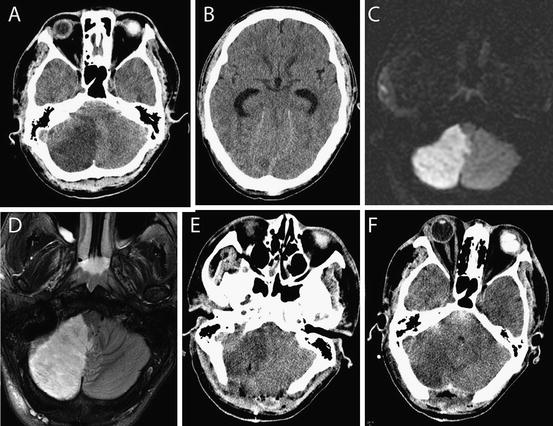
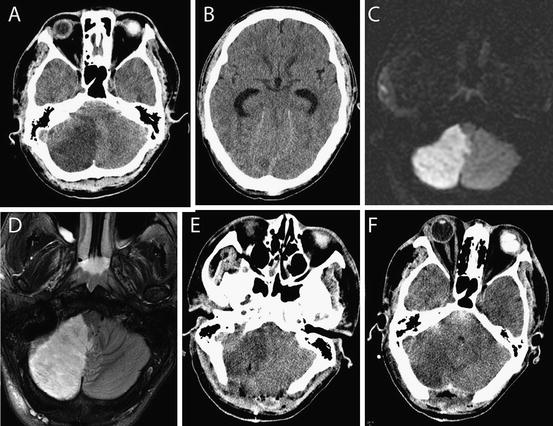
Fig. 2.5
Cerebellar stroke may lead to edema and obstructive hydrocephalus. This 27-year-old man with a history of an intracardiac arteriovenous shunt presented with a 4-h history of headache, nausea, and vomiting and decreased level of consciousness. He presented in extremis with bilateral fixed pupils and no motor response. (a, b) A posterior inferior cerebellar artery infarct was diagnosed with complete obliteration of the fourth ventricle (a) and acute hydrocephalus (b). (c, d) An emergent extraventricular drain was inserted followed by magnetic resonance imaging (MRI) diffusion-weighted imaging (c) and T2 imaging (d) that confirmed a completed stroke as hyperintensity in the vascular distribution. (e, f) The patient was taken for posterior fossa decompression and expansion duroplasty. Edematous, infracted tissue was resected from the cerebellum during the procedure to facilitate closure. The fourth ventricle was patent after the case (f). The patient went on to make a full neurological recovery
Treatment of Hemorrhagic Stroke
Hemorrhagic stroke is broadly organized into ICH that involves bleeding into the parenchyma of the brain (Fig. 2.6), SAH that involves bleeding in the subarachnoid space from a ruptured vessel and intraventricular hemorrhage (IVH) where bleeding occurs into the ventricular system. All three can be caused by trauma, but spontaneous cases are classified as strokes. In all three cases the acute management relates to the avoidance of secondary deterioration to preserve neurological function remaining after the primary injury. The common etiologies of spontaneous ICH include hypertensive hemorrhages, amyloid angiopathy, coagulopathy or anticoagulation therapy-related hemorrhages, bleeding into ischemic stroke, bleeding from ruptured vascular malformations, and bleeding from tumors. As discussed in the introduction there is no convincing evidence for an ischemic penumbra surrounding an ICH, although there may be a metabolic penumbra that promotes cell death in the subacute phase [54]. Generally, secondary deterioration in ICH relates to enlargement of the clot and compression of adjacent structures. Therefore the central tenet of treatment of ICH is prevention of clot expansion and treatment of the underlying source of bleeding and/or decompression of the hematoma in cases where ICP is elevated.
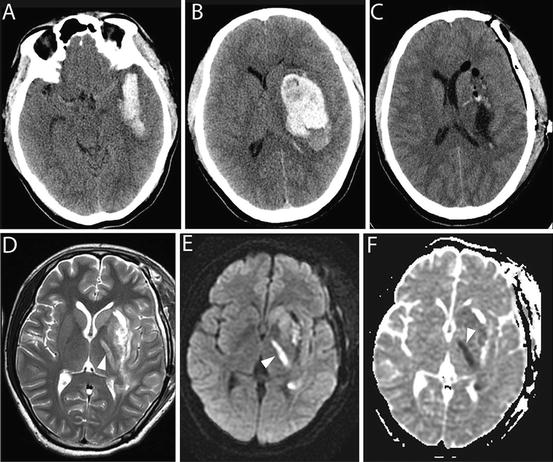
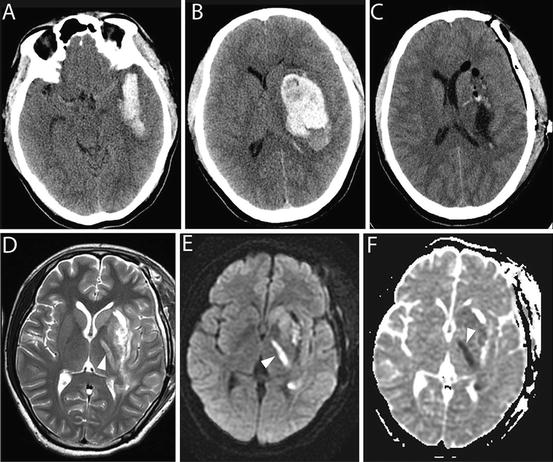
Fig. 2.6
Intracerebral hemorrhage requiring surgical evacuation. This 37-year-old male presented with acute onset headache, nausea and vomiting, aphasia, and progressive right hemiparesis. (a, b) CT revealed a left putamenal hemorrhage with heterogeneous densities of blood. The hematoma was found to come within 1 cm of the cortical surface (a) and was deemed a surgical lesion. (c) Emergent craniotomy was performed and the entire hematoma was removed. (d–f) The patient had no clinical improvement following surgery. Delayed MRI revealed persistent edema in tissue adjacent to the hematoma cavity with hyperintensity in the posterior limb of the internal capsule (arrow). Diffusion-weighted imaging (e) and apparent diffusion coefficient map (f) confirmed the stroke (arrow (e) and (f))
Spontaneous SAH is generally related to ruptured brain aneurysms (about 85 % of cases), a group of idiopathic cases (about two-thirds being benign perimesencephalic SAH) and occasionally from arteriovenous or other brain vascular malformations. In aneurysmal SAH, there is a risk of re-hemorrhage with clot expansion and a risk of delayed cerebral ischemia during which time there is an increased risk of stroke [55]. Hence, treatment of SAH involves rapid diagnosis and treatment of the source of bleeding and treatment of aneurysmal SAH involves prevention and treatment of angiographic vasospasm, which is the main cause of delayed cerebral ischemia.
IVH is generally related to a preexisting ICH or SAH with extension into the ventricles and is associated with worse outcome in the case of ICH [56]. In IVH, deterioration relates to obstructive or communicating hydrocephalus and elevated ICP necessitating cerebral spinal fluid diversion [57]. The rest of the chapter will focus on the treatment of ICH.
Acute Medical Management
Principles of acute medical management for ICH are similar to those discussed for acute ischemic stroke with the exception of major differences in blood pressure management and the addition of managing anticoagulant reversal in the acute phase. Evidence-based guidelines for the management of ICH have been assembled and endorsed by the AHA and collaborating groups [58].
Acute blood pressure management is important in avoiding early re-hemorrhage in hypertensive ICH. The AHA guidelines recommend reduction of the blood pressure as follows: aggressive reduction of blood pressure if systolic >200 mmHg or mean arterial pressure >150 mmHg, reducing blood pressure to target a cerebral perfusion pressure of >60–80 mmHg in cases where systolic pressure is >180 mmHg or mean arterial pressure >130 mmHg and there is evidence of elevated ICP, and reduce blood pressure modestly to systolic ~160 mmHg and mean arterial pressure ~110 mmHg in cases where systolic pressure is >180 mmHg or mean arterial pressure is >130 mmHg and there is no evidence of raised ICP. There are several intravenous, oral, and transdermal agents that can be used for acute lowering of blood pressure including beta-blockers like labetalol or metoprolol, calcium channel blockers like nicardipine, and smooth muscle relaxants like hydralazine or nitrates.
For patients presenting with anticoagulant or fibrinolytic therapy-related hemorrhage, urgent reversal of the underlying therapy is necessary to prevent rebleeding. Patients treated with warfarin are deficient in vitamin K dependent clotting factors, therefore urgent replacement of these factors is required to reverse the elevated INR associated with warfarin. Vitamin K can be administered with fresh frozen plasma or with one of several different prothrombin complex concentrate products [59]. Patients on heparin receive protamine reversal. Antiplatelet therapy is associated with worsened outcomes following ICH [60]. Replacement of platelets does not seem to change outcome in these patients [60]. However, platelet replacement may be considered before, during, and after surgical procedures to avoid bleeding in these cases. Patients who have undergone fibrinolysis and subsequently suffer an ICH are at an elevated risk for mortality and poor neurologic outcome. Replacement of deficient coagulation factors is recommended in these cases but there is little evidence to guide management. Administration of recombinant factor VIIa is no longer recommended for the treatment of ICH due to a lack of improvement in functional outcome and an elevated risk of thromboembolic complications in the randomized controlled trial in ICH [61].
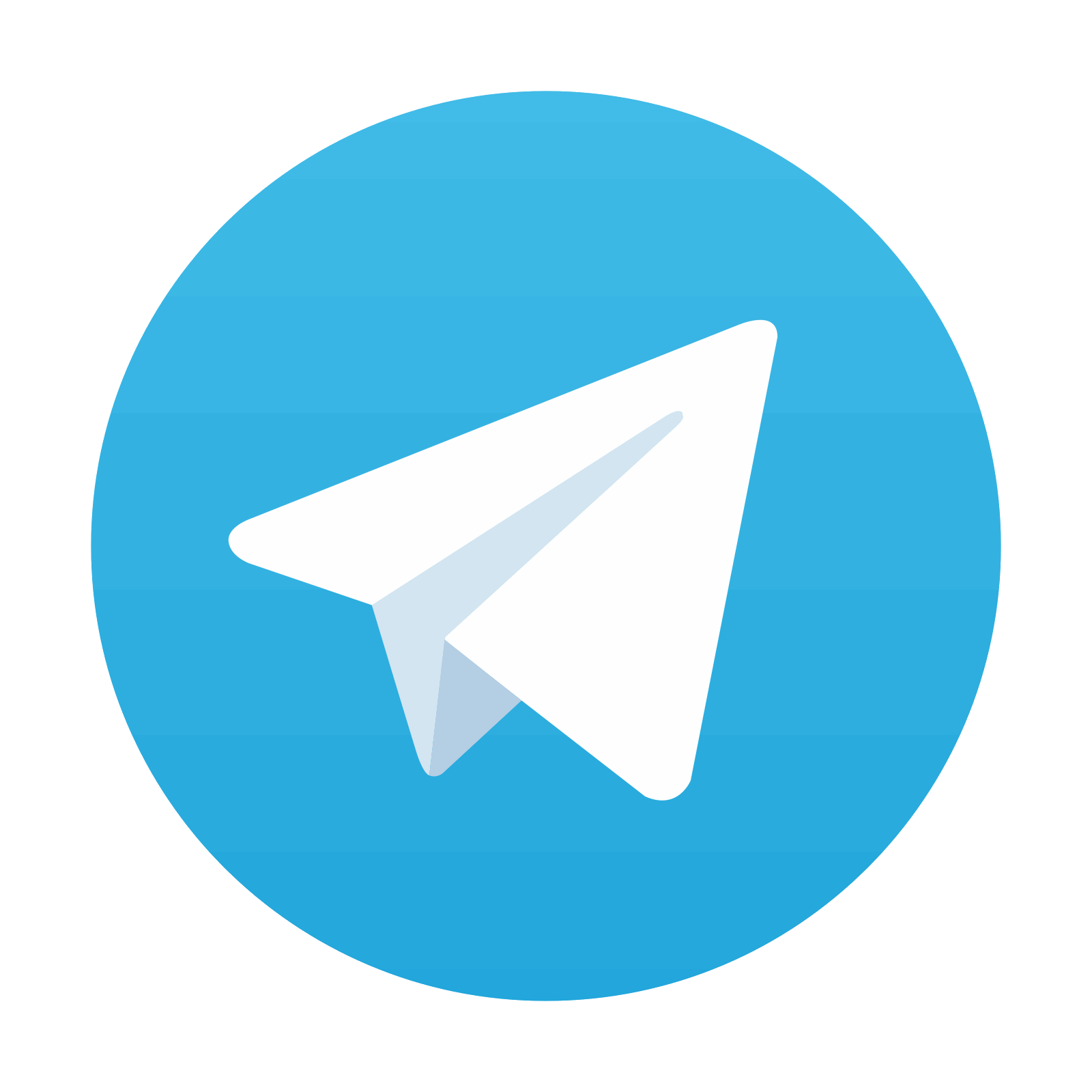
Stay updated, free articles. Join our Telegram channel

Full access? Get Clinical Tree
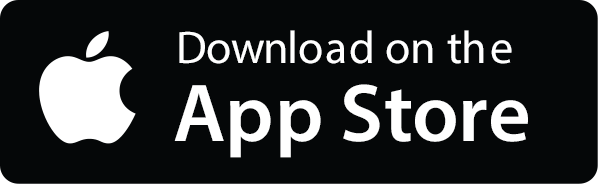
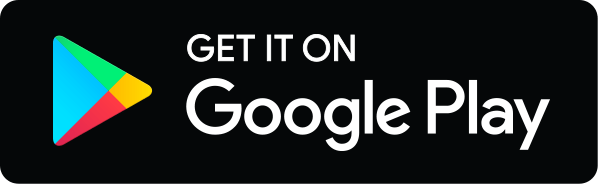