Treatment of Cancer Pain: Role of Neural Blockade and Neuromodulation
Allen W. Burton
Phillip C. Phan
Michael J. Cousins
Epidemiology
Cancer
Almost 1.4 million Americans were newly diagnosed with cancer in 2004, approximately 4,000 per day (1). In the same year, 564,000 U.S. deaths were attributed to cancer, about 22% of all deaths. It is estimated that over 10 million people in the United States are living with cancer at present, around 3% of the population. The demographic of cancer continues to slowly evolve with the overall number of cases slowly trending upward, and the number of long-term survivors, including those with chronic pain issues, continuing to grow (2).
Cancer Pain and Related Symptom Burden
The diagnosis of cancer is distressing to the patient in many ways. First, the patient fears that cancer will shorten his life. Second, the patient is apprehensive that the cancer will bring with its progression significant pain and suffering. Indeed, pain is already experienced by 20% to 50% of cancer patients initially at time of diagnosis. With cancer progression, 75% of patients with advanced cancer will experience pain (3). Up to 80% of cancer patients described their pain as having moderate to severe intensity.
According to the World Health Organization (WHO), an estimated 6.6 million people worldwide die from cancer every year (4). Recent WHO studies pointed out that cancer-related pain continued to be a significant source of global health concern. With advances in therapeutic modalities, about 80% of cancer pain can be controlled easily (5). Unfortunately, even with these advances, cancer pain remains widely undertreated even in developed countries (6). In the United States, the reasons for undertreatment of cancer pain are complex and encompasses many barriers, as outlined in Table 45-1.
Recently, there is a growing awareness that pain control is an essential part of comprehensive cancer care. Some studies have showed a direct correlation between good pain control and cancer patient’s length of survival, as well as responsiveness to timely oncologic treatment (7,8). In addition to problems stemming from immobility (deep venous thrombosis, pneumonia), uncontrolled pain has also proven to be major risk factor in cancer-related suicides (9,10). Further, issues of long-term survival with increasing treatment options prolonging survival add to the complexity of care required. The landscape of “cancer pain” is shifting quickly into a chronic pain situation in many instances, thereby blurring previous lines of distinction in treatment strategies most suited for “noncancer” versus “cancer” pain (2).
Assessment
Pain is always subjective and is experienced only by the patient. Because of the subjective nature of pain, versus an objectively measurable parameter such as blood pressure or tumor size, much research has been directed toward accurate measurement of pain and correlation with interference in activities. Over the past 20 years, the assessment of pain has been the subject of much research and refinement of techniques and instruments (see Chapters 37,38 and 46,47,48,49). A cursory review is presented in this section; the reader is directed to the references cited for more details on the assessment of cancer pain. In addition to evaluating the pain symptom, the physician has to focus on a myriad of related symptoms. The cancer patient often experiences fatigue, insomnia, depression, anxiety, somnolence, and even cognitive impairment. Increasingly, certain symptoms are thought to cluster, perhaps related to alterations in cytokine levels, and new research is focused in this area. The psychological symptoms, such as anxiety and depression, are deeply intertwined with, and can often have a large impact on, the patient’s perception and expression of pain. Furthermore, the patient can develop symptoms such as nausea, vomiting, and headaches as side effects of therapeutic intervention. The cancer patient thus commonly presents with a constellation of symptoms that have profound impact on the patient’s psychological well-being, functional status, and quality of life.
Screening Instruments
Many pain clinics utilize a questionnaire to aid in and standardize assessment.
Brief Pain Inventory
The Wisconsin Brief Pain Inventory (BPI) and Memorial pain assessment card are well-accepted and standard tools for evaluating cancer pain (11,12). At The University of Texas MD Anderson Cancer Center, an institutionally approved MD Anderson questionnaire (modified BPI) is used for initial and follow-up assessment of patients (Fig. 45-1).
Table 45-1 Barriers to effective cancer pain control | |
---|---|
|
Advantages to the Wisconsin Brief Pain Inventory (BPI) include the following:
15-minute questionnaire, which can be self-administered
Includes several questions about the characteristics of the pain and associated symptoms, including its origin and the effects of prior treatments
It incorporates two valuable features of the McGill Pain Questionnaire, a graphic representation of the location of pain and groups of qualitative descriptors. Severity of pain is assessed by a series of 0 to 10 scales (11-point numerical rating scales or NRS) that score pain at its best, worst, and on average. The perceived level of interference with normal function (on several measures including fatigue, nausea, depression, anxiety, drowsiness, difficulty thinking clearly, shortness of breath, poor appetite, insomnia, and overall feeling of well-being) is quantified with a NRS also.
Consistent evidence suggests that the BPI is cross-culturally valid and is useful, particularly when patients are not fit to complete a more thorough or comprehensive questionnaire (13,14). Further evidence shows its validity and usefulness in documenting outcomes and health status of noncancer pain patients (15).
Pediatric Cancer Pain Assessment
This complex topic is beyond the scope of this chapter, but pain assessment in the child should be undertaken in an age-appropriate manner, using the proper tool. Examples of pediatric assessment tools include Beyer’s The Oucher, Eland’s color scale-body outline, Hester’s poker chip tool, McGrath’s faces scale, and others (16) (see Chapter 47).
Pain History
Objective observations of grimacing, limping, and vital signs (tachycardia) may be useful in assessing the patient, but these signs are often absent in patients with chronic pain (see Chapter 37). Pain evaluation should be integrated with a detailed oncologic, medical, and psychological history. The initial evaluation should include evaluation of the patient’s feelings and attitudes about the pain and disease, family concerns, and the premorbid psychological history. A comprehensive but objective approach to assessment instills confidence in the patient and family that will be valuable throughout treatment.
A comprehensive evaluation of the patients with cancer pain includes the following:
Primary complaint. The chief complaint is obtained to ensure appropriate triage (i.e., a patient with severe pain due to a bowel obstruction may need to be sent to the emergency center for urgent treatment).
Oncologic history. Next, the oncologic history is obtained to gain the context of the pain problem. The oncologic history includes diagnosis and stage of disease; therapy and outcome, including side effects; and the patient’s understanding of the disease process and prognosis.
Pain history. The pain history should include any premorbid chronic pain. For each new pain site, record onset and evolution, site and radiation, pattern (constant, intermittent, or unpredictable), and intensity (best, worst, average, current) using numeric rating 0 to 10 scales, character of pain, exacerbating and relieving factors, pain interference with usual activities, neurologic and motor abnormalities (including bowel and bladder continence), vasomotor changes, and current and past analgesics (use, efficacy, side effects). Prior treatments for pain should also be noted (radiotherapy, nerve blocks, physiotherapy, etc.).
Medical record and radiologic studies review. Many of the treatments for cancer can cause pain (e.g., chemotherapy- and radiotherapy-induced neuropathies or postoperative pain syndromes; Table 45-2) (17). Many specific cancers can cause well-established pain patterns due to known likely sites of metastasis, for example, breast to long bones, spine, chest wall, brachial plexus, and spinal cord; colon to pelvis, hips, lumbar plexus, sacral plexus, and spine; and prostate to long bones, pelvis, hips, lung, and spine (18). (See Chapter 31, Tables 31-5,31-6,31-7,31-8,31-9.)
Psychological history. This should include marital and residential status, employment history and status, educational background, functional status, activities of daily living, recreational activities, support systems, health and capabilities of spouse or significant other, and past (or current) history of drug or alcohol abuse (19,20).
Medical history (independent of oncologic history). This includes coexisting systemic disease, exercise intolerance, allergies to medications and medication use, prior illness and surgery, and a thorough review of systems, including the following:
General (including anorexia, weight loss, cachexia, fatigue, weakness, insomnia)
Neurologic (including sedation, confusion, hallucination, headache, motor weakness, altered sensation, incontinence)
Respiratory (including dyspnea, cough, pneumonia)
Gastrointestinal (including dysphagia, nausea, vomiting, dehydration, constipation, diarrhea)
Psychological (including irritability, anxiety, depression, dementia, suicidal ideation)
Genitourinary (including urgency, hesitancy, or hematuria)
Physical Examination
The physical examination must be thorough, although at times it is appropriate to perform a focused examination. In patients
with spinal pain and known or suspected metastatic disease, a complete neurologic examination is mandatory. When cognitive impairment is suspected, a mini-mental status examination will clarify the level of impairment and allow tracking over time (see Chapter 37). Gonzales et al. found new evidence of metastatic disease in 64% of patients, and this finding resulted in new antitumor therapy for 18% of patients evaluated by their pain service (21).
with spinal pain and known or suspected metastatic disease, a complete neurologic examination is mandatory. When cognitive impairment is suspected, a mini-mental status examination will clarify the level of impairment and allow tracking over time (see Chapter 37). Gonzales et al. found new evidence of metastatic disease in 64% of patients, and this finding resulted in new antitumor therapy for 18% of patients evaluated by their pain service (21).
Table 45-2 Incidence of developing chronic postoperative pain by type of surgery | ||||||||||||
---|---|---|---|---|---|---|---|---|---|---|---|---|
|
Clinical Plan of Care
The clinical plan of care is developed after all items of the history are evaluated.
Formulate clinical impression (diagnosis). Multiple diagnoses usually apply, and it is optimal to use the most specific
known diagnosis. For example, T11 compression fracture (pathologic versus osteoporotic) with severe incidental pain; metastatic breast carcinoma (with known bony metastasis; nausea with dehydration; and constipation).
Formulate recommendations (plan) and alternatives for each problem. For example (related to the above problem list), magnetic resonance imaging (MRI) of the thoracic and lumbar spine with consideration of vertebroplasty if appropriate; oxycodone slow-release 10 mg twice daily, with oral transmucosal fentanyl citrate for breakthrough pain; management including further chemotherapy, radiotherapy, or bisphosphonates as deemed appropriate by the patient’s oncologist; metoclopramide 10 mg orally 30 minutes prior to meals and as needed for nausea; and addition of Senokot-S twice daily for constipation.
Coordinate care. A call to the referring oncologist and/or primary care provider (and copying them on any clinical notes) helps to ensure good communication and coordination of care between all of a patient’s physicians.
Exit interview. The exit interview with the patient is ideally performed by the physician, but any trained professional can be designated for this important duty. Items to be addressed include:
Explain the probable cause of symptoms in terms the patient can understand.
Discuss prognosis for symptom relief, management options, and specific recommendations. In addition to writing prescriptions, oral and written instructions should be provided. Educational material regarding medications, pain management strategies, procedures, or other issues should be provided. Potential side effects should be discussed.
Arrange for follow-up with clinic contact information, including an “after-hours” contact number, which is imperative because of the dynamic nature of cancer pain.
Etiology and Classification of Cancer Pain Syndromes
Pain in cancer patients can have many causes. Most cancer pain syndromes are tumor-related, but an increasing array of more chronic treatment-related painful syndromes are seen with increasing life expectancy (see Chapter 31, Tables 31-5,31-6,31-7,31-8,31-9). Numerous schemas for classification of cancer pain syndromes have been explored. This chapter explores time course and pathophysiology as relevant classifications in relation to treatment strategies.
Pain Syndrome Time Course: Acute
The large majority of acute cancer pain is due to tumor invasion of pain-sensitive structures. This invasion causes a derangement of physiologic processes including inflammation, edema, acidosis, and necrosis of pain-sensitive tissues. Further pathologic processes may include invasion of bone or soft tissues, obstruction of lymphatic or vascular vessels, distention of hollow organs, distortion of solid organs, and compression of nervous system structures (22). All of these cause typical acute cancer pain syndromes, most of which are diagnosable during the oncologic workup (see also Chapter 31, Tables 31-5,31-6,31-7,31-8,31-9). Many of these same pains improve with successful treatment of the tumor, thus reassessment is important because of the dynamic nature of tumor-related pain.
Pain Syndrome Time Course: Chronic
A significant source of pain can also be related to the cancer treatments. Most of the time, this pain is more chronic, with a gradual onset and often delayed diagnosis and treatment. Certain types of chemotherapy often cause painful peripheral neuropathy including the taxanes, platinum compounds, vincristine and its analogs, and some newer agents such as bortezomib (23). Often, this neuropathy is dose-related and resolves with a lowering of the dose of the offending agent in subsequent courses of chemotherapy. However, in some patients, the painful neuropathy is permanent, severe, and very disabling. It can lead to problems with gait or fine motor tasks of the hands. Radiation treatment may also cause neural injury in the form of plexopathy, chronic radiation myelopathy, and chronic radiation enteritis and proctitis, as well as nonspecific postradiation head and neck pain, most likely a myofascial pain syndrome (24). Other postradiation problems include an increase in long-term pelvic fractures (25).
Surgical treatment also can lead to chronic postoperative pain syndromes. For example, the post–radical mastectomy patient often has pain in the posterior upper arm, axilla, and anterior chest wall secondary to damage of the intercostobrachial nerve (26). Similarly, nerve damages during thoracotomy and radical neck dissection can cause pain in the distribution of the affected nerves (27). Phantom limb pain is especially common after amputation procedures, with burning and cramping sensation in the area of the amputated limb (28) (see also Chapter 31 and Tables 31-5,31-6,31-7,31-8,31-9).
The increasing use of combination treatments in numerous cancers, such as combined chemoradiation prior to surgical resection of head and neck or breast tumors for example, have increased cure rates. However, they may also increase the toxicity and long-term sequelae of treatment. In such cases, chronic pain may not be attributable to one or another of the therapies, and the term treatment–related toxicity has been coined to describe this new phenomenon (29).
As the pain syndromes become chronic—defined as lasting beyond the expected healing time course—the treatment algorithms become similar to those used for noncancer chronic pain. Many confusing issues may develop within this patient population, such as those patients with very slowly progressive cancer and associated pain that may last many years. In many cases the lines between treating cancer pain versus noncancer pain syndromes are blurring (2,30).
Cancer Pain Pathophysiologic Classification
In any assessment of cancer pain, an understanding of pain classification is helpful in delineating both the mechanism of pain and its responsiveness to therapeutic interventions. Pain can be broadly classified into nociceptive and neuropathic pain; however, in many patients, both mechanisms may be operating, and in all patients, psychological and environmental factors contribute to pain.
Nociceptive Pain
Somatic
Nociceptive pain occurs when non-neurologic tissues suffer insult or injury. The associated neurologic structures, however, are not injured and remain functional. Consequently, the injuries of the damaged tissues are detected as noxious stimuli, and these noxious signals are transmitted along the various pain pathways (see Chapters 31 and 32). The pain perceived by the patient is only very broadly proportional to the degree of tissue damage caused by the cancer, and this is also strongly influenced by psychologic and environmental factors. This pain experienced by the patient may be responsive to nonsteroidal anti-inflammatory drugs (NSAIDs) because tissue damage from cancer inevitably initiates activation of cellular phospholipase A2 to release arachidonic acids (AA) from cell lipid membrane. Cyclooxygenase (COX) enzymes then act on AA to produce potent inflammatory mediators such as thromboxanes, prostaglandins, and leukotrienes. Nonsteroidal anti-inflammatories, including the COX inhibitors, attenuate this initial inflammatory reaction at the site of the tissue damaged by cancer and thus reduce the initial pain signals. One must be aware of the growing concerns about cardiovascular side effects associated this group of compounds (31).
Opiate therapy is also effective in helping to control nociceptive pain. Opioid analgesics act on pain receptors at the level of spinal cord and in the brain to modulate pain pathways in the central nervous system (CNS). Nociceptive pain responds to opioids in a scaled manner, such that pain control is generally proportional to opioid dosage (32).
Nociceptive pain can further be categorized into nociceptive somatic pain and nociceptive visceral pain. Nociceptive somatic pain results from activation of nociceptive receptors in somatic tissues. These nociceptors are sensitive to mechanical, chemical, and thermal stimuli. They are located in skin, bone, muscle, tendon, joint, and connective tissues. The pain signals from these nociceptors are carried along sensory nerve fibers. The patient’s perception of nociceptive somatic pain is characterized typically as aching, dull, sharp, throbbing, and is well localized to the injured tissue site.
Visceral Pain
Nociceptive visceral pain, in contrast, is poorly localized. It originates typically from solid organs of the chest, abdomen, and pelvis. The nociceptive receptors in these solid organs typically do not respond to cutting or burning stimuli. They are, however, extremely sensitive to any mechanical stress or torsion of organs, as well as to tension or traction on mesenteric or vascular attachments to organs. The visceral nociceptive pain signals are carried by autonomic sympathetic fibers. The pain is often described as a vague, dull, aching, or pressure-like (33). The patient often perceives this nociceptive visceral pain as a referred pain that is falsely localized to a distant site. For example, pancreatic cancer pain often presents as a referred mid-back pain, and cancer involvement of diaphragm causes pain in the shoulder (see also Chapter 31 and Figs. 31-21 and 31-22).
Neuropathic Pain
The second major class of cancer pain is neuropathic pain. This pain differs from nociceptive pain in that the cancer causes direct injury to the neural tissues. Tumor destruction of peripheral nerves will cause abnormal and exaggerated pain signal transmission. Tumor invasion of peripheral or central nervous systems results in abnormal pain signal processing, integration, and perception. The cancer patient often reports extraordinary pain perception, with pain feeling different from the usual pain sensation (34). Neuropathic pain is often described as diffuse with superficial areas, is excessively sensitive to noxious stimuli (hyperalgesia/hyperesthesia), occurs even with non-noxious stimuli (allodynia), and includes abnormal painful sensations (dysesthesias). Neuropathic pain is thought to be slightly less responsive to opioid analgesics, and also requires adjuvant medications and, at times, interventional therapy for effective control (35) (see also Chapters 31, 32, 37, and 46).
Treatment Modalities
Pharmacologic Therapy
World Health Organization Guidelines
As discussed earlier, the barriers to effective pain control are multifold. As a result, clinicians have traditionally undertreated cancer pain. A growing recognition of this health issue has led to the development of numerous guidelines for treating cancer pain, including, most famously, the World Health Organization (WHO) step-ladder approach to treating cancer pain. Although too simple to serve as a comprehensive treatment algorithm, the principle of treating more resistant cancer-related pain with stronger/higher doses of opioids is generally sound (Table 45-3). (The treatment of chronic noncancer pain syndromes with chronic opioids remains controversial.)
Nonopioid Analgesics
The nonopioid class of analgesics includes both acetaminophen, NSAIDs, and perhaps tramadol. Nonopioids are commonly used for mild cancer pain, as directed by the WHO analgesic ladder. Even in the patient with advanced cancer, nonopioid analgesics are often used as co-analgesics combined with opioid (see also Chapter 33). Nonopioids help to reduce the dosage requirement of opioids, thus decreasing the opioid-associated side effects of nausea, constipation, somnolence, and cognitive impairment. However, both acetaminophen and NSAIDs can suppress a fever response, indicative of a mounting infection in the immunocompromised patient who is undergoing immunosuppressive chemotherapy.
Table 45-3 The World Health Organization (WHO) three-step analgesic ladder for cancer pain | ||||||
---|---|---|---|---|---|---|
|
Adjuvant Medications
The heterogeneous class of adjuvant medications has a defined role in the WHO three-step analgesic ladder. These agents fall into five general categories: antidepressants, anticonvulsants, oral local anesthetics, corticosteroids, and miscellaneous (Table 45-4).
These adjuvant medications act to promote certain desirable effects (or prevent opioid-related side effects) in the cancer patient. Tricyclic antidepressants are useful in certain types of neuropathic pain, especially burning dysesthetic pain. They produce significant sedation as a side effect at high dose. Consequently, they can be helpful in the cancer pain patient with insomnia and depression. Anticonvulsants and local anesthetics have a membrane-stabilizing effect and are extremely effective in neuropathic pain secondary to nerve injury in both peripheral and central nervous systems. Corticosteroids have potent anti-inflammatory effects and thus are helpful in cancer patients with spinal cord compression, intracranial tumors, organ capsule distention, and bone infiltration. Steroids also have CNS effects, improving mood and a sense of well-being in the cancer patient. Antiemetics and stool softeners should routinely be prescribed along with opioids. The other miscellaneous adjuvant medications have variable effects and are tailored to the patient’s individual pain and associated symptoms.
Opioid Analgesics
Opioid analgesics are a mainstay of treatment for cancer pain. In the WHO three-step analgesic ladder, “weak” opioids are recommended for treatment of moderate cancer pain, whereas “strong” opioids are prescribed for severe cancer pain. The weak opioids have slightly less potency and also fewer side effects. Some common oral weak opioids are listed in Table 45-5.
These weak opioids are produced in combination with acetaminophen or aspirin, thus they are “weak” because of the limitation imposed by acetaminophen or aspirin ceiling doses, above which the patient is at higher risk of renal and liver toxicity.
The “strong” opioids are more potent because they are made in pure form, without addition of aspirin or acetaminophen. These opioids do not have a maximum ceiling dose, but at higher dosages, the patient tends to experience more frequent and significant side effects of nausea and vomiting, constipation, pruritus, somnolence, and cognitive impairment. Some common strong opioids are listed in Table 45-6.
Table 45-4 Adjuvant drugs for treatment of cancer pain | ||||||||||||||||||||||||||||||||||||||||||||||||
---|---|---|---|---|---|---|---|---|---|---|---|---|---|---|---|---|---|---|---|---|---|---|---|---|---|---|---|---|---|---|---|---|---|---|---|---|---|---|---|---|---|---|---|---|---|---|---|---|
|
Failure of Noninterventional Therapies
It has been claimed that 70% to 90% of patients with cancer pain have satisfactory pain relief from pharmacologic therapies alone, following the WHO guidelines (36). This means, however, that 10% to 30% of these patients have pain that cannot be effectively managed with medications alone. Additionally, it must be pointed out that the standard 70% to 90% success rates using the WHO step-ladder include patients with mild, moderate, and severe pain grouped together, with higher success rates in the mild pain group. Thus, the 70% to 90% adequate cancer pain treatment almost certainly overestimates WHO approach success in the most severe cancer pain syndromes. The reasons for this failure of pharmacologic treatment are variable (37), varying from physician- to patient-related factors. The physician-related reasons for pharmacologic failure include inaccurate assessment of pain and deficiency in knowledge of current analgesics and adjuvant medications. Patient-related reasons range from fear of addiction to appearance of adverse side effects (38,39). Failure of pharmacologic control of cancer pain due to lack of efficacy of existing drugs in some patients is also commonly seen with cancer progression. Patients with advanced disease can present with intractable pain from multiple metastases and multiple pain sites (see Chapter 31, Tables 31-5,31-6,31-7,31-8,31-9).
Table 45-5 “Weak” opioids for treatment of moderate cancer pain | ||||||||
---|---|---|---|---|---|---|---|---|
|
Interventional Pain Therapies
Goal of Intervention
Patients who fail to respond to conservative pharmacologic treatments may be candidates for interventional therapies. A large armamentarium of invasive procedures is available to achieve better control of cancer pain. However, the role of interventional therapies must be placed in the proper context. Usually, these procedures cannot be employed as the sole
treatment for cancer pain, especially for advanced cancer patients. Rather, they should be used as part of a multimodality approach in the treatment of cancer pain (40,41). However, in cases in which the risk–benefit ratio is favorable and outcome evidence is strong (i.e., celiac plexus block), the patient need not “fail” WHO ladder therapy to be a candidate for interventional pain therapy. As discussed previously, the etiologies of cancer pain are diverse, and pain is interrelated with the constellation of symptoms experienced by the cancer patient. Consequently, a global, multimodal approach to treatment of cancer pain is necessary. It includes the appropriate antineoplastic therapy, management of analgesics and adjuvant pain medications, behavioral and psychiatric support, and finally interventional therapies. Most often, interventional pain procedures will not completely eliminate the need for pain medications. The therapeutic goal of such procedures is to help alleviate cancer pain, reduce the overall analgesic need, and thereby minimize associated opioid-related side effects, with the overall aim of enhancing quality of life.
treatment for cancer pain, especially for advanced cancer patients. Rather, they should be used as part of a multimodality approach in the treatment of cancer pain (40,41). However, in cases in which the risk–benefit ratio is favorable and outcome evidence is strong (i.e., celiac plexus block), the patient need not “fail” WHO ladder therapy to be a candidate for interventional pain therapy. As discussed previously, the etiologies of cancer pain are diverse, and pain is interrelated with the constellation of symptoms experienced by the cancer patient. Consequently, a global, multimodal approach to treatment of cancer pain is necessary. It includes the appropriate antineoplastic therapy, management of analgesics and adjuvant pain medications, behavioral and psychiatric support, and finally interventional therapies. Most often, interventional pain procedures will not completely eliminate the need for pain medications. The therapeutic goal of such procedures is to help alleviate cancer pain, reduce the overall analgesic need, and thereby minimize associated opioid-related side effects, with the overall aim of enhancing quality of life.
Table 45-6 “Strong” opioids for treatment of severe cancer pain | ||||||||||||||
---|---|---|---|---|---|---|---|---|---|---|---|---|---|---|
|
Communication
Prior to proceeding with any invasive pain procedure, communication between the pain physician and the relevant parties is absolutely essential. The patient must first be educated about the risks and benefits of the interventional procedure. He must be allowed an opportunity to have questions about the procedure extensively and satisfactorily answered. He must, at the same time, be grounded in realistic expectations in terms of outcomes and possible complications from the chosen procedure. The patient should be made aware of the efficacy of the procedure, duration of effectiveness, and possibility of failure of the procedure to provide complete or even partial pain relief. He must understand that the interventional procedure is part of a multimodal approach to pain control.
With cancer patients, especially those critically ill or preterminal, family members and caregivers are often involved in the decision-making process. Family support helps the patient cope emotionally with cancer disease and with procedural interventions. Like the patient, the family must also be educated and have realistic expectations about the procedure.
Effective communication with other professional members of the care team is also important. These include the patient’s oncologists, primary care providers, and all the relevant consultants. Treatment of cancer is a multidisciplinary effort. The interventional procedure should be planned in coordination with the overall cancer treatment. For example, the cancer patient may undergo chemotherapy with resultant thrombocytopenia. In such case, the interventional procedure must be carried out prior to or following chemoinduction, when the patient’s platelet count is normalized. Typically, other members of the patient’s care team are informed about the planned interventional procedure and given a chance to voice any input or concern.
Detailed Physical Examination
A thorough physical examination of the patient prior to the procedure is also critical. This entails a complete neurologic evaluation. Interventional procedures for pain control involve neurologically sensitive tissues such as nerves and CNS structures. The objective of intervention is to disrupt or modulate pain pathways involved in nociception. Interventional procedures like neurolysis of peripheral nerves will not only block pain transmission but also block sensory and motor innervations as well. Consequently, it is important to document before and after the procedure a complete and thorough physical examination, with focus especially on pain and neurologic changes. Changes such as sensory and motor blockade are closely monitored after procedures.
Categories of Interventional Techniques
Interventional therapies can be categorized into three groups: neurolytic techniques, neuromodulatory techniques, and surgical techniques. Neurolytic or neuroablative techniques are procedures that target the destruction of those nerves or neural structures that are involved in the generation or transmission of pain signals. Neurolytic lesions can be created using a variety of agents. Lysis is achieved with chemicals (glycerol, alcohol, or phenol), heat (radiofrequency coagulation), or cold (cryotherapy) (see also Chapters 39, 42, and 44).
Neuromodulatory techniques have as their basis Wall and Melzack’s gate control theory of pain (42) (see Chapters 40 and 41), which is also discussed in light of current knowledge in Chapter 51. Regardless of the exact locus of such a gate, the concept is accurate in describing how afferent input of all types is heavily modulated along the route of transmission into the brain. All of these modulation sites become therapeutic targets when discussing the treatment of pain (43). Neuromodulative techniques aim to modulate pain signals along the transmission pathway. These techniques include local anesthetic blockade or regional infusion of drugs at epidural, intrathecal, intraventricular, or perineural sites, and the electrical stimulation of the CNS and peripheral nervous system (PNS).
Surgical techniques are another class of interventional pain procedures, ranging from minimally invasive percutaneous vertebroplasty to highly invasive neurosurgical destructive techniques, including percutaneous cordotomy, dorsal root entry zone (DREZ) lesions, thalamotomy, cingulotomy, hypophysectomy, and trigeminal tractomy. Most of these procedures are done stereotactically under monitored anesthesia care (MAC) and fluoroscopic or computed tomography (CT) guidance. Nondestructive neurosurgical techniques include deep brain stimulation and motor cortex stimulation; both of these are currently experimental.
Neurolytic Procedures
Over the past century, many chemical and physical ablative techniques have been developed with the goal of disrupting the transmission of pain signals along the neural pathways. Chemical neurolysis is achieved with alcohol (50%–100%), phenol (5%–15%), and glycerol (100%). These agents produce nerve injury that results in degeneration of nerve fiber distal to the lysis lesion (Wallerian degeneration) (44). This disrupts the nerve cell transmission of pain and results in a nociceptive block. However, after Wallerian degeneration, the nerve axon can regenerate within 3 months. Thus, chemical neurolysis provides a temporary block of nociception lasting about 3 to 6 months (44,45).
In the past, alcohol has been classically used in neurolysis (46). Many clinicians today favor the use of phenol for peripheral neurolysis because it is less neurotoxic than alcohol (47). Alcohol is used in concentrations up to 100%. It causes nonselective destruction of all nerves, as well as surrounding soft tissues. Alcohol is rapidly soluble in blood and is hypobaric relative to cerebrospinal fluid (CSF). If injected into the intraspinal
space, it will rise rapidly against gravity, diffusing away from the initial injection site. Specifically, it damages nerves by extracting fatty substances and precipitating proteins in the nerve axon, resulting in Wallerian degeneration (48). The lesion is proportional to both the concentration and volume of alcohol used. There may be a higher risk of neuritis associated with alcohol injection versus other techniques (49). Also, patients usually experience intense burning pain initially with alcohol injection. However, after intrathecal injection, patients experience a warm numb feeling.
space, it will rise rapidly against gravity, diffusing away from the initial injection site. Specifically, it damages nerves by extracting fatty substances and precipitating proteins in the nerve axon, resulting in Wallerian degeneration (48). The lesion is proportional to both the concentration and volume of alcohol used. There may be a higher risk of neuritis associated with alcohol injection versus other techniques (49). Also, patients usually experience intense burning pain initially with alcohol injection. However, after intrathecal injection, patients experience a warm numb feeling.
Phenol, now more commonly used, is thought to be associated with lower risk of postablation neuritis (49). Phenol 5% is equivalent to alcohol 40% concentration in neurolytic potency (50). Phenol is less water-soluble than alcohol and thus tends to concentrate more around the injection site. It acts by diffusing into the axon and denaturing proteins, causing Wallerian degeneration. Many clinicians prefer phenol because the intensity (or “density”) and duration of neural blockade are less with phenol and thus provide a wider margin of safety.
Less commonly used is glycerol in peripheral neural blockade. It is used primarily for treatment of trigeminal neuralgia. It is injected directly into the trigeminal cistern (cave of Meckel) with good efficacy in treating trigeminal neuralgia. Other neurolytic chemicals that have been tried include ammonium salt compounds and hypertonic and hypotonic saline solutions, with variable results (44). Radiofrequency thermocoagulation (RFTC) or radiofrequency ablation (RFA) is also employed to produce a physical nociceptive block (51). As opposed to chemical neurolysis, the lesion created by radiofrequency heating is discrete, and the size of the lesion is controlled by the temperature of the probe and duration of application. Some studies have shown that radiofrequency ablation can produce a longer-lasting block versus chemical neurolytic techniques (52) (see Chapters 42 and 44).
Cryotherapy can also be used to achieve neurolytic lesioning. Applying extreme cold to the nerve will result in long-lasting nerve blockade (44). Intraoperative cryoneurolysis of intercostal nerves has been reported with good efficacy in controlling postthoracotomy pain (53). Wide application of cryoablation has been hindered by the large size of the probe (14 g), relative complexity of the equipment involved (requires a compressed nitrogen source), and the relatively short duration of the lesion versus the aforementioned techniques (see Chapter 42).
Patient selection for chemical neurolysis, radiofrequency ablation, and cryoneurolysis techniques is extremely important in achieving the desired outcome. In most cases, the patient should have an advanced, progressive cancer with a limited life expectancy (6–12 months). The pain should be severe, persistent, refractory to conservative treatment, and amenable to neural blockade (discrete and localized to a region served by one or two nerves, i.e., the chest wall or jaw line). Potential risks are associated with neuroablation, as outlined in Table 45-7. Despite the inherent risks of neuroablation however, patients with advanced cancer and well-localized nociceptive pain can benefit greatly from neurolytic blockade. Peripheral, central, and sympathetic neurolysis has been used for many decades to alleviate suffering in cancer patients with intractable pain (54).
Neural Blockade in Head and Neck
Over 40,000 patients are diagnosed with head and neck cancer each year in the United States, and over 500,000 are diagnosed worldwide (55). Cancer of the head and neck constitutes about 5% of all malignant diseases in the United States, and affects about 6.6 million worldwide (56). Because of dense facial and neck innervations, cancer commonly causes not only disfiguring facial lesions but also disabling pain in face and neck. The incidence of pain following treatment for head and neck cancer may be as high as 50%, with more than 50% disabled 1 year after diagnosis, highly correlated to pain scores (57,58,59). Blocking relevant trigeminal or cervical nerve branches can control such pain. Efficacy of interventional nerve block, however, can be affected by tumor distortion of anatomy, radiation-induced fibrosis of local tissues, and possible overlapping sensory innervations of neighboring cranial nerves V, VII, IX, X, and upper cervical nerves (see Chapter 17). Careful anatomic evaluation of facial pain and proper selection of nerve block with a local anesthetic test block is indicated prior to consideration of neurolytic blockade in these patients.
Table 45-7 Potential risks with neuroablation techniques | |
---|---|
|
Trigeminal Nerve Block
Indications
Blockade of trigeminal ganglion or specific branches of trigeminal nerves will alleviate somatic and neuropathic pain from cancer. Trigeminal neuralgia (tic douloureux) is discussed in Chapter 42.
Anatomy
The trigeminal or Gasserian ganglion is formed from two nerve roots that arise from the ventral pons of the brainstem. These roots fuse anteriorly and enter the Meckel cave, a recess in the middle cranial fossa. Three sensory divisions then exit anteriorly as the ophthalmic nerve (V1), maxillary nerve (V2), and the mandibular nerve (V3). Destruction of the Gasserian ganglion may be useful in alleviating intractable pain from invasive tumors of the orbit, maxillary sinus, and mandible (60). Conversely, each individual sensory nerve can be blocked separately, if selective blockade is desired.
Techniques
Local anesthetic, neurolytic, and radiofrequency lesioning techniques for Gasserian ganglion blockade are described in Chapter 42 (see Figs. 42-34,42-35,42-36,42-37,42-38). Radiographs showing the position of the needle are presented in Figure 45-2.
Complications
After local anesthetic or neurolytic agent injection, inadvertent vascular puncture can lead to neurotoxicity, including seizure and death. When the needle is directed through the pterygopalatine fossa, injury to blood vessels can result in facial hematoma or ocular subscleral hematoma. Postprocedure neuritis can cause significant dysesthetic pain in trigeminal sensory areas. Ocular dryness and masticator weakness are also possible after neurolytic trigeminal block (see also Chapter 20).
Occipital Nerve Block
Indications
Occipital nerve block is effective in treating oncologic pain in the posterior scalp and occipital region.
Anatomy
Most of the posterior scalp is innervated by the greater occipital nerve, which arises from the dorsal rami of the C2 nerve root (see Chapter 17, Fig. 17-2). It emerges subcutaneously in the posterior scalp just slightly inferior to the superior nuchal line and 2 to 3 cm lateral to the greater occipital protuberance. At this point of emergence, it is just medial to the occipital artery (61) (see Chapter 17, Fig. 17-10).
Techniques
The occipital nerve block technique is described in Chapter 17 (see Fig. 17-10). In the case of occipital neuralgia, the patient may be a candidate for suboccipital peripheral nerve stimulation (see Chapter 41). Radiographs showing the final position of suboccipital electrodes are presented in Figure 45-3.
Complications
Because of the superficial location of the greater occipital nerve and relative ease of this block, complications are minimal. The close proximity of the greater occipital artery may increase risk of intravascular injection, which can be avoided by frequent aspiration while slowly injecting the neurolytic solution. Neuritis can also occur with neurolytic agents, especially with alcohol (see also Chapter 20).
Superficial Cervical Plexus Block
Indications
Superficial cervical plexus block is often used to treat cancer pain in the dermatome of the neck innervated by the cervical plexus.
Anatomy
The superficial and deep cervical plexus arise from the first four cervical nerves. The cervical plexus lies just lateral to the first cervical vertebrae. It is located anterior to the levator scapulae and middle scalene muscles, and posterior to the sternocleidomastoid (SCM) muscle. The plexus gives off both superficial sensory branches and deep motor branches. This anatomic division of sensory and motor nerves allows for selective sensory blockade of the superficial sensory branches of the cervical plexus without compromising the motor function of the neck muscles.
These superficial branches arise from the plexus and pierce the deep fascia of the neck at the posterior border of the SCM muscle (see Chapter 17, Fig. 17-9). It is at this point, where the bundle of the superficial cervical plexus emerges, that sensory innervation to the plexus can be blocked easily (62). The superficial cervical plexus gives rise to the sensory nerves that supply sensation to the skin and superficial fascia of the head, neck, and shoulder. These include the lesser occipital, greater auricular, accessory, anterior cervical, and suprascapular nerves (63).
Techniques
The technique for this block is described in Chapter 17 (see Fig. 17-9). If local anesthetic block is successful, it can be followed with neurolytic agents to achieve a longer blockade. In this region, the authors use a dilute (3%) phenol solution because of the vascular structures and muscles in the area. A note of caution is that the external jugular vein crosses the midpoint of the lateral border of the SCM. Careful aspiration prior to infiltration is helpful to avoid intravascular injection (64).
Complications
There are few complications with this superficial cervical block. The most common complication is intravascular injection into the external or internal jugular vein, with resulting systemic toxicity. Also, placement of needle into the jugular vein may result in hematoma or even air embolism (see also Chapter 20).
Neural Blockade of Upper Extremity
Brachial Plexus Block
Indications
Malignancy involving the upper extremity includes sarcoma of bone as well as soft tissue sarcoma. In the
United States, almost 13,000 new cases of bony sarcoma and 7,000 new cases of soft tissue sarcomas are diagnosed each year (65). In most cases, surgical resections of sarcomas with limb-sparing procedures are performed with good result. These patients, however, often have severe pain from direct tumor invasion of the neurovascular bundle or as a consequence of surgical tumor resection. Neural blockade of the brachial plexus is effective in controlling somatic nociceptive pain in upper extremity cancer.
United States, almost 13,000 new cases of bony sarcoma and 7,000 new cases of soft tissue sarcomas are diagnosed each year (65). In most cases, surgical resections of sarcomas with limb-sparing procedures are performed with good result. These patients, however, often have severe pain from direct tumor invasion of the neurovascular bundle or as a consequence of surgical tumor resection. Neural blockade of the brachial plexus is effective in controlling somatic nociceptive pain in upper extremity cancer.
For short-term palliation of cancer pain, brachial plexus block can also be performed with a catheter left in place for continuous infusion of local anesthetic. In cases of severe intractable pain from invasive tumors of the brachial plexus or soft tissues and bone of the shoulder and upper extremity, destruction of the brachial plexus may be indicated. The patient should be made aware of the full consequences of neurolysis of the brachial plexus, including paralysis of the upper extremity. Many patients who are candidates for this block will already have significant motor deficits in the extremity (66).
Anatomy
The brachial plexus is formed from fusion of the ventral rami of C5–T1 nerve roots. These nerve roots, with possible contribution from C4 and T2, emerge from the lateral aspect of the vertebral bodies and run laterally and inferiorly, in the interscalene compartment. These nerves of the brachial plexus run down the interscalene compartment to pass behind the clavicle and above the top of the first rib, then into the axilla (see Chapter 13, Figs. 13-1,13-2,13-3,13-4,13-5).
Techniques
There are multiple approaches to the brachial plexus blockade, including interscalene block, supraclavicular block, and axillary block. In cancer pain from tumor involvement of the shoulder, interscalene block is preferred (67). The techniques are described and illustrated in Chapter 13, Figures 13-6,13-7,13-8,13-9,13-10,13-11,13-12,13-13,13-14,13-15.
Once efficacy of local anesthetic blockade in relieving cancer pain has been proven, the patient may wish to proceed with a longer-lasting neurolytic block using phenol. A volume of 20 mL of 6% phenol is slowly injected into the intrascalene compartment of the brachial plexus. The neurolytic block is done under fluoroscopic guidance in our facility. Again, motor paralysis of the upper extremity can be expected with this neurolysis of the brachial plexus. Another method of neurolysis of the brachial plexus produces a radiofrequency lesion of the cervical nerve roots (see Chapter 42), which we have done in our institution in some cases of pain refractory to brachial plexus neurolysis.
If a less prolonged blockade of the brachial plexus is desired, a continuous local anesthetic infusion of the brachial plexus can be performed. The infraclavicular approach for brachial plexus block is preferred here, because the catheter can remain in the same position for up to 3 weeks (68). The infraclavicular entry site permits easy catheter threading into the plexus, and catheter position is not affected by the patient’s movement. The technique for infraclavicular block and insertion of a catheter for infusion is described in Chapter 13, Figures 13-20,13-21. Once the infraclavicular catheter has been inserted and the catheter is sutured well in place, infusion of 0.125% or 0.25% bupivacaine is used to provide continuous brachial plexus analgesia. It is effective in controlling somatic pain for several days and sympathetically mediated pain for up to a few weeks.
Complications
Complications from interscalene block are possible because of its proximity to many sensitive structures in the neck. Intravascular injection, as mentioned earlier, will lead to systemic toxicity including seizure. Subarachnoid injection can cause sensory, motor, total spinal anesthesia, and even death. Phrenic nerve block is an expected condition that accompanies a successful interscalene block. In patients with respiratory compromise, the patient’s ability to tolerate a local anesthetic phrenic nerve block with hemidiaphragm paralysis should be considered before neurolytic blockade.
Complications for infraclavicular brachial plexus block are similar to interscalene block. Proximity to the subclavian artery and vein increases the potential for intravascular injection. The apex of the lung is also close, so pneumothorax risk is higher also. Although the probability of phrenic nerve block is less with the infraclavicular approach, the risk of recurrent laryngeal nerve blockade and consequent vocal cord paralysis is higher (see also Chapters 20 and 50).
Neural Blockade in Thorax
Intercostal Nerve Block
Indications
Lung cancer is the number-one cancer killer in both males and females. It accounts for 15% of malignant disease in males and 13% in females. Patients diagnosed with lung cancer often require thoracotomy, with surgical biopsy or resection of tumor mass. Many such patients experience chest wall pain from either direct tumor involvement or from surgical trauma to intercostal nerves (69). In addition to lung cancer, aggressive breast cancer may also invade ribs and intercostal nerve bundles to cause pain (70).
Tumor invasion of lung parenchyma and visceral pleura is not thought to cause pain because these are nociceptive-insensitive structures. However, cancer involvement of the parietal pleura will elicit a pain response. Pain is transmitted from parietal pleura along somatic nerves, including the intercostal nerves from T1 to T12. Intercostal nerve blockade is effective in blocking this somatic pain (71).
Anatomy
The intercostal nerves are formed from the ventral rami of thoracic nerves from T1 to T12. Each nerve, joined by an intercostal vein and artery, runs in a neurovascular bundle in the subcostal groove. It gives off four branches as it runs anteriorly in the intercostal space. The first is the gray rami communicantes, which joins the sympathetic ganglion. The second branch is the posterior cutaneous nerve, which innervates the paravertebral region. The third branch is the lateral cutaneous nerve, which innervates the axilla and lateral chest wall. The fourth is the anterior cutaneous nerve, which innervates the anterior thorax (see Chapter 16, Fig. 16-4). Considerable sensory overlap occurs between those branches as well as between the intercostal nerves themselves. Thus, pain from one area may require blockade of multiple adjacent intercostal nerves (72).
Techniques
For neurolytic block, the procedure should be performed under fluoroscopic or ultrasound guidance. Also, some new data support increased efficacy with proximal radiofrequency lesioning of the dorsal root ganglion (DRG) (see also Chapter 42). The details of this technique are described in Chapter 16, Figures 16-6,16-7,16-8,16-9,16-10,16-11,16-12. Once the needle is in place, water-soluble contrast dye injected into this groove should show a nice spread along the inferior border of rib. A neurolytic solution of 10% phenol can be injected, using 3 to 5 mL for each intercostal block. The proximal approach to the DRG is generally done under fluoroscopic guidance, using an oblique approach and a curved needle. Sensory testing with radiofrequency allows the operator to manipulate the needle tip in the foramen to optimal position (i.e., until the paresthesia reproduces the patient’s pain; see Chapter 42).
Complications
Some common complications with intercostal nerve blockade include pneumothorax and systemic toxicity. Pneumothorax results from needle puncture through parietal and visceral pleura. This will create an air leak from lung into pleural space. A simple pneumothorax may progress into a tension pneumothorax with its life-threatening implications. The patient should be closely monitored after the procedure, and a postprocedure chest radiograph is recommended.
Another complication is systemic toxicity from absorption of anesthetic or neurolytic solution into the intercostal neurovascular bundle. Because of the close proximity of the intercostal artery and vein to nerve, absorption of injected solution into the intercostal space is likely. However, considering the small volume used for neurolysis, systemic toxicity is less likely. Another less likely complication is neuraxial spread of the anesthetic or neurolytic solution. A clear risk is the development or worsening of preexisting neuropathic pain as a result of neuroplastic changes in response to the intercostal denervation.
Instead of chemical neurolysis, cryoanalgesia and radiofrequency ablation have also been used in intercostal nerve blockade (see Chapter 42). Cryoanalgesia or freezing of intercostal nerves has been shown to control pain in postthoracotomy patients if done under direct visualization of the intercostal bundle at termination of surgery (72).
Intercostal nerve radiofrequency ablation of the DRG can also be used for long-lasting blockade. A blunt-tipped 100-mm 22-gauge radiofrequency electrode with a 5-mm active tip is inserted into the subcostal space (see Chapter 42). One mL of 2% lidocaine is injected into the electrode cannula. Lesioning is then accomplished by coagulation at 80°C for 60 seconds or using other similar lesioning parameters. We use a curved-tip probe to optimally locate the DRG in the proximal neural foramen (Figs. 45-4 and 45-5).
Sympathetic Blockade for Cancer Pain
Visceral pain arises from cancer involvement of sympathetically innervated organs. Innervation of viscera is best described as being through “visceral nociceptive afferents,” since such fibers utilize sympathetic plexuses and ganglia as an anatomic route to the DRG. Insults to these organs can be from abnormal distention of organ wall or viscus, tension or torsion on mesenteric vessels, and ischemia. Such visceral pain is commonly seen with gastrointestinal malignancies such as hepatic metastases, intestinal tract tumor, and pancreatic cancer. Sympathetically mediated pain (SMP) can be part of a neuropathic pain problem (most commonly complex regional pain syndrome or CRPS), as when there is direct injury to nervous tissue such as occurs in brachial and lumbosacral plexopathies (73,74) (see Chapter 46). No evidence suggests that sympathetic blockade provides long-term relief of SMP, despite some patients obtaining short- to medium-term relief. Studies have shown that sympathetic blockade may provide short-term relief of SMP (74,75) (see Chapters 39 and 46).
![]() Figure 45-4. Intercostal nerve radiofrequency ablation at level of dorsal root ganglion (DRG). Fluoroscopy image showing needle in final position. Anteroposterior view. |
Anatomy
The sympathetic axis is made up primarily of a pair of ganglionated paravertebral chains that run from the base of the skull to the tip of the coccyx. It also consists of several major vertebral plexuses, including the celiac, cardiac, and hypogastric plexuses (see Chapter 39). Table 45-8 lists major sympathetic structures and the corresponding tissues innervated (75).
These sympathetic structures are attractive targets for blockade of visceral pain (75,76,77,78,79,80,81,82,83,84,85,86) and ischemic limb pain (82,83) (see Chapter 39), as well as SMP (see Chapter 46). Interruption of pain pathways at these discrete sites has been demonstrated to have a useful role in controlling oncologic pain (76,77). Sympathetically mediated pain associated with CRPS is much more difficult to manage, probably because of the central neuroplastic changes associated with CRPS (see Chapter 46).
Table 45-8 Sympathetic structures | ||||||||||||||
---|---|---|---|---|---|---|---|---|---|---|---|---|---|---|
|
Stellate Ganglion Block
Indications
Stellate ganglion block helps control SMP in the head, neck, and upper extremity. Sympathically mediated pain is commonly seen in tumor invasion of brachial plexopathy or in Pancoast tumor (78).
Anatomy
The stellate or cervicothoracic ganglion is formed from fusion of the inferior cervical and the first thoracic sympathetic ganglia. A detailed description of the efferent sympathetic nerves and cervicothoracic sympathetic ganglia is given in Chapter 39.
Techniques
Because of many sensitive structures in the neck adjacent to the stellate ganglion, fluoroscopic imaging is recommended. The patient is placed in the supine position with neck slightly extended. The classic technique is to identify the Chassaignac tubercle at C6 and enter the needle at this site (see Chapter 39, Figs. 39-26,39-27,39-28). However, many clinicians recommend Racz’s technique at the C7 level for its improved safety margin (79). The patient’s neck is prepared in a sterile manner with Betadine, and the skin over the body of the C7 vertebral body is anesthetized with local 1% lidocaine. The proceduralist’s nondominant hand is used to palpate the carotid artery at the level of C7 and retract it laterally. The dominant hand introduces a 1.5-inch, 22-gauge needle just medial to the palpated carotid artery and directs that needle in a medial direction. The target of the needle tip is the ventrolateral aspect of the C7 vertebral body, not the transverse process, as seen in
the classic approach. Once the needle tip contacts the C7 vertebral body, it is slightly withdrawn about 1 mm. The needle is then carefully aspirated for CSF or blood. A contrast dye, 1 to 2 mL, is injected to confirm the caudocephalad spread in front of the prevertebral facia (Fig. 45-6). For gangliolysis, a mixture of 3% phenol, local anesthetic, and steroid is used (i.e., 5 mL of 6% phenol in saline, 5 mL of 0.5% bupivacaine, and 80 mg of methylprednisone).
the classic approach. Once the needle tip contacts the C7 vertebral body, it is slightly withdrawn about 1 mm. The needle is then carefully aspirated for CSF or blood. A contrast dye, 1 to 2 mL, is injected to confirm the caudocephalad spread in front of the prevertebral facia (Fig. 45-6). For gangliolysis, a mixture of 3% phenol, local anesthetic, and steroid is used (i.e., 5 mL of 6% phenol in saline, 5 mL of 0.5% bupivacaine, and 80 mg of methylprednisone).
![]() Figure 45-6. Stellate ganglion block at C7 level. Fluoroscopy image after injection of contrast media. |
The volume of this mixture injected is dependent on the blockade desired. A volume of 10 mL of neurolytic mixture is adequate for stellate ganglion blockade of SMP to the head and upper extremity. If blockade of pain from thoracic viscera is desired, a volume of 15 to 20 mL of neurolytic mixture is necessary. During injection, the clinician must exercise caution to prevent intravascular injection. Even 1 mL of local anesthetic injected into carotid or vertebral artery can cause loss of consciousness and seizure. An initial test dose, frequent aspiration, and slow injection are good safety measures.
Efficacy
Evidence of sympathetic blockade in the head can include a myriad of symptoms characteristic of Horner syndrome such as miosis, ptosis, enophthalmos, facial anhidrosis, and nasal congestion. Sympathetic blockade to the upper extremity results in visible engorgement of veins in the hands and forearm. Resolution of prior pain suggests that such pain is sympathetically mediated. (However, see Chapter 38 describing diagnostic and prognostic neural blockade.)
Complications
Because of anatomic proximity to critical structures, serious complications of stellate ganglion block can include pneumothorax, anesthetic-induced seizure from intravascular injection, and total spinal anesthesia from subarachnoid injection. Other less serious problems include localized hematoma, infection, or neck muscle tenderness. Many clinicians fear a permanent Horner syndrome with neurolysis. Anecdotally, Racz et al., however, have not reported any long-term Horner syndrome using their modified technique just discussed (79).
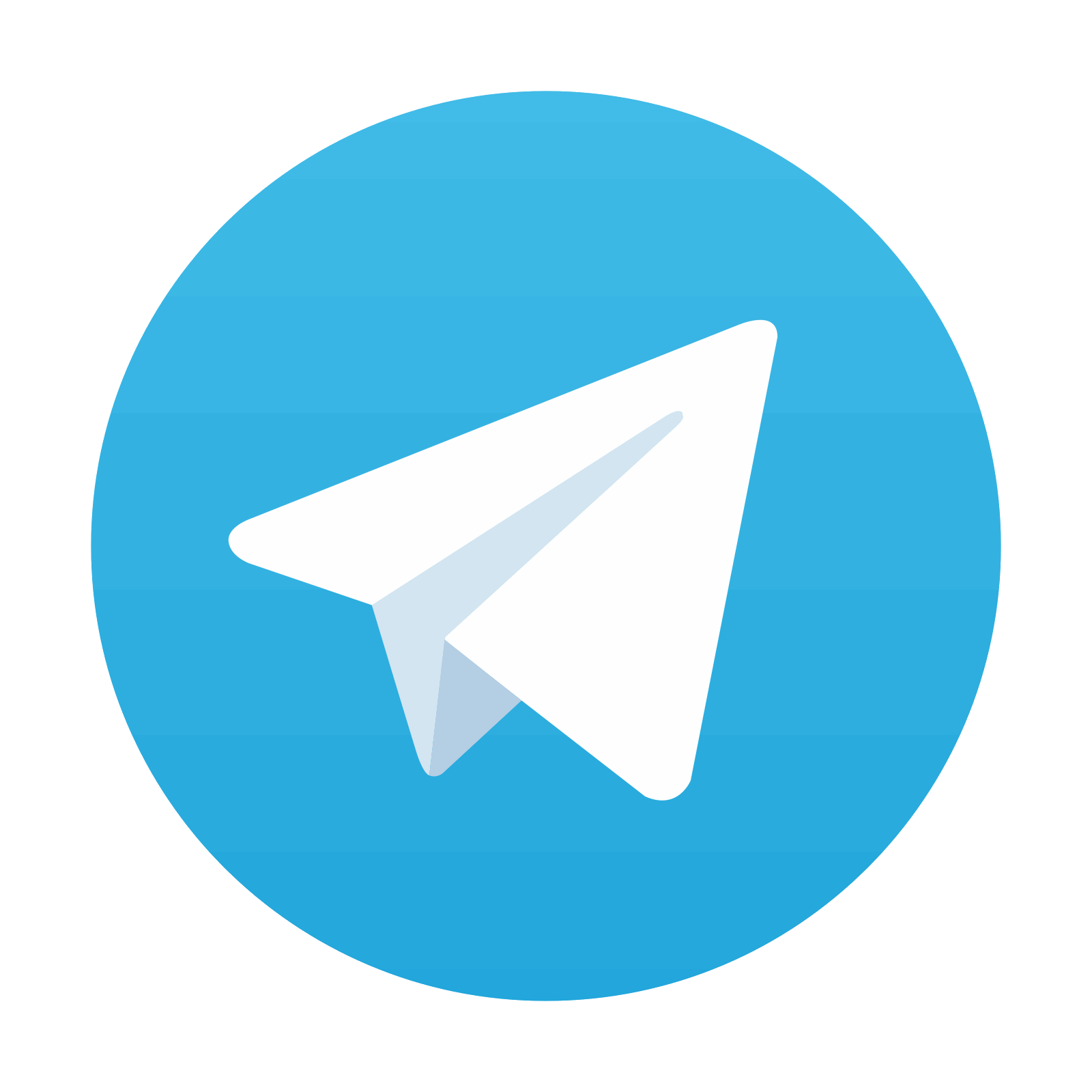
Stay updated, free articles. Join our Telegram channel

Full access? Get Clinical Tree
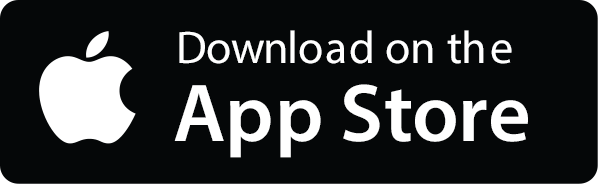
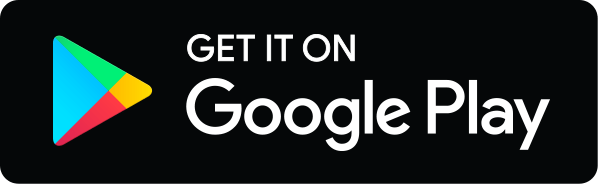
