TRAUMATIC BRAIN INJURY
Impact of traumatic brain injury
Brain Trauma Foundation clinical practice guidelines
Initial resuscitation
Critical care management after initial resuscitation
STROKE
Ischemic stroke
1. Pathophysiology
2. Diagnosis
3. Treatment
Hemorrhagic stroke and intracerebral hemorrhage
1. Pathophysiology
2. Diagnosis
3. Treatment
BRAIN DEATH AND ORGAN DONATION
The importance of brain death and organ donation
Physiology of brain death
Diagnosis of brain death
Care of the brain-dead organ donor
Non-heart-beating donors
I. TRAUMATIC BRAIN INJURY
A. Impact of traumatic brain injury
Each year in the United States, approximately 52,000 people die and 70,000 to 90,000 suffer permanent disability because of TBI. TBI results from the primary injury, or structural damage from the initial transfer of force, and the secondary injury, or cerebral ischemia from subsequent systemic and cerebral events. A study based on information from the Traumatic Coma Data Bank revealed that, in patients who sustained severe head injury (Glasgow Coma Scale Score [GCS] score ≤ 8), the presence of hypotension (systolic blood pressure [SBP] < 90 mm Hg) and/or hypoxia (arterial oxygen tension [PaO2] < 60 mm Hg) at the time of arrival at the hospital was associated with increased mortality and worsened outcomes in survivors, independent of the severity of injury. The mortality was 75% when patients had both hypotension and hypoxia.
Intracranial complications, such as edema, hemorrhage, and vasospasm, contribute to a reduction in cerebral blood flow (CBF) on post-TBI day 1 to almost 50% of the pretrauma baseline average of 45 mL/100 g of brain/minute. An estimated CBF ≤ 15 mL/100 g/minute is associated with irreversible cerebral damage. To minimize secondary injury and associated complications, patients who have moderate or severe TBI require treatment in an intensive care unit (ICU).
B. Brain Trauma Foundation clinical practice guidelines
The Brain Trauma Foundation (BTF) developed evidence-based clinical practice guidelines (CPGs) that are revised periodically as new data become available. Recent studies suggest that ICU protocols based on BTF guidelines can decrease morbidity, mortality, and cost of care.
C. Initial resuscitation
Patients who have TBI should be either treated at a designated trauma center that has neurosurgical coverage or transferred to such a center after initial stabilization. Investigators noted a 50% increase in mortality when patients who sustained TBI were initially transported to a non-trauma center. Patients transported to non-trauma centers should be transferred to designated trauma centers as soon as they are stabilized.
The prompt assessment and management of TBI begin with the treatment of associated injuries that may cause hypoxia, hypoventilation, and shock. This is best accomplished using a systemic approach such as the Advanced Trauma Life Support (ATLS) Algorithm, which consists of primary and secondary surveys.
1. Primary survey
To the extent possible, a brief history is obtained according to the AMPLE mnemonic (Allergies, Medications, Past medical history, Last meal, Event). Examination and immediate resuscitation are performed according to the ABCDE mnemonic (Airway, Breathing, Circulation, Disability, Exposure). Plans for initial operative or non-operative management are based on the results of the primary and the secondary surveys.
a. Airway: In the setting of TBI, airway management is performed with particular attention to changes in MAP, ICP, arterial oxygen tension (PaO2), arterial carbon dioxide tension (PaCO2), and cervical stability.
1. Indications for intubation include inability to protect the airway, difficulty with oxygenation or ventilation, shock, a GCS score < 9, and rapid neurologic deterioration.
2. Manual inline stabilization (MILS) should be maintained during intubation to minimize the risk of cervical spinal cord injury (SCI) for all patients in whom cervical spine injury has not been ruled out. Although recent work has persuasively questioned the value of MILS, it is still considered to be the standard of care. Modification of this technique should only be made with extreme caution by the most experienced airway managers.
3. Rapid sequence induction (RSI) should be used in patients who require emergent intubation. As with all intubations, airway managers should consider awake-topical intubation if they are uncertain about their ability to establish an airway quickly and safely.
4. For the majority of TBI patients, direct laryngoscopy (DL) is still considered to be the fastest and safest way to accomplish intubation in most patients, including those who have possible cervical spine injuries.
5. Flexible fiberoptic (FFO) and video-laryngoscopy (VL) techniques provide visualization of the larynx with less force required to establish a line of sight than with DL. Flexible fiberoptic (FFO) intubation may be useful in patients who have a difficult airway and unsecured cervical spine fractures. The technique may be limited in unstable or agitated patients and those who have blood in or significant trauma to the airway. Newer fixed fiberoptic and prism devices, including the Glidescope, Airtraq, and optical stylets, may offer better-grade views than DL when patients require MILS. These devices vary in their susceptibility to soiling and may fail when there is blood in the airway. Skilled operators who are familiar with these devices may find them helpful when DL views of the larynx are inadequate for safe intubation.
6. Laryngeal mask airway (LMA) devices, including the intubating LMA, are useful backup tools for ventilation and intubation. The intubating LMA has been shown in several studies to be a safe and reliable ventilation and intubation conduit in trauma patients. Intubation success is significantly increased when operators employ “up–down movement” and “the two Chandy maneuvers”. The up–down movement consists of moving an inserted LMA up about 6 cm and reinserting it down until a potentially folded epiglottis is repositioned. The two Chandy maneuvers are used with the Fastrach LMA (FT-LMA) and consist of (1) sagittal rotation of the FT-LMA to improve ventilation and (2) lifting the metal handle of the FT-LMA to prevent the ETT from impacting the arytenoids.
7. Surgical airway techniques, such as cricothyroidotomy and tracheotomy, are also backup methods for intubation and, in rare cases, may be the first line of management.
8. Lidocaine, 1.5 mg/kg iv given 1.5 minute before intubation, blunts the increase in ICP that occurs in response to airway manipulation.
9. The induction drugs propofol and thiopental decrease ICP, CBF, and cerebral metabolic rate for oxygen consumption (CMRO2). They also have anticonvulsant effects. However, they can also decrease MAP and cerebral perfusion pressure (CPP = MAP − ICP) to an extent that may be harmful in trauma patients. Etomidate, 0.3 mg/kg iv, may be a better choice as it produces similar decreases in ICP and CMRO2 without significant decreases in CPP. The importance of etomidate-induced transient adrenal suppression in trauma patients is being debated. Several researchers have reported favorable cerebral and general hemodynamic effects of ketamine and gamma aminobutyric acid (GABA)-agonist combinations in TBI. A recent controlled trial comparing etomidate and ketamine in a group of critically ill patients, including a substantial number of trauma and coma patients, found no difference in clinically important outcomes at any time during treatment. Nonetheless, ketamine remains controversial for TBI because it increases ICP in some patients.
10. Several neuromuscular-blocking drugs are appropriate for TBI.
a. The depolarizing muscle relaxant succinylcholine (SCH), 1.5 mg/kg, has the fastest onset of action among the currently available muscle relaxants (30 to 45 seconds). It has been shown in animal studies to increase ICP, but human studies have been inconsistent. In a controlled study in brain-injured patients, SCH’s effects on ICP were not significantly different from normal saline. SCH is contraindicated > 24 hours after TBI associated with spinal, crush, or burn injuries because of the risk of hyperkalemia.
b. High doses of non-depolarizing neuromuscular-blocking drugs such as rocuronium, 1 mg/kg, and mivacurium, 0.2 mg/kg, do not increase ICP. They have slower onset, longer duration, and fewer side effects than SCH. At standard doses, the onset of action is slower (60 to 90 seconds), which makes them less suitable for use during a true RSI. Rocuronium at 1.2 mg/kg (twice the standard intubating dose) may produce adequate relaxation in < 1 minute, making this regimen best suited for RSI when SCH is contraindicated.
11. Endotracheal intubation must be confirmed by physical examination plus a method for CO2 detection such as colorimetric or continuous capnography.
12. Chest x-rays are useful for verification of endotracheal tube position as well as identification of associated chest pathology such as pneumothorax, lung contusion, and pulmonary edema.
b. Breathing/Ventilation
1. Supplemental high-flow oxygen is provided to all patients before intubation to prevent hypoxia (PaO2 < 90 mm Hg) and provide sufficient apneic time for RSI.
2. Positive-pressure ventilation is provided as needed to maintain adequate ventilation and oxygenation.
3. After intubation, the fraction of inspired oxygen (FiO2) can be adjusted to minimize oxygen toxicity, but oxygen saturation should be maintained above levels now considered acceptable for patients who have acute respiratory distress syndrome (ARDS). Although we lack high-quality data, it is probably reasonable to maintain oxygen saturation (SpO2) at or above the mid-90s. This should be easy to accomplish in patients who do not have pulmonary contusions by keeping the FiO2 between 0.3 and 0.6.
4. A positive end-expiratory pressure (PEEP) of 5 to 10 cm H2O is generally safe in TBI patients. PEEP > 10 to 15 cm H2O may impair venous return. In hypovolemic patients, any positive-pressure ventilation may decrease cardiac output and, with that, MAP and CPP. Higher PEEP may exaggerate this effect by increasing intrathoracic pressure and interfering with right-sided filling.
5. Many TBI patients retain cerebrovascular responsiveness to changes in PaCO2. The PaCO2 should therefore be kept at near-normal levels of 35 to 40 mm Hg to maintain CBF. Patients subjected to hyperventilation demonstrate increased lactate/pyruvate ratios and other markers of cerebral ischemia. In addition, patients who have a PaCO2 < 30 or > 50 mm Hg upon presentation to the ED after intubation in the field are less likely to survive than patients whose PaCO2 is within this range. Hyperventilation is best reserved for brief periods as part of the treatment of refractory intracranial hypertension, particularly when definitive therapy such as surgical decompression is planned.
6. Sedation and analgesia, with daily interruption to assess mental status, should be provided to mechanically ventilated patients until they are ready for weaning from mechanical ventilation. The ideal sedative drug in TBI should have rapid onset and offset, anticonvulsant properties, and favorable effects on CPP. Short-acting sedatives, such as propofol, 10 to 50 mcg/kg/minute, or dexmedetomidine, loading dose 1 mcg/kg over 10 minutes; then 0.2 to 0.7 mcg/kg/hour for < 72 hours, have rapid onset and offset. This allows rapid awakening for neurologic assessment. Both drugs are problematic, however, as they can cause hypotension, and, with dexmedetomidine, this may be complicated by bradycardia. Patients receiving prolonged infusions of propofol may require many hours to awaken sufficiently for reliable neurologic examination.
Midazolam, 0.05 mg/kg bolus; then 0.03 to 0.15 mg/kg/hour, is a benzodiazepine that possesses antiseizure properties and is less likely to cause hypotension than propofol. Analgesia and blunting of stimulation associated with the endotracheal tube can be achieved with opioids such as fentanyl, 0.5 to 1 mcg/kg bolus; then 0.7 to 2 mcg/kg/hour. Patients whose tracheas are not intubated may receive hydromorphone for pain provided that close attention is paid to respiratory function. Hydromorphone is free from active metabolites, is available in intravenous, intramuscular, and oral forms, but is 7.5 times more potent than morphine including its respiratory depressant effect. Morphine and meperidine, even more so, should be avoided in renal failure as their renally excreted metabolites (including seizure-inducing ones) may accumulate and cause respiratory depression and/or seizures.
c. Circulation: Life-threatening hypovolemic or cardiogenic shock as from tension pneumothorax and cardiac tamponade is identified and either controlled or treated definitively. Systolic BP is maintained at or above 90 mm Hg. Patients may be resuscitated using intravenous fluids and/or blood. In early trauma, all shock is assumed to be hypovolemic or obstructive. Vasoactive drugs are used only after these etiologies are ruled out and less common causes, such as myocardial dysfunction or neurogenic shock, are suspected.
d. Disability: When not absolutely contraindicated by the need for immediate endotracheal intubation, the initial neurologic assessment should be performed before the administration of sedative or neuromuscular-blocking drugs. Neurologic status is assessed by using the Glasgow Coma Scale (GCS) with attention to the signs and symptoms of increased ICP and brain herniation. The GCS defines neurologic impairment in terms of eye opening and verbal and motor responses. In addition, the examiner notes the pupillary response and diameter, presence of lateral deficits, and level of spinal motor and sensory deficits. The GCS scoring system provides a quantitative measure of neurologic status, progress, and prognosis with good interevaluator agreement.
Signs of transtentorial brain herniation include unilateral pupillary dilatation, unilateral fixed lateral gaze, sudden neurologic deterioration, contralateral hemiparesis, coma, hypertension, and bradycardia. Emergency therapy includes reassessment and treatment of extracranial insults such as hypoxia and shock, elevation of the HOB to 30°, mannitol infusion, brief hyperventilation, and surgical decompression.
e. Exposure: The patient is fully undressed and examined for any associated injuries while precautions are taken to avoid hypothermia.
2. Secondary survey
a. The secondary survey includes a more complete history and physical examination as well as laboratory and ancillary testing to diagnose the extent of TBI and associated injuries.
b. Commonly requested investigations include x-ray examination of the chest and pelvis, complete metabolic panel, complete blood count, clotting parameters (prothrombin time and partial thromboplastin time [PT/PTT]), serum osmolality, urinalysis, ethanol level, urine drug screen, and blood type and screen.
c. Unless contraindicated by need for immediate laparotomy or other procedure to prevent death from shock, all TBI patients should have a non-contrast computed tomographic (CT) scan of the head and cervical spine as soon as possible. In addition, a CT scan of the abdomen is often necessary.
d. If immediate craniotomy, laparotomy, thoracotomy, or other interventional procedure is required, the concurrent placement of an IVC for monitoring and treating ICP should be discussed with the neurosurgeon. Epidural and subdural hematomas that demonstrate significant mass effect are more amenable to surgical intervention than are other intracranial lesions.
D. Critical care management after initial resuscitation
1. ICP monitoring
a. Indications for placement of an ICP monitor include an abnormal CT scan and GCS score of 3 to 8 after adequate resuscitation of shock and hypoxia or a normal CT scan and GCS score of 3 to 8 accompanied by 2 or more of the following: age > 40, posturing, or systolic BP (SBP) < 90 mm Hg.
b. ICP monitoring using an intraventricular catheter is preferred because it provides dependable readings and allows therapeutic drainage of CSF.
2. Treatment to reduce ICP and enhance CPP should be correlated with the patient’s neurologic examination, overall physiologic status, and state of CBF autoregulation. With TBI-induced loss of autoregulation, CBF becomes MAP dependent, which increases the risk of both cerebral ischemia when the MAP decreases and hyperemia (and edema) when the MAP goes up. If an ICP monitor is not in place, treatment aimed at decreasing ICP should be initiated when either neurologic deterioration occurs or signs of brain herniation are present.
a. Recommendations for optimizing CPP and ICP vary. The “Lund” approach posits that the integrity of the blood–brain barrier (BBB) for salts is lost after TBI so that the goal of management is to limit the resulting edema and ICP elevation by using colloid with diuretics and maintaining ICP < 20 mm Hg. A CPP of as low as 50 mm Hg is acceptable if necessary to control ICP. The Rosner approach is based on the observation that cerebral autoregulation in the injured brain can often be present at a MAP of as high as 100 to 110 mm Hg. As MAP is increased beyond the normal limit of autoregulation, ICP falls and CPP rises. Consequently, the achievement of higher MAP and CPP is emphasized.
In fact, the optimization of MAP and CPP is best based on individual patient pressure-reactivity curves. A 2005 study classified patients as having defective or intact autoregulation based on whether ICP rose more than 2 mm Hg in response to a 15 mm Hg increase in MAP (ICP/MAP slope > 0.13). The authors found that the pressure-passive, or defective, autoregulation patients (ICP/MAP slope > 0.13) seemed to benefit from a lower ICP treatment threshold (20 mm Hg) and lower target CPP (50 to 60 mm Hg). In contrast, pressure-active, or intact, auto-regulation patients (ICP/MAP slope < 0.13) appeared to benefit from a higher ICP treatment threshold (25 to 30 mm Hg) and higher target CPP (> 70 mm Hg). Although the BTF recommends maintaining CPP > 70 mm Hg in patients who have suspected loss of autoregulation, a recent study found that optimizing CPP for individuals at somewhere between 60 to 100 mm Hg maximized their brain tissue oxygenation (PbtO2). The PbtO2 worsened above or below this range.
b. Therapy aimed at decreasing ICP includes elevation of the HOB to 15° to 30°, control of seizure activity, ventilation to low-normal PaCO2 (35 to 40 mm Hg), maintenance of normal body temperature, release of any obstruction to jugular outflow (such as circumferential endotracheal tube tape), assurance of optimal fluid resuscitation and overall physiologic status, and administration of sedatives and neuromuscular-blocking drugs as needed. If these measures fail to decrease ICP, additional therapies are provided in a first- and second-tier stepwise manner.
1. First-tier therapy
a. Incremental CSF drainage via an IVC
b. Diuresis with mannitol, 0.25 to 1.5 mg/kg over 10 minutes. Mannitol improves CBF by decreasing blood viscosity and transiently decreases ICP by decreasing brain edema. However, mannitol-induced diuresis may cause hypotension, especially in the early resuscitative phase when invasive monitoring is not yet in place and the extent of associated injuries is unknown. Therefore, euvolemia or mild hypervolemia is maintained and serum osmolarity is monitored and maintained < 320 mOsm/L during mannitol therapy. An alternative or supplement to mannitol is hypertonic saline, given in various concentrations and doses. Investigators found favorable effects on ICP and PbtO2
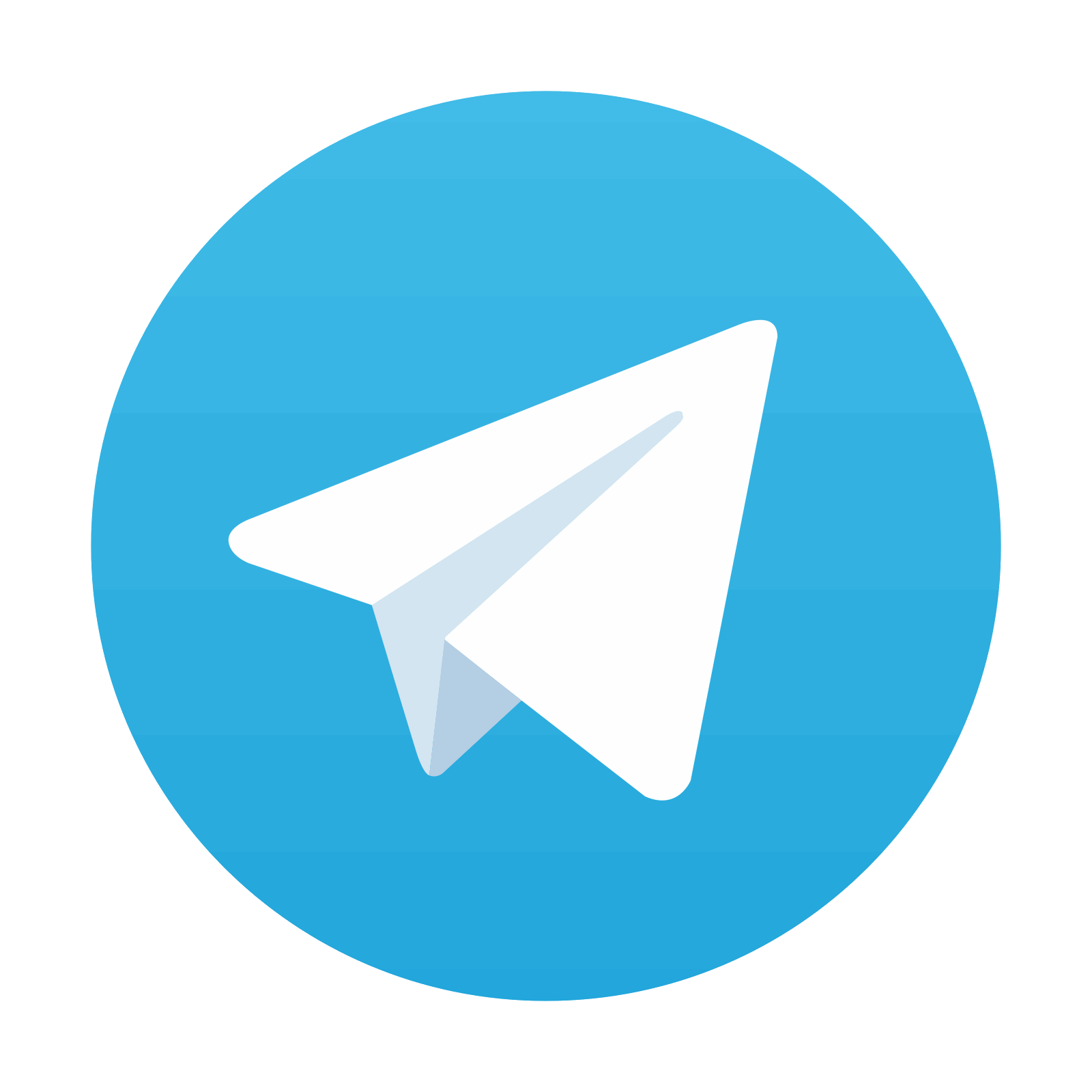
Stay updated, free articles. Join our Telegram channel

Full access? Get Clinical Tree
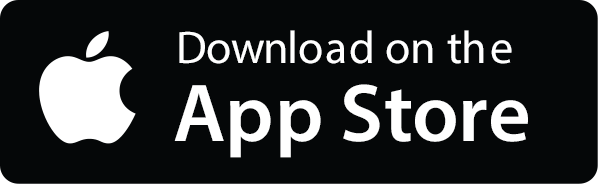
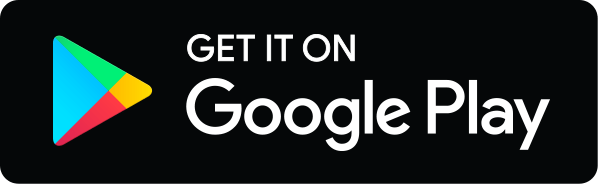