CLINICAL PEARL
In-hospital treatment of TBI should focus on minimizing secondary insults to the injured brain due to hypotension, hypoxemia, hypercarbia, hypocarbia, hyperthermia, intracranial hypertension, seizures, hypoglycemia, and hyperglycemia.
A. Cerebral metabolic rate, cerebral blood flow, and cerebral autoregulation. Global cerebral metabolic rate (CMR) for oxygen and glucose is higher in children than in adults (oxygen 5.8 vs. 3.5 mL/100 g brain tissue/min and glucose 6.8 vs. 5.5 mL/100 g brain tissue/min respectively). Unlike in adults, cerebral blood flow (CBF) changes with age and may be higher in girls compared to boys. Following TBI, CBF and cerebral metabolic rate for oxygen (CMRO2) may not be matched, resulting in either cerebral ischemia or hyperemia. The incidence of cerebral hyperemia is 6% to 10% and CMRO2 may be normal, low, or high after TBI [6]. Metabolic failure is an integral component of the pathologic aftermath of TBI. In pediatric TBI patients, low oxygen extraction fraction (OEF)—a marker of metabolic dysfunction—correlates with the severity of injury and outcome [9]. Similar to adults, the incidence of impaired cerebral autoregulation is higher following severe compared to mild TBI and children with impaired cerebral autoregulation early after TBI may lead to cerebral ischemia and poor long-term outcome [10].
B. Intracranial pressure. In adults, normal ICP is between 5 and 15 mm Hg compared to 2 to 4 mm Hg in young children. Unlike the adult with relatively poor cranial compliance, the infant with open fontanelles may be able to accommodate slow and small increases in intracranial volume by expansion of the skull. However, rapid expansion of intracranial volume, small as it may be, can explain the not uncommonly encountered rapid deterioration in infants following TBI. Intracranial hypertension may be difficult to diagnose, is associated with poor neurologic outcomes and death, and may be present in children with open fontanelles and sutures.
CLINICAL PEARL
TBI often leads to uncoupling between CBF and metabolism and leads to impairment of cerebral autoregulation. Hyperemia after pediatric TBI is relatively uncommon whereas cerebral ischemia is more common.
IV. Imaging modalities. The initial radiographic evaluation of the pediatric patient with TBI is performed using computed tomography (CT) imaging which can rapidly detect intracranial hematoma, contusion, skull fracture, cerebral edema, and obliteration of the basal cisterns indicating concern for elevated ICP. However, prior to transport to CT scanner, initial evaluation and resuscitation must be performed and appropriate monitoring must be instituted. Patients with DAI may initially have a normal CT scan despite significant neurologic findings and increased ICP; repeat CT scan often shows secondary injury due to cerebral edema [11]. In childhood, conventional MRI compared to CT scan has high sensitivity and specificity, better correlation with the outcome, and lack of radiation [12,13]. Although conventional MRI sequences are able to depict the precise anatomical location, extent, quality, and degree of TBI, functional MRI (fMRI) sequences give information about the microstructural (diffusion-weight imaging [DWI]/diffusion tensor imaging [DTI]), biochemical (magnetic resonance spectroscopy [MRS]), and hemodynamic perfusion-weighted imaging (PWI) short- and long-term consequences, and complications of TBI [12,13]. However, MRI is typically not obtained during the initial period because of its time-consuming imaging requirements. In addition, there are growing concerns about cumulative radiation exposure during CT scanning, and the current guidelines for the acute medical management of severe TBI in infants, children, and adolescents provide a level III recommendation that in the absence of neurologic deterioration or increasing ICP, obtaining a routine repeat CT scan >24 hours after the admission and initial follow-up study may not be indicated for decisions about neurosurgical intervention.
CLINICAL PEARL
Head CT imaging is often required to assess the extent and type of TBI. However, early MRI may be necessary to fully understand the extent of TBI. Routinely repeated head CT scanning is not advised due to ionizing radiation risks to the developing brain and other sensitive organs.
V. Indications for surgery. The major goal of surgery for TBI is to optimize the recovery of viable brain. Most operations deal with the removal of mass lesions for the purpose of preventing herniation, intracranial hypertension, or alterations in CBF. In general, unless small and venous, EDHs should be evacuated in comatose patients. SDHs that are associated with herniation, are greater than 10 mm thick, or produce a midline shift of >5 mm should be removed. Indications on intraparenchymal mass lesions include progressive neurologic deterioration referable to the lesion, signs of mass effect on CT, or refractory intracranial hypertension. Penetrating injury may often be managed with local debridement and watertight closure if not extensive and if there is minimal intracranial mass effect (as defined above). Patients with severe brain swelling as manifest by cisternal compression or midline shift on CT or intracranial hypertension by monitor are potential candidates for decompressive craniectomy. The relatively increased frequency of diffuse swelling in the pediatric population makes children more frequently candidates for such treatment.
3
VI. Anesthetic and perioperative management. The cornerstones of modern TBI management are field resuscitation, expeditious triage, emergent surgical evacuation of mass lesions, control of ICP, and support of CPP, multimodal monitoring and optimization of physiologic environment. Given the adverse impact of secondary insults on outcomes of TBI, the perioperative period may be particularly important in the course of TBI management. Despite the aggressive interventions to rapidly correct the secondary insults in the ED, it is not unusual for one or more of these complicating factors to persist or remain undetected as the patient is emergently transported to the operating room. Hence, perioperative period is an opportunity for the anesthesiologists to correct the pre-existing secondary insults. Moreover, surgery and anesthesia may predispose the patient to new onset secondary insults (such as intraoperative hypotension and new onset hyperglycemia), which are preventable/treatable. Therefore, the perioperative period may be a potential window to initiate interventions that may improve the outcome of TBI. Perioperative management involves rapid evaluation, continuation of cerebral and systemic resuscitation, early surgical intervention, intensive monitoring, and anesthetic planning.
CLINICAL PEARL
Surgery and anesthesia may predispose the patient to new onset secondary insults which are preventable/treatable. Anesthesiologists should anticipate and be prepared to rapidly treat these secondary insults.
A. Initial assessment. The initial approach to the traumatized child involves the primary and secondary surveys. Briefly, this involves rapid assessment of the airway, breathing, circulation, neurologic status, and associated injuries. The Glasgow Coma Scale (GCS) score (modified for children) is the most commonly used neurologic assessment (Table 29.2) and for grading the severity of TBI. A GCS score of 13 to 15 signifies mild TBI, 9 to 12 is moderate TBI, and 3 to 8 is severe TBI. Signs and symptoms of intracranial hypertension or impending herniation, such as altered level of consciousness, pupillary dysfunction, lateralizing extremity weakness, or Cushing’s triad (hypertension, bradycardia, and irregular respirations) should alert the need for urgent interventions to control ICP including possible surgical decompression. Bruising around the mastoid (“Battle’s sign”) and in the periorbital area (“Raccoon eyes”) and rhinorrhea indicate possible basilar skull fracture. The initial assessment and stabilization is usually achieved in the ED and resuscitation initiated before the patient is transported to CT scanner and then to the operating room. Nevertheless, it is important for the anesthesia team to perform another rapid assessment as the patient is received in the operating room. Associated thoracic, abdominal, spinal, and long bone injuries may be stable or evolve during the perioperative period and must be considered in differential diagnosis of new onset hypotension, anemia, hemodynamic instability, or hypoxemia during anesthesia and surgery. As the patient is transported to the operating room, all resuscitative measures should continue.
Table 29.2 Glasgow coma scale and modification for young children
CLINICAL PEARL
Initial assessment of pediatric TBI victim involves rapid assessment of the airway, breathing, circulation, GCS score, pupillary response, and associated injuries. Cushing’s triad (hypertension, bradycardia, and irregular respirations) indicates impending herniation.
1. Cervical spine immobilization. In infants <6 months of age, the head and cervical spine should be immediately immobilized using a spine board with tape across the forehead, and blankets or towels around the neck. In infants ≥6 months of age, the head should be immobilized either in the manner described above or by using a small rigid cervical collar. Children >8 years of age require a medium-sized cervical collar. The use of rigid cervical collars is essential as it prevents cervical distraction during laryngoscopy. Since children under 7 years of age have a prominent occiput, a pad placed under the thoracic spine provides neutral alignment of the spine and avoids excessive flexion that may occur in the supine position. These two maneuvers are paramount in avoiding iatrogenic cervical spine injury.
2. Airway management. The most important therapy during the primary survey phase is to establish an adequate airway. The lucid and hemodynamically stable child can be managed conservatively but if the child has altered mental status, attempts should be made to establish the airway by suctioning the pharynx, chin-lift and jaw thrust maneuvers, or insertion of an oral airway. Children requiring surgery and those with a GCS score <9 require tracheal intubation for airway protection and management of increased ICP. Airway management in such circumstances is complicated by a number of factors including urgency of situation (because of pre-existing/worsening hypoxia), uncertainty of cervical spine status, uncertainty of airway (due to presence of blood, vomitus, debris in the oral cavity or due to laryngopharyngeal injury or skull base fracture), full stomach, intracranial hypertension, and uncertain volume status. All TBI patients requiring tracheal intubation must be considered to have full stomach and airway management must account for possible underlying cervical spine injury. In the patients who arrive with an indwelling tracheal tube, adequate position of the tube must always be confirmed since the tube can possibly migrate during transport, leading to endobronchial intubation or even dislodgement.
The choice of technique for tracheal intubation is determined by urgency, individual expertise/skills, and available resources and generally incorporates rapid sequence orotracheal intubation with cricoid pressure and manual in-line stabilization. The anterior portion or cervical collar may be removed when manual in-line stabilization is established to allow greater mouth opening and facilitate laryngoscopy. Newer airway devices, particularly GlideScope video laryngoscope, have gained popularity in recent years for use in trauma victims and may be useful in difficult airway scenarios. However, recent studies show no difference in first-attempt success rates between video laryngoscopy and direct laryngoscopy in the newborn or infant simulators for pediatric emergency medicine providers although video laryngoscopy led to increased percentage of glottic opening (POGO) scores [14]. In any case, it is advisable to have a backup plan ready in case of difficult intubation, given the risk of intracranial hypertension resulting from increased cerebral blood volume (CBV) because of hypoxemia and hypercarbia during prolonged intubation attempts.
The choice of induction agents and muscle relaxants is important for successful uncomplicated airway management. Tracheal intubation is a noxious stimulus and can increase ICP. Hence, sodium thiopental (3 to 5 mg/kg), etomidate (0.2 to 0.6 mg/kg), or propofol (2 to 3 mg/kg) are often used to induce anesthesia before intubation in hemodynamically stable patients. All these agents decrease the systemic hemodynamic response to intubation, blunt increases in ICP, and decrease the CMRO2. In addition, administration of lidocaine (1.5 mg/kg) and short-acting narcotic such as fentanyl (1 to 2 μg/kg) can decrease the catecholamine release associated with direct laryngoscopy. However, propofol and thiopental may cause cardiovascular depression leading to hypotension, especially in the presence of uncorrected hypovolemia. Etomidate (0.2 to 0.6 mg/kg) may be advantageous particularly in patients with unstable hemodynamic status due to little change in blood pressure during induction despite reduction of CMRO2. Pediatric data on adrenal insufficiency following single-dose etomidate in TBI patients are lacking, although adrenal depression has been observed following etomidate use in children with sepsis [15]. Ketamine, which causes limited cardiovascular compromise, is often considered relatively contraindicated for intubating patients with risk for or pre-existing increased ICP for the fear of associated increased CBF and increased ICP. However, in mechanically ventilated pediatric patients with intracranial hypertension, ketamine has actually been shown to effectively decrease ICP and prevent untoward ICP elevations during potentially distressing interventions without lowering blood pressure and CPP [16]. In fact, ketamine may be a safe and effective drug for patients with TBI and intracranial hypertension and it can possibly be used safely in emergency situations. The choice of muscle relaxant for rapid sequence induction is between succinylcholine and rocuronium. While the clinical significance of the effect of succinylcholine on increasing ICP is questionable, increases in ICP secondary to hypoxia and hypercarbia are well documented and much more likely to be clinically important [17]. Hence, in patients with TBI, clinicians may not avoid using succinylcholine if difficult intubation is anticipated.
CLINICAL PEARL
Patients with GCS <9 require tracheal intubation to protect airway and to control ICP. Rapid sequence orotracheal intubation should be performed with cricoid pressure and manual in-line stabilization. Etomidate and ketamine may be used safely in TBI patients with unstable hemodynamics, if needed.
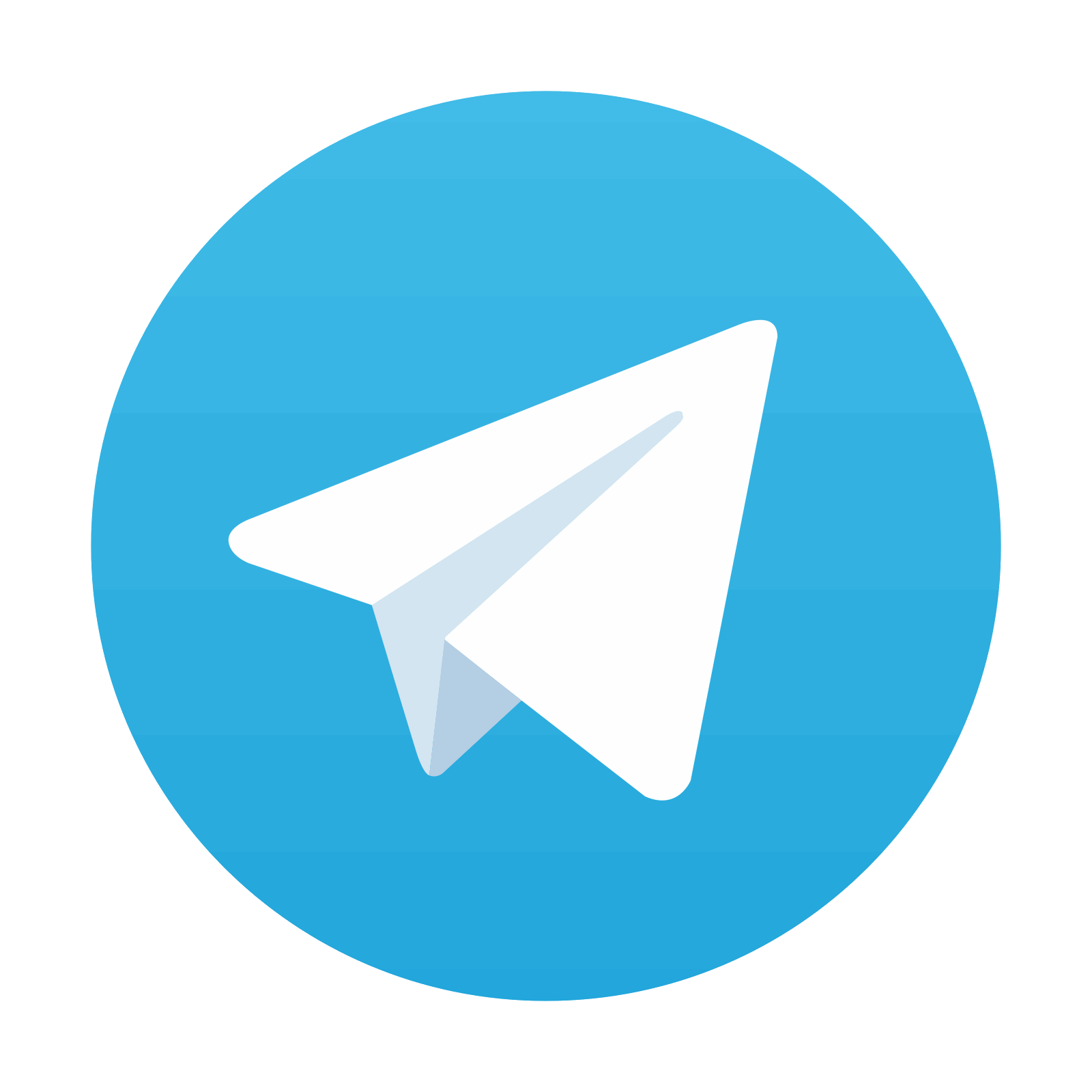
Stay updated, free articles. Join our Telegram channel

Full access? Get Clinical Tree
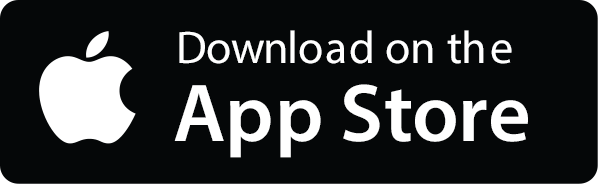
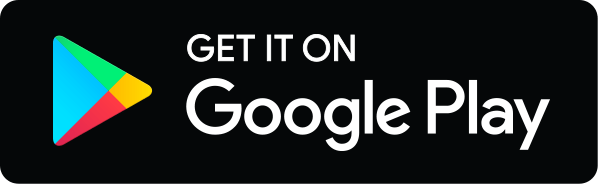