Fig. 33.1
Extravehicular activity during STS-117 to repair the damaged thermal blanket using a surgical staple gun
Parabolic Testing
Numerous procedures were validated in human and animal studies on the microgravity aircraft. The parabolic microgravity program uses an aircraft to simulate low gravity states induced by parabolic flight. The aircraft pulls up into a 45° nose high attitude and climbs about 5,000 ft. At that point, the aircraft pushes the nose over to about 45° nose low (Figs. 33.2 and 33.3). At the top of the parabola, a microgravity state is induced for about 20–30 s. The maneuver is repeated 30–40 times in order to simulate spaceflight conditions for equipment and procedure validation. The problem is that it is only a short duration of time to apply the procedures, but this is the only adequate simulation of the microgravity state available currently. Both the Russian and US space programs have used parabolic flights to evaluate medical procedures and equipment. In 1967, the Russian space program evaluated laparotomies on parabolic flights. The system utilized a transparent surgical canopy with a magnetic instrument holder. It was noticed that the procedures went fairly well. The one complication noted was that arterial bleeding formed droplet streams that spread throughout the containment area decreasing visualization. Bowel evisceration during laparotomy could also obscure the visual field [
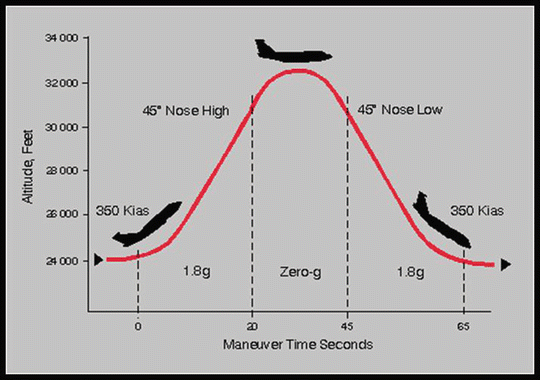
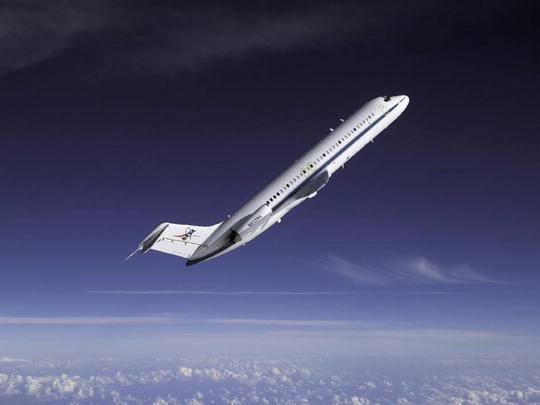
24]. The US parabolic experimental program found that surgical procedures could be performed with only minor modifications. The requirement for restraining the patient, surgical hardware, and the surgical personnel had to be stringently observed [25]. The patient, even if awake for a minor surgical procedure, must remain restrained. The operating personnel must also be limited in motion from the waist down, but leave the arms and hands free of obstructions.
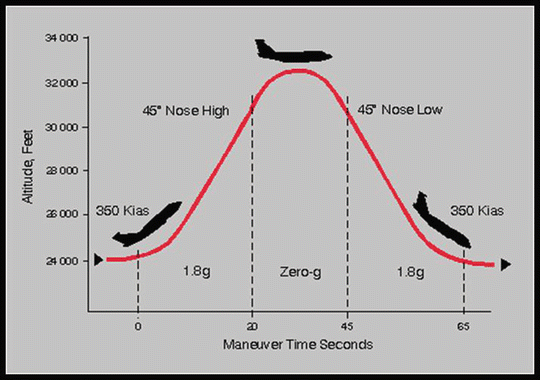
Fig. 33.2
NASA parabolic flight profile
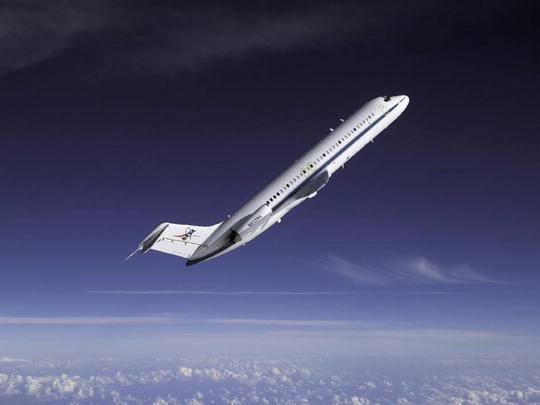
Fig. 33.3
NASA C-9 microgravity aircraft
Bleeding and free blood could be contained with common surgical methods of sponges and suction, but must be addressed immediately [26]. All phases of surgical procedures have been evaluated. Prepping, draping, equipment prep, and restraining have all been proven in microgravity. Lighting and enclosed environments have also been validated. Again, the emphasis has been on adequate restraint of the equipment. Glove packages must have tape on the backing to secure them, and commercially available surgical drapes with an adhesive band proved to be excellent solutions for microgravity operations. Conventional antiseptics had enough surface tension to remain in place as well [27–29].
It was found that procedure-oriented kits were preferable to individually packaged instruments. Extra packaging became an encumbrance. Standard Velcro, elastic cords, and magnetic surgical tables helped to contain the instruments. Sharp blocks (a block of Styrofoam for sharps), an adhesive pad, and a plastic bag near the surgical table or as a pocket on a gown were used to facilitate rapid trash disposal. Maintaining an organized surgical table and rapid trash disposal are critical in the microgravity arena. This facilitated speeding the procedures and maintaining a good visual field [29]. The ISS uses a floor-level restraint system that was developed from similar devices flown on the Space Shuttle and Russian Mir space station. The CMRS (Crew Medical Restraint System) can act also as a backboard for patient transport if needed. It is easily stored and has built-in belts to restrain patients and operating personnel. There is even a built-in cervical restraint system.
Surgical Field Testing
One of the major impedances to surgical care in microgravity (low Earth orbit) or zero gravity (away from planetary gravitational forces) is the atmospheric contamination of the vehicles’ atmosphere with blood or other body fluids. The closed-loop systems could easily be overwhelmed with surgical debris and blood [30]. The spacecraft also presents a hazard to the surgical site. The clean vehicle at launch accumulates dross that increases the risk of contamination of the wound area. Particles tend to be larger and contain dust, food particles, and sloughed skin elements. In a limited space environment, the surgical zone may be in close proximity to the galley or exercise facilities. Also there can be numerous scattered areas or waste disposal. This may aid in surgical trash disposal, but it would also be a potential source of contamination for the procedure. Long-duration spaceflight has shown to produce immunosuppression and altered immune responses [31, 32]. Impaired healing of wounds has also been seen. A system for contaminant containment for the field and prevention of infectious contamination of the surgical site are requisite requirements (Fig. 33.4).
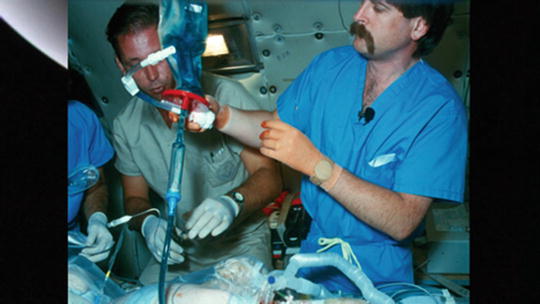
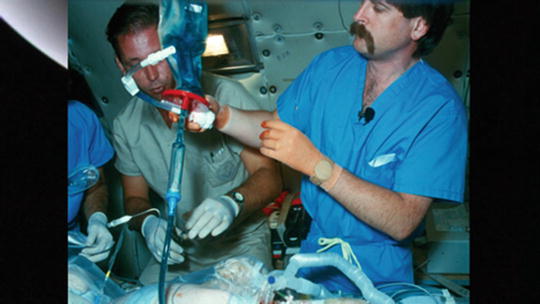
Fig. 33.4
Enclosed surgical field testing on NASA microgravity aircraft
The Russian space community built several enclosed systems and tested them in parabolic flights [24, 32]. In 1978, an inflatable Lexan surgical enclosed bubble was proposed by Mutke [33]. Markham and Rock tested several inflatable enclosures and simulated surgical procedures in parabolic flight [34–36]. These proved to be quite successful at containing floating instruments, solids, and fluids. NASA tested a similar inflatable canopy [32]. Anesthetized animals were used as surgical subjects, and the systems were evaluated for ease of use, portability, and containment. It was noted during these experiments that venous bleeding appeared to be increased. It was surmised to be due to an inability of the venous walls to provide compression in microgravity. Unopposed surface tension caused both arterial and venous areas of bleeding to form large fluid domes [26]. Arterial bleeding was not entrapped by sponges or suction when a large stream of droplets was formed. The overhead canopy placed over the surgical area would be useful in containing uncontrolled bleeding or if copious irrigation was required. Another method of containing contaminants in a surgical field is the use of laminar flow devices. The airflow would direct the fluid or other detritus into a downstream suction collection device. NASA also tested this concept in parabolic flight. Bleeding and debris not restrained by local methods were swept away from the visual field directly over the open wound. Still most bleeding was controlled by surgical sponges, gauze, or suction directly at the site.
Surgical and Advanced Life Support Procedures
NASA conducted numerous parabolic flights to test operative and resuscitative techniques in a microgravity environment. These tests were conducted to see what procedures could be done, how the equipment behaved, and if the procedures could be done with minimal equipment. The crew medical officer (CMO) for each journey may be a nonphysician. Cardiopulmonary resuscitation (CPR) was found to be more difficult in lower-gravity environments, but can be accomplished. It was reinforced that restraint of both the subject and the rescuer was critical in most situations. The “handstand” procedure of placing one’s feet on the wall opposite to the victim’s chest and placing the CMOs hands over his head and on the chest was found to be effective. Also the Heimlich method of standing behind the victim and performing CPR was validated as providing adequate CPR [37–39]. Ventilation support with artificial ventilators was performed, and the parameters were unchanged from the terrestrial environment. Similarly, respiratory mechanics on ventilatory support was not clinically different [40].
ALS (Advanced Life Support) procedures of venous cutdowns, cricothyroidotomies, peritoneal lavages, and chest tube insertions were also evaluated. These proved to be more difficult than in normal gravity. Fluid infusions and drainage systems required modifications to the techniques. Intravenous fluids had to have all the free air removed and required fluid pumps to infuse the fluids. Pressurizing the fluids with external pressure bags did work well. Drainage lines had to be as short as possible and large in diameter to prevent surface tension and capillary action from inhibiting flow. Percutaneous peritoneal lavage was more dangerous. The GI tract is more dilated due to decreased peristalsis in low-gravity environments. This resulted in additional pressure of the bowel on the abdominal wall and increased the risk of perforation. A modification of the technique would be required to increase the air in the abdominal cavity to decrease this likelihood. Another complicating factor was that the increased fluid tension and lack of capillary fluid pull lead to decreased lavage fluid drainage. An advantage to this procedure is that it required less training than their open lavage counterpart. Open peritoneal lavage techniques were accomplished, but required more training and the use of surgical canopies.
Chest tube insertion was also performed on the surgical evaluation parabolic flights. The equipment employed incorporated a Heimlich valve and a Sorenson drainage system. This proved to provide adequate drainage and eliminated the risk of contamination from the vehicle environment. Another advantage was that the blood from a hemothorax in this type of system could be used to autotransfuse a trauma victim [41–43]. A percutaneous Seldinger dilatation method proved easy to train, required only a minimal amount of equipment, and did not require a dedicated surgical field. Suture ties around the chest tube site to secure the tube had to perform several functions and were critical in the procedure. Studies have shown that suturing in microgravity is similar but slower than normogravity [44]. They had to retain the tube in position and control fluid and blood leakage. Chest tube drainage generally did not require suction, because the intrathoracic pressure provided the push to the drainage system. The advantage of a chest tube placed in microgravity was that the hemothorax fluid was equally distributed along the chest wall in an adherent sheet rather than pooling in a dependent location. Some loculation did occur though by surface tension, but overall was not as critical as in a one-G environment. The procedure only encompassed about 1 h of ground-based instruction and could possibly be done by computer-based training (CBT). Telemedicine guidance of peritoneal lavage and chest tube insertion could also be done by a remotely located surgeon.
Other surgical procedures have been performed in the simulated microgravity environment. Leg dissection, thoracotomy, laparotomy, and craniotomy were simulated. Specifically craniectomy, C-section, and laminectomy were conducted on the STS-90 Neurolab flight [45]. Other procedures included ureteral stenting, thoracoscopy, and microsurgery [46–48]. The evaluation of surgical skills in these major procedures demonstrates equivocal results. Subjective evaluation suggests that surgical procedural performance decreases when the operator is not acclimated to the microgravity environment which may further lead to task erosion and subsequent tissue injury.
Numerous evaluations of endoscopy and laparoscopy have been performed [45, 47, 49, 50]. The concerns observed during these procedures were the potential for impaired visualization due to lack of bowel retraction from gravity. Also floating debris of blood and detached materials could impede the visual field. The elastic mesenteric tethering of the bowel in position and fluid surface tension caused surgical detritus to adhere to the abdominal wall. Parabolic flights proved insightful with animal subjects used to simulate the human equivalent. The endoscopic tools and procedures proved to be feasible and comparable to the one-G environment. Abdominal laparoscopy has the advantage of providing a natural surgical canopy by the subject’s own body.
The weight of the abdominal wall causes the abdominal cavity to form a flattened oval. This oval in microgravity is changed to a more rounded shape. This leads to an increase in the anterior to posterior diameter and increases the visual field. This actually facilitates minimally invasive abdominal procedures. Other advantages of minimally invasive surgeries are preventing contamination of the cabin with biological particulates, preventing abdominal contamination, maintaining thermal regulation, and possible blood collection and autotransfusion [51, 52].
Minimally invasive surgeries (MIS) have several drawbacks. Thoracoscopy presents a different set of problems from laparoscopy. In the microgravity environment, the retraction of the mediastinum by gravity is not present. This causes anatomical obscuration of the visual field. Selective bronchial intubation, pulmonary segmental deflation, and chest insufflation would be required to make a thoracoscopic procedure viable. It is very difficult to establish a pneumoperitoneum without direct visualization of the abdomen and has a high risk of bowel perforation. Minimally invasive surgeries still remain limited in the management of multi-trauma cases [53, 54]. There are numerous publications that have raised the question on the use of MIS in critically ill patients [55–57]. This raises questions if an injured astronaut can tolerate the increased intra-abdominal pressures required for MIS [58]. Astronauts have reduced red cell counts and plasma volumes and decreased cardiac output which put the crewmembers at risk for injury and may be compounded with the stresses of laparoscopy.
In any minimally invasive surgical procedure, the CMO would need to have the training, ability, and experience specific to endoscopic procedures in order to perform them. Highly trained surgeons with considerable proficiency would be required to perform these procedures. Also significant deskilling occurs when a surgeon does not continually practice these procedures. It would require a simulated surgical environment to accompany the mission to remain proficient in these skills. Several studies have been undertaken to reduce the required training and skill-level retention to accomplish minimally invasive surgical procedures. Broderick et al. investigated simulating hand-assisted laparoscopy in parabolic flights [51]. Dulchavsky has also proposed the method of using mini-laparoscopes for peritoneal drainage with telemonitoring [47].
New methods and equipment have been developed making space surgery a more viable and easier option. Miniaturization of laparoscopic equipment has made the possibility of these procedures even more viable. Large amounts of supporting equipment are no longer required for most procedures. A portable computer had substituted for large bulky video monitors, and fiber optics can provide adequate lighting and visualization of the field. Stereoscopic three-dimensional displays are coming on line that can be incorporated into virtual reality headgear. Abdominal wall lift devices are in development that may eliminate the need for CO2 or N2 insufflation [59]. This would allow the abdominal wall to be retracted anteriorly improving the visual field and allow the mesenteric attachments to hold the bowel in place. The change in the abdominal wall shape is different with lift devices than with insufflation. The viscera have a higher propensity to float and obscure the visual filed in the intra-abdominal compartment [60]. Controlling hemorrhage is made easier by fibrin glue injectors, laser scalpels, and advanced stapling devices. Tissue sealants and fibrin glues have been compounded into a foam and easily applied. These have been found to be more effective than surgical packing currently in use [61]. A device now is in development that can detect vascular flow prior to cutting and suturing. This uses pulsatile light absorption characteristics of hemoglobin to noninvasively characterize hidden blood vessels. By using a multichannel LED/sensor pair that employs NIR and red light, blood vessels can be detected and vessel size determined. This would help avoid unintended cuts to the vasculature caused by poor visibility in a minimally invasive procedure. Such a device prevents the obscuration of the visual field by streams of blood caused by inadvertent vessel injury [62].
Accessing disease pathology via an intraluminal route is a revolution in medicine. This has been demonstrated in trauma, vascular surgery, and cardiology care. Access to the central circulation also enhances hemodynamic support and measurements and can enhance guided interventional angiographic therapies. It also can provide an extremely efficient method to administer specific pharmacologic treatments. Via central venous access, inotropes and vasopressors can be administered safely. Vasopressors may be required in the treatment of space-adapted physiology. Heparin-bonded extracorporeal circuits are used in multisystem trauma for both rapid rewarming and also facilitating hemodynamic support [63, 64]. Doppler guided needles are currently available and smart ultrasound-guided “bibs” that use automated algorithms for vascular identification are being developed [65, 66]. In the future, full robotic control may be possible for cannula insertion and diagnostic assessment. This offers some real advantages in deep exploration missions and may reduce the training required for these missions.
Telerobotics and telepresence are offering an increase in precision and the ability to operate from long distances. They offer the capability to enhance the images and dexterity of the individual surgeon. Telemedicine also has the capability to provide consultation and even surgical procedures to be performed from a distance. Investigations have shown that operating across continents and to undersea environments can be accomplished [67, 68]. While these techniques might be practical in low Earth orbit, they would be inhibited by communication delays outside of that realm. A Mars mission could not avail itself of telemedicine. Electronic delays from 8 to 40 min would render this option impossible.
Surgical Procedures in Space
The Space Shuttle Neurolab mission on STS-90 conducted the first surgical procedures on animals in orbit. A leg wound was created and then closed with Dermabond adhesive. Several other procedures were conducted on that mission and provided insight and verified parabolic experiments of surgical procedures in space. The mission confirmed that the procedures were only as difficult as ones performed on Earth. Spaceflight experience also demonstrated that there were no obvious changes in manual dexterity, proprioception, or fine motor control of the hand. It reinforced the principle that restraint of the operator, patient, and surgical equipment was critical. It took a diligent effort to contain equipment in place and immediately discard any trash. Procedures took longer because of the need to assure restraint of the equipment. It was noticeable that fluids coalesced and surface tension predominated in microgravity. Sponging and suction at the site of bleeding controlled the environment and allowed continued visualization of the field. Scalpels and needles required special care, but that restraint on Styrofoam blocks proved adequate. If not restrained, loose needles needed to be called out and identified immediately in order to prevent accidental punctures.
Diagnostics
The physiological alterations that occur in the spaceflight environment will impact disease presentation, diagnostic evaluation, treatment, and management. Ultrasonography has proved to be the most valuable diagnostic tool in spaceflight. Exams such as the extended focused assessment with sonography for trauma (eFAST) to evaluate the need for emergent surgical intervention have been proven on orbit [69–73]. Ultrasound (US) is dependent on gravity to locate free fluid in the thorax and abdomen. Thus far surface tension has caused these to form on orbit, but may not be counted on in all situations. Ultrasound evaluation has been shown to delineate several conditions, including pneumothoraces and sinus fluid levels [72, 74, 75]. The development of three-dimensional US displays has led to improvements in analysis of injuries in acute abdomens. It can better quantify volumes of fluid when compared to two-dimensional US [76, 77]. US contrast media may also be used to help quantify and follow any hemorrhages in real time [78]. Future developments may include the use of integrated computer programs to help predict the progression of an injury. Hoyt’s research predicted survivable outcomes based on terrestrial volumes of hemorrhage to be 2 h if the bleeding was determined to be 25 ml of bleeding per minute. If bleeding exceeded 100 ml/min, a mortal outcome could be expected in 30 min if bleeding was uncontrolled [79]. Programs could be generated based on the physiological changes encountered in spaceflight.
US can also be utilized as an interventional device. It has been utilized for guided percutaneous aspiration of intraperitoneal fluid using a swine model in microgravity [80]. US requires user-dependent skills and requires dedicated training for the onboard operators. Current efforts guide less-experienced users from Mission Control using skilled experts who remotely mentor the onboard astronauts. This will require onboard trained ultrasonographers for deep space missions [81, 82]. NASA scientists have created a research program to facilitate minimally trained crew medical officers to use US autonomously and/or using remote guidance [71, 82]. US offers the greatest potential currently, but miniaturized CT or MRI scanners are also being developed that can be adapted for space travel. These will require specialized operators or automated diagnostic tools for immediate analysis. More than likely images can be transmitted back to consultants for analysis. Thus both diagnostic evaluation and interventional techniques could be performed in microgravity with the use of ultrasound-dependent techniques. A noninvasive way to perform surgery is by focused ultrasound. This can be used to ablate or emulsify soft tissue tumors in the tissues of the abdomen, brain, and heart. It offers a method to also treat benign prostatic hyperplasia and possibly bone cancer. Ultrasonic atomization causes mechanical tissue disruption by boiling histotripsy. Ultrasonic atomization occurs by having an ultrasound wave in liquid encountering an air interface. This interaction ejects small liquid droplets into the air. Boiling histotripsy was developed at the University of Washington that uses high-intensity focused ultrasound (HIFU). This HIFU is then used to fractionate tissue into its submicron components. The HIFU rapidly heats tissue as the transducer focuses to form a millimeter-diameter bubble of boiling material in milliseconds. This fractionates the tissue into its submicron components. This technology offers a method of noninvasive precise tissue atomization and is currently being explored for space usage [83].
Trauma Care in Space
Trauma stabilization and care on long-duration or long-distance mission presents unique difficulties in management. In these situations, the need for rapid evacuation can be a disastrous situation. Physiological alterations of microgravity, diminished immune systems, inexperienced or ill-equipped care providers, limited equipment, and extreme distance can conspire against a reasonable survival in traumatic injuries. The advantage to space exploration is that advanced procedures, training, and advanced technology can be incorporated into the design of these missions. These missions are analogous to rural trauma or trauma in undeveloped countries. Rural trauma in the United States shows that in distant populations, mortality can be up to 50 % greater than urban populations. Trauma in rural populations accounts for 60 % of deaths in the US, despite only 20 % of the population reside in these areas [84–86].
Crewmembers in orbit are hemodynamically challenged after 72 h in a microgravity environment. They have about a 15 % decrease in circulating red blood cell and plasma volume. This is defined as a class I hemorrhage terrestrially. Another factor in space physiology that is unique is the blunting of cardiovascular reflexes. These combined result in a decreased ability for a crewmember in microgravity to respond to blood loss. This can result in a shortened time in which intervention can have the greatest effect. They immediately move in to a class II type of hemorrhagic shock. The initial response to trauma must be rapid and consideration to fluid resuscitation must be given priority. As we have seen, ATLS procedures can be readily accomplished in the microgravity environment [40]. Intravenous access has been demonstrated experimentally and aboard the ISS. Securing an airway has also been demonstrated in parabolic flight using endotracheal intubation, laryngeal mask insertion, or surgical tracheostomy. A FAST (focused assessment with sonography for trauma) ultrasound can be utilized to evaluate for traumatic injury as well as confirm the endotracheal tube position [87, 88].
The truncal region requires surgical intervention to control internal bleeding. External pressure is not efficacious to control hemorrhage in this area. Ninety nine percent of deaths are due to thoracic or abdominal bleeding [89, 90]. Ultrasound has been used to localize intrapleural, intraperitoneal, and retroperitoneal bleeding terrestrially, in parabolic flight and onboard space vehicles. It is as sensitive as terrestrial-based applications [69–73, 91]. Management of these injuries has changed due to rapid diagnostic procedures. No longer is explorative surgery required and it has given way to observation and repeated scanning techniques. This also implies that surgical or intensive monitoring must be available in case there is recurrent hemorrhage. Observation may also be complicated by and require interventions in the cases of abscesses, pseudoaneurysms, urinomas, or biliomas. Many of these can be treated with percutaneous interventions and have been demonstrated in parabolic as well as actual spaceflights [80, 92]. These conditions still require surgical expertise if severe recurrent hemorrhage occurs. This would require specialized training and physician intervention [3, 93]. In the space environment, it may be better to intervene in a staged fashion rather than going directly to open procedures. In all of these cases, anesthetics would be required. Gaseous anesthetics have innumerable problems in a closed-loop environment. Re-inhalation and intoxication of the ones performing the interventions is a real risk. Also the incorporation of anesthetic decontamination equipment into the environmental control system may be space and cost prohibitive [3]. Intravenous anesthetic techniques are preferable and have been demonstrated in parabolic flights.
Immediate Damage Control Procedures
Severe shock and sepsis may demand an immediate surgical intervention before extensive diagnostics can localize the condition. A group of flight surgeons, trauma surgeons, and biomedical engineers emphasized that a laparotomy may be required to stabilize a patient prior to further procedures or deorbiting to Earth [94]. As discussed in Chapters 14 and 15, the paradigm of only completing the necessary components via limited procedures is referred to as damage control (DC) surgery. These methods do not require prolonged procedures that tax the patient’s physiological reserves. Also these procedures do not require extensive equipment outlays [8, 95]. These procedures are not significantly different from the terrestrial environment. Solid-organ bleeding can be tamponaded with packs around the offending organ. The abdominal wall can be left open for further procedures to follow. An open abdominal wall facilitates converting noncompressible bleeding into compressible visceral bleeding by direct methods. Fibrin glue and tissue sealants can also be used easily in these DC surgeries. These procedures have been demonstrated by physician extenders and non-surgeons [96]. These types of procedures would allow immediate DC surgery to be performed to stabilize the crewmembers condition. Then planning and further diagnostics can take place with consultation with ground control. Then long-distance training or reviews and simulations can be undertaken to perform a definitive surgical procedure.
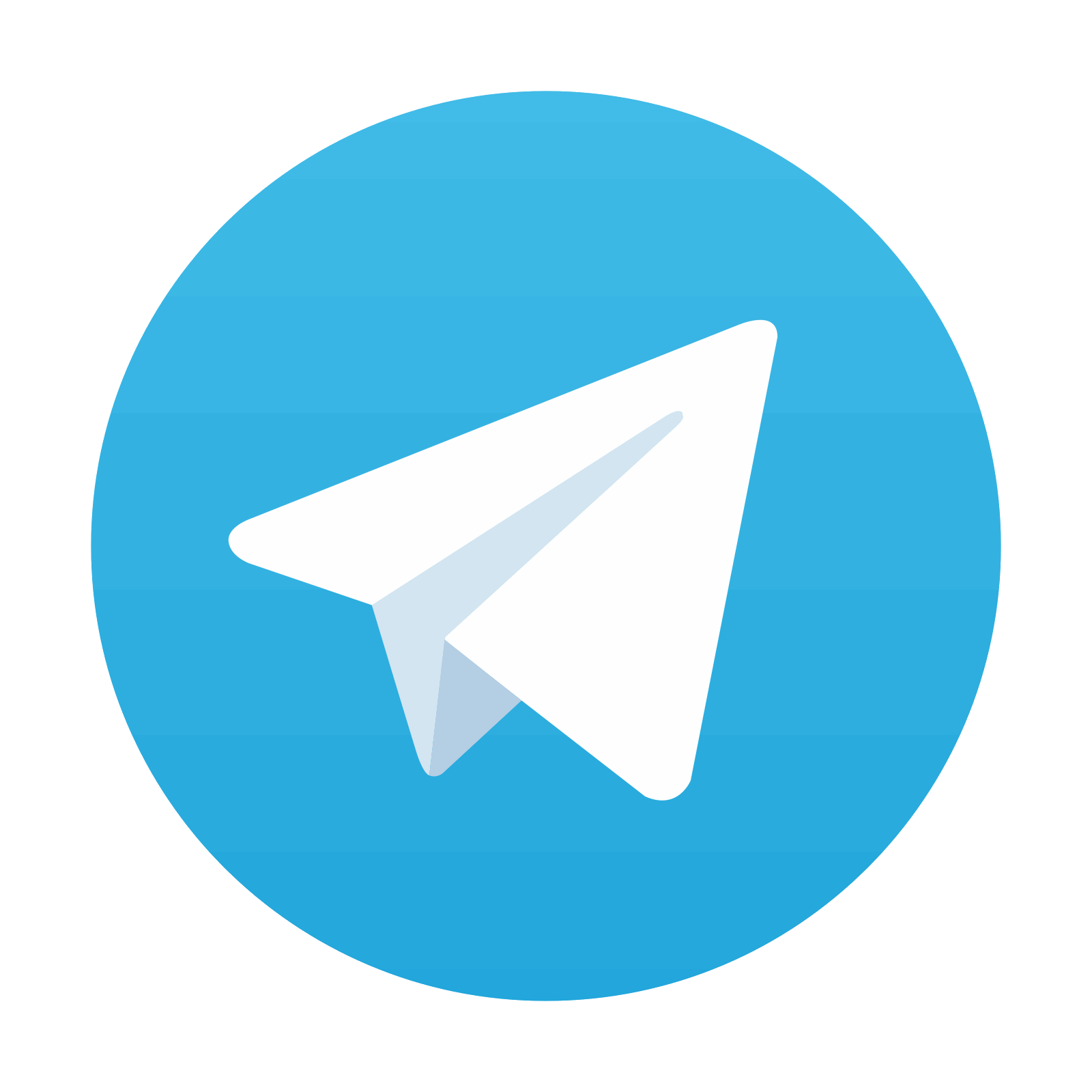
Stay updated, free articles. Join our Telegram channel

Full access? Get Clinical Tree
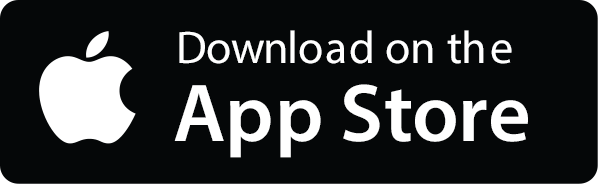
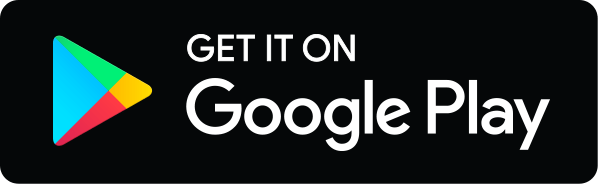