TREATMENT
Transfusion Decision-Making
Transfusion based on sound physiologic principles and an understanding of relative risks and benefits should give maximal benefit to the patient, with efficient use of a valuable and finite resource. Utilizing data from recent studies, it is increasingly possible to base transfusion practice on scientific grounds. The following transfusion guidelines are presented based on the best evidence currently available. Given the active ongoing investigations in this area, it is likely that frequent updates will be forthcoming.
Whole Blood
There have been few widely accepted indications for whole blood in modern transfusion practice. Storage of whole blood precludes the extraction of components and, from a systems perspective, is highly inefficient. As such, whole blood is not available from most blood banks in the United States. In theory, the goals of oxygen delivery and volume expansion can be achieved with PRBC and crystalloid solutions. Experience with the use of whole blood by the US military (9) has suggested that transfusion of warm fresh whole blood to combat-related trauma patients may have survival benefits versus similar patients receiving component therapy (10). The military experience with whole blood has not crossed into civilian medicine at this time (11), though it has launched discussion in related topics, such as appropriate PRBC/plasma/platelet ratio in massive hemorrhage resuscitation, limiting crystalloid infusion during hemorrhage resuscitation, and whether early plasma and platelet transfusion in hemorrhage conveys survival benefit.
Some pediatric cardiovascular surgeons use fresh whole blood for pediatric cardiovascular surgical patients. Cardiovascular surgery, especially cases involving cardiopulmonary bypass, instigates hemodilution and platelet dysfunction, which results in coagulopathy, as well as cytokine release and complement activation, which results in inflammation. Proponents of whole blood for pediatric cardiovascular surgery claim whole blood has improved hemostatic properties and induces less inflammation (12,13); however, this has been refuted by an RCT finding no clinical or biochemical advantage in fresh whole blood over component therapy in pediatrics undergoing cardiopulmonary bypass. In this study, fresh whole blood was actually associated with longer length of stay in ICU, fluid overload, and longer ventilator time (14).
Red Blood Cells
Packed red blood cells are the most commonly transfused blood product. The indications for transfusing PRBC are generally divided between two main categories of patients: those with and without acute hemorrhage. In both cases, PRBCs are transfused with the purported effects of increasing circulatory volume, transporting oxygen, and the rheologic effect of increasing blood flow/viscosity; in reality, these effects may not be demonstrated. Today, transfusions are not recommended for volume expansion, perhaps with the exception of massive hemorrhage, and may lead to transfusion-associated circulatory overload. Blood viscosity, while necessary to maintain microvascular circulation, may only benefit from rheologic support of transfusion in severe hemodilution, and a significant increase in blood viscosity may actually hinder perfusion (15). Despite this, anemia is a more common indication for PRBC transfusion than active hemorrhage (4,16–22). Practitioners commonly assume that anemia confers a risk for ischemia due to decreased oxygen delivery and, similarly, assume that PRBC transfusion can improve oxygen delivery and mitigate the risk of ischemia. In actuality, anemia may increase the risk of ischemia, and a PRBC transfusion may improve tissue oxygenation in some cases of severe anemia but, in many situations, the risk of transfusing PRBC appears to be greater than the probability of benefit.
The decision to transfuse a nonhemorrhaging patient can generally be made according to substantial evidence in the literature. As of this writing, approximately 9,000 patients have been enrolled in multiple RCTs comparing practices of giving PRBC transfusions at restrictive hemoglobin thresholds (i.e., 7 to 8 g/dL) versus liberal hemoglobin thresholds (i.e., 9 to 10 g/dL). Four RCTs evaluating the patient outcomes of 30- or 60-day mortality, organ failure, ability to walk 10 feet, myocardial infarction, and revascularization events in various patient groups (ICU, coronary artery bypass graft or cardiac valve surgery, hip fracture with cardiovascular disease, acute coronary syndrome) found no significant difference in outcomes in patients transfused restrictively or liberally (23–26). Two RCTs evaluating patient outcomes of 30- or 45-day mortality, myocardial infarction, and revascularization events in various patient groups (acute coronary syndrome, upper gastrointestinal bleed) favored restrictive transfusion practice over liberal (27,28); one of these studies associated increased mortality with liberal transfusion. Conversely, one RCT in cardiac surgical patients associated slightly higher mortality with restrictive transfusion practice at hemoglobin threshold of 7.5 g/dL (29). The summation of this high-quality evidence supports the use of a restrictive transfusion threshold, such as hemoglobin of 7 g/dL in ICU patients and gastrointestinal bleeds and hemoglobin of 8 g/dL in cardiac surgery or acute coronary syndrome patients.
It is important to understand that, despite intention of giving PRBC transfusion to anemic patients to enhance oxygen utilization by tissue, this does not always occur (15,30–33). Global oxygen delivery (DO2) is determined by the arterial content of oxygen as well as cardiac output (DO2 = CO × CaO2; where CO is the cardiac output and CaO2 is the arterial oxygen content). Arterial oxygen content is dependent on hemoglobin level and hemoglobin saturation. The ratio of oxygen delivery to global oxygen consumption (VO2), or DO2/VO2, is known as the oxygen extraction ratio and can be measured by mixed venous saturation (SvO2). The oxygen extraction ratio in a homeostatic patient is generally wide, around 20% to 30%, which allows for a broad margin of safety. With anemia or blood loss, as DO2 decreases and the oxygen extraction ratio narrows, a critical DO2 level is reached when DO2 can no longer keep up with VO2. A simple hypothesis postulates that a PRBC transfusion, by increasing both cardiac output and arterial oxygen content, would increase DO2 and therefore VO2. However, in actuality, VO2 seems to demonstrate independence of DO2 in circumstances where hypoxia is not severe. Studies show conflicting results on whether DO2 is actually increased after PRBC transfusion (4,34,35). While a PRBC transfusion almost always causes a posttransfusion rise in hemoglobin, which in itself is often associated with increased DO2, the VO2 is not always increased and ischemia (measured in terms of blood lactate level) is rarely improved (15,32,34,36,37); the reasons for this are not entirely understood. One reason may be that the increase in hemoglobin after a PRBC transfusion, by increasing blood viscosity, dampens the sympathetic response to anemia, decreasing cardiac output (4,38,39). Other reasons may include inability of oxygen to dissociate from hemoglobin based on depleted 2,3-diphosphoglycerate in transfused red blood cells, decreased functional density of the microcirculation, or the belief that many patients whom receive PRBC transfusions do not have severe enough ischemia whereby their VO2 is in the dependent phase with DO2 (4,15,40–42).
The decision to transfuse PRBC in nonhemorrhaging patients should be based on clinical assessment and not solely on hemoglobin levels. Common symptoms of anemia may include shortness of breath, fatigue, and tachycardia, but hypovolemia can also cause these symptoms, and the latter can be easily treated with crystalloids. Additional consideration should be given to factors such as patient age, comorbidity, and evidence or risk of ischemia. Consider the possibility of systemic hypoperfusion when there is persistently high lactate or central or mixed venous saturation below 60%. If the combined laboratory and clinical assessment of the nonhemorrhaging patient meet evidence-based guidelines for transfusion, one unit of PRBC at a time should be transfused. Hemoglobin equilibrates 15 minutes after transfusion is completed, and one PRBC unit can be expected to increase hemoglobin by 1 g/dL and hematocrit by 3%. After one unit, hemoglobin and clinical reassessment should be repeated to determine if further PRBC transfusion is necessary. The ultimate goal of the PRBC transfusion should not be to maintain a hemoglobin number, but to improve patient outcome. Guidelines for PRBC transfusion can be found in Table 144.2.
Platelets
Most platelet transfusions are given prophylactically to reduce the risk for spontaneous bleeding in thrombocytopenic patients. As described earlier, platelets can be prepared from whole blood, where one single platelet concentrate comes from one whole blood donation, or from apheresis, where up to three units can be collected at a time from a single donor. Platelet concentrates from whole blood can be transfused singly, often to pediatric patients, but are more commonly pooled together in pools of four or six concentrates, commonly referred to as a “six-pack.” Platelet units from apheresis donors are also known as “single-donor platelets.” One apheresis unit is considered equivalent to one pooled platelet containing four to six concentrates from multiple donors; the transfusion of the apheresis type of platelets is on the rise (1). The administration of an apheresis unit of platelets is advantageous since it exposes the recipient to a set of foreign antigens from a single donor, whereas an equivalent dose of pooled platelet transfusion exposes the patient to four to six sets of foreign antigens, increasing the risk of alloimmunization, or antibody-formation, to foreign antigens such as human platelet antigens (HPA) or human leukocyte antigens (HLA). In addition, bacterial contamination is less likely with single-donor apheresis platelets. In cases of alloimmunization to HPA or HLA, platelet refractoriness can occur, potentially necessitating special laboratory evaluation and procurement of products such as crossmatched platelets or HLA-selected platelets. These are costly and time consuming and may cause delay in transfusion.
TABLE 144.2 Guidelines for Transfusion of Packed Red Blood Cells |
![]() |
The efficacy of platelet transfusion may be assessed both by clinical parameters (improved hemostasis) and by following the platelet counts at 1 hour and 24 hours as an estimate of platelet survival. The platelet count at 1-hour posttransfusion of a unit of apheresis platelets (or apheresis-equivalent pooled platelets) should increase by 30,000 to 60,000 platelets/μL. Less pronounced responses should be expected with repeated transfusion and the development of alloimmunization, or in the presence of fever, sepsis, consumption, or splenomegaly. Failure of an appropriate rise in platelet count at 1-hour posttransfusion is suggestive of immune platelet refractoriness (i.e., alloimmunization), and failure to sustain increased platelet counts in 24-hour posttransfusion is suggestive of nonimmune platelet refractoriness (i.e., fever, sepsis, consumption, or splenomegaly) (43).
The evidence to support platelet transfusion decision-making ranges from low to moderate quality, as there are both observational studies and RCTs evaluating this concept (44). Three RCTs determined that prophylactic transfusions significantly reduced risk for spontaneous grade two or greater bleeding in inpatients with radiation and/or chemotherapy-associated hypoproliferative thrombocytopenia (45–48). Four RCTs determined the most appropriate platelet count threshold for prophylactic platelet transfusion to effectively reduce bleeding in therapy-associated hypoproliferative thrombocytopenia is 10,000 cells/μL (49–52). Transfusion of platelets at a 10,000 cells/μL threshold was associated with less platelet usage and fewer transfusion reactions, while transfusion at a 20,000 to 30,000 cells/μL threshold did not further decrease incidence of bleeding or bleeding-related mortality.
When the decision to transfuse platelets is made, dosing should commence with a single apheresis platelet or apheresis-equivalent single pool of platelets. Six RCTs have evaluated platelet dosing and determined that two apheresis platelet units does not decrease bleeding risk compared to one apheresis platelet unit (53,54), and that half of an apheresis platelet unit actually conveys the same prophylactic bleeding protection as one whole apheresis platelet unit (54–58).
Evidence supporting prophylactic platelet transfusion for invasive procedures is largely based on observational studies. A set of clinical practice guidelines on platelet transfusion from the AABB (formerly American Association of Blood Banks) suggests an appropriate platelet count for placement of central venous catheter is 20,000 cells/μL and for lumbar puncture or for major elective nonneuraxial surgery is 50,000 cells/μL (44). Guidelines for platelet transfusion can be found in Table 144.3.
Plasma
Plasma is used as a source of clotting factors in bleeding patients or patients requiring an invasive procedure with multiple coagulation factor deficiencies, such as those in liver dysfunction or consumptive or dilutional coagulopathy. An understanding of the half-life of clotting factors is necessary to help understand the appropriate use of plasma. Factor VII, the main clotting factor of the extrinsic pathway of the coagulation cascade, has the shortest half-life, in the range of 2 to 7 hours. Most factor VII is, therefore, depleted from plasma products before their manufacturing is complete, and thus plasma cannot correct a deficiency in factor VII, diagnosed by a prolonged prothrombin time (PT) and normal activated partial thromboplastin time (aPTT). The next factors with the shortest half-lives are factors V and VIII, with 15 to 36 and 8 to 12 hours, respectively. These factors also decline during plasma processing and storage, but not below the levels required for hemostasis. It is important to understand that normal hemostasis can be achieved with only 5% to 30% of normal clotting factor activity (59). The PT and the aPTT can be used to assess patients for need for plasma transfusion and to follow the efficacy of administered plasma. If both PT and aPTT are prolonged, consider a decrease in final common pathway clotting factors (prothrombin, fibrinogen, factor V, factor X) or a combined decrease in extrinsic and intrinsic factors, such as in vitamin K antagonists or liver disease. If aPTT alone is prolonged, consider a decrease in extrinsic clotting factors (factors VIII, IX, XI, and XII), such as with lupus anticoagulant.
TABLE 144.3 Guidelines for Transfusion of Platelets | |
![]() |
Clinical practice for the evaluation of bleeding or bleeding risk often deviates from the assessment of PT and aPTT as described above, focusing rather on the international normalized ratio (INR). The INR is a calculated value meant to standardize commercial reagents against international standards, in order for patients on vitamin K antagonists to achieve accurate and standardized results assessing their therapeutic range of treatment regardless of which laboratory performs the test. Some argue that the INR should only be used for this purpose but many in clinical practice use the INR to evaluate bleeding risk or target the INR for “correction” with therapeutic intervention in bleeding patients.
Two main points must be emphasized: firstly, that an INR prolonged in the mild to moderate range (<2) is not predictive of bleeding, and, secondly, plasma does not “correct” a mild to moderate elevation in INR. Twenty-five published studies between 1966 and 1996 have addressed these points, with the preponderance of data showing that the PT/INR does not show any correlation with clinical bleeding in association with invasive procedures unless they are very abnormal and that prophylactic plasma does not attenuate bleeding risk (59–73). The actual INR measured on plasma products varies between 1.14 and 1.4. Appropriate prophylactic utilization of plasma can thus be considered for patients requiring an invasive procedure with an INR in the moderately prolonged range, conservatively between 1.7 and 2.0.
Additional indications for plasma include dilutional coagulopathy related to massive hemorrhage, factor deficiencies where commercial factor concentrates are unavailable (factor V, factor XI), vitamin K antagonist reversal if vitamin K and/or four-factor prothrombinase complex concentrate are not available, thrombotic thrombocytopenic purpura if plasmapheresis is not available, and treatment or prophylaxis of thromboembolism in deficiency of antithrombin, protein C, or protein S.
TABLE 144.4 Guidelines for Transfusion of Plasma |
![]() |
Plasma should be avoided for normalizing an INR below 1.7 in the absence of bleeding, blood volume expansion or nutrition support, coagulopathy that can be corrected with vitamin K administration, and single-factor deficiency where commercial factor concentrates are available.
The dosing of plasma varies according to the underlying disease process necessitating the transfusion. When plasma is given for clotting factor replacement, a dose of 10 to 20 mL/kg total body weight is often used, which would increase clotting factors approximately 20% immediately posttransfusion. Guidelines for plasma transfusion can be found in Table 144.4.
Cryoprecipitate
Cryoprecipitate was originally developed as treatment for hemophilia A, due to the factor VII content. Each unit of cryoprecipitate contains a minimum of 80 IU of factor VIIIC and 150 mg fibrinogen in 5 to 20 mL plasma; it also contains von Willebrand factor and factor XII. With technologic advances in recombinant factor development, cryoprecipitate is no longer used for its original purpose, but is instead used for acquired coagulopathy, such as in clinical settings associated with hemorrhage, or in consumptive disorders of fibrinogen. Solid clinical evidence for appropriate utilization and fibrinogen threshold for cryoprecipitate transfusion is lacking. Empirically, general guidelines for the transfusion of cryoprecipitate include bleeding patients with fibrinogen below 100 mg/dL or von Willebrand disease unresponsive to desmopressin when factor concentrates are unavailable. Cryoprecipitate has fallen out of favor, particularly in some European countries where it has been removed from the market, in lieu of fibrinogen concentrate, which delivers fibrinogen in a smaller volume and with a safer profile than a human donor blood product (74).
In adults, cryoprecipitate is often given in a pool of 5 to 10 individual units, resulting in a volume between 50 and 200 mL, dependent on individual blood banks; each unit in the pool will increase the fibrinogen by 5 to 10 mg/dL in an average-sized adult. In children, cryoprecipitate can be given in individual units at a dose of 1 to 2 units/10 kg, which can increase fibrinogen by up to 100 mg/dL. Guidelines for cryoprecipitate transfusion can be found in Table 144.5.
TABLE 144.5 Guidelines for Transfusion of Cryoprecipitate |
![]() |
Blood Component Modification
Once the decision to transfuse a patient with a blood product is made, attention must be turned to whether the patient requires modification to the blood product, such as leukoreduction, irradiation, or washing. Leukoreduction is a process that depletes the white blood cells in either a PRBC or platelet blood product, and this process can be completed prestorage or poststorage (i.e., pretransfusion). Prestorage leukoreduction is often preferred because earlier removal of white blood cells from the product decreases the possibility of cytokine leak from white blood cells into the product. In blood products collected by apheresis, leukoreduction is inherently performed by the mechanics of apheresis collection. Leukoreduced blood products are considered cytomegalovirus-safe (“CMV-safe”); the sites of CMV latency are thought to include progenitor cells that express CD34 and monocytes that express CD13 and CD14 antigens, thus leukoreduction of blood products decrease the transmission of CMV. One school of thought reasons that leukoreduced products are superior to CMV-seronegative products because, in early CMV infection, when the virus is solely intracellular, antibody tests used to connote a donor as seronegative would result as negative despite viral infection. In actuality, neither CMV-seronegative nor CMV-safe products equal “zero risk” to patients, as CMV transmission can occur at extremely low rates from both types of products (75). To combat acquisition of CMV from transfusion, patients in at-risk disease states should be transfused with leukoreduced blood products and then be monitored for CMV infection and/or treated with CMV prophylactic medication. CMV-seronegative blood products, while also effective at decreasing CMV transmission, are more difficult to acquire and cause delays in transfusion and potential costs to the health system. Overall, leukoreduction is indicated for decreased transmission of CMV, decreased incidence of febrile non-hemolytic transfusion reaction (HTR), and decreased likelihood of developing HLA alloimmunization in transplant patients or patients that are transfusion-dependent.
Irradiation is a process whereby gamma or x-ray irradiation causes DNA cross-linking in T-lymphocytes, rendering them inactive and unable to mount an immune response against the recipient. When T-lymphocytes do mount immune responses against immunocompromised transfusion recipients, the result is transfusion-associated graft versus host disease (TA-GVHD), which is nearly uniformly fatal. Indications for irradiated blood products include bone marrow or hematopoietic stem cell transplant candidate/recipient, congenital cellular immune deficiency, pediatric oncology on active chemotherapy, intrauterine transfusion, transfusion from blood relative, neonates less than 4 months of age, or patients receiving fludarabine, other purine analogues, or alemtuzumab chemotherapy. Caution should be exercised in pediatric patients receiving large-volume or rapid infusion of irradiated PRBC if the irradiation was performed more than 24 hours before the transfusion, as irradiation can damage red blood cells, leading to potassium leak into product supernatant and placing the patient at risk for hyperkalemia.
Washing is a process that removes most plasma/supernatant from PRBC or platelet products. The process of washing can remove up to 99% of the plasma/supernatant but also results in cellular loss of up to 33% of the red blood cells or platelets; it also causes increased red blood cell fragility, making them more susceptible to hemolysis, and can adversely affect platelet function. Washing decreases the shelf-life of the product, and, if performed in an open system, can increase the chance of bacterial contamination. The process is labor-intensive and can create over a 1-hour delay for transfusion. The indications for washed PRBC and platelets are in patients with IgA deficiency and anti-IgA antibody if no IgA-deficient products are available or in patients with history of severe anaphylactoid reactions to blood products.
Risks of Blood Transfusion
Even though a blood transfusion is a potentially life-saving intervention, significant risks are still involved in the administration of these products. Direct risks causally related to the transfusion range from mild, in the case of urticaria, to fatal and include transfusion-transmitted infection, transfusion reactions, volume overload, and potential for alloimmunization and mistransfusion. There are also plausible risks for increased morbidities such as increased infection, prolonged ventilator use, increased length of stay, and organ dysfunction, as well as increased mortality. A summary of blood transfusion risks can be found in Table 144.6.
The U.S. Food and Drug Administration (FDA) produces an annual report on fatalities related to blood transfusion. During the FDA fiscal year 2013 (October 1, 2012, to September 30, 2014), 65 transfusion recipient fatalities were reported to the FDA (76). Of the 65 deaths, 38 were directly related to the transfusion, and, in 21 deaths, the transfusion could not be ruled out as the cause of death. The majority of deaths were due to transfusion-related acute lung injury (TRALI) (14 deaths), followed by transfusion-associated circulatory overload (TACO) (13 deaths), non-ABO HTR (5 deaths), microbial infection (5 deaths), and ABO hemolytic-transfusion reaction (1 death). The leading causes of transfusion-related death over the past 5 years have remained primarily TRALI, followed by TACO. Physicians are bound by oath and duty to fully understand the risks of transfusion, along with the benefits, alternatives, and consequences of not transfusing, in order to engage patients in discussions regarding transfusion decision-making and to obtain informed consent.
TABLE 144.6 Risks of Blood Transfusion |
![]() |
Transfusion-Transmitted Infection
Infectious pathogens within blood products have been well-documented as posing a threat to public safety. Nearly all blood products are administered to recipients without sterilization or pathogen inactivation, and thus infectious pathogens that are not detected in the donor at the time of donation can be passed to the recipient. Pathogens range from bacterial to viral to parasitic to prion, some of which are highly tested for in donors but others of which rely on accurate answers and comprehension of donor screening questions in order to defer donors from donating potentially infectious blood.
Currently, tests are required on all blood donations for the detection of hepatitis B virus (HBV), hepatitis C virus (HCV), human immunodeficiency virus, types 1 and 2 (HIV-1/2), human T-cell lymphotropic virus, types I and II (HTLV-I/II), syphilis, west nile virus (WNV), and Trypanosoma cruzi. All of these pathogens have been documented to cause transfusion-transmitted infection. Technologic advances in many of the viral tests have become so advanced that, via detection of viral nucleic acid, transmission of HBV, HCV, and HIV through transfusion is nearly nonexistent. The rate of transmission of these is so low that risk is calculated only by theoretical modeling. There are still windows of time, however, immediately after infection occurs when even the highest quality tests cannot detect the virus. These periods of time, known as the “infectious window period,” is 7.4 days for HCV, 9.1 days for HIV, and 26.5 to 18.5 days for HBV (77). Extensive questioning of donors on risk-associated behavior is also used to defer donors who have engaged in activities that may confer infection from these viruses.
Other than syphilis, there are no specific tests on donors for bacteria. Bacterial contamination occurs in approximately 1 in 3,000 blood products, and can cause anything from asymptomatic bacteremia in recipients to death. Babesia microti and Staphylococcus aureus have caused the most transfusion-transmitted infection fatalities over the past 5 years (76). Platelets do undergo blood culture prior to transfusion, although occasional culture-negative platelets have still caused transfusion–transmitted infection. The requirement for a secondary test for bacterial detection in platelet products is under current consideration by the FDA.
Transfusion–transmission of malarial protozoa and the prion responsible for variant-Creutzfeldt–Jakob disease have been documented. There are no FDA-approved tests for these pathogens and therefore donor screening questions help protect recipients from being at risk for these infections.
Transfusion Reactions
As discussed earlier, the noninfectious complications of transfusion are more likely to cause fatality than infectious ones, and the three leading causes of transfusion-associated mortality are TRALI, TACO, and HTR. Categories of adverse transfusion reactions and their management according to AABB are shown in Table 144.7. Transfusion reactions can be categorized broadly by timing and etiology into acute (<24 hours) or delayed (>24 hours) and immunologic or nonimmunologic. The responsibility for understanding transfusion reactions lies both with the physician ordering the transfusion, as he or she must discuss the risks with the patient, and with the transfusionist administering the blood product, as he or she must be vigilant for signs and symptoms of a reaction. Many common signs and symptoms of transfusion reactions are shared among the various types of reactions and generally include:
- Fever (defined as temperature ≥38°C and an increase of at least 1°C from pretransfusion value)
- Chills and/or rigor
- Skin manifestations such as urticaria, rash, flushing
- Hypo- or hypertension
- Respiratory distress such as tachypnea, dyspnea, wheezing, or coughing
- Pain at site of infusion or in abdomen, back, chest, or flank
- Jaundice (hyperbilirubinemia)
- Nausea/vomiting
TABLE 144.7 Transfusion Reactions: AABB Classification | ||
![]() |
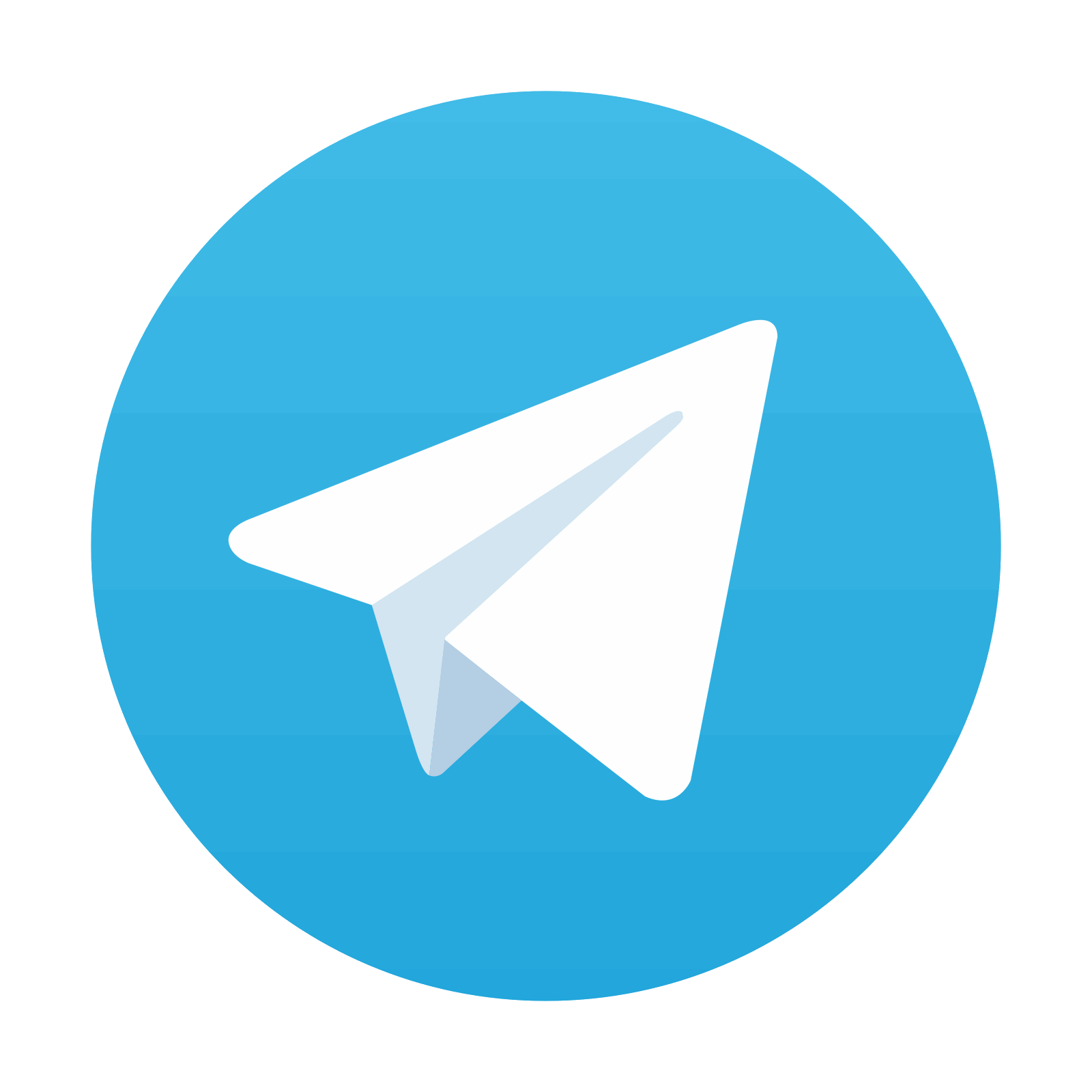
Stay updated, free articles. Join our Telegram channel

Full access? Get Clinical Tree
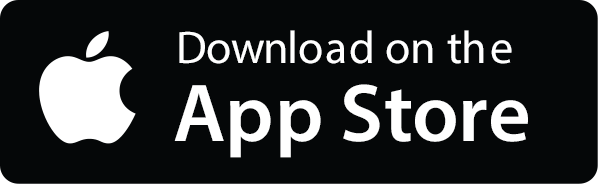
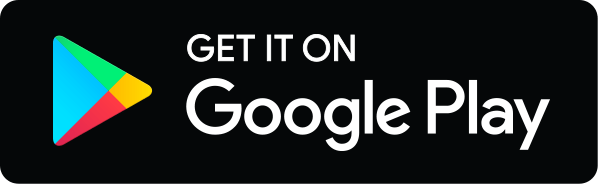