Transfusion Therapy: Blood Components and Transfusion Complications
Terry Gernsheimer
Transfusion support can be a key element in decreasing morbidity and mortality of the critically ill patient by the support of oxygen delivery and correction of hemostatic abnormalities. An understanding of the benefits, limitations, and risks of blood component therapy is of fundamental importance in the intensive care setting. This chapter will outline blood components available for transfusion, their appropriate dosages, and therapeutic effects. Complications of transfusion therapy, including infectious risks, transfusion reactions, effects of storage, and immunomodulatory effects, as well as methods to minimize these complications, will be discussed.
Blood Component Therapy
Cellular Blood Components
Red Blood Cells
One unit of “packed” red blood cells (pRBC) is processed by the removal of platelet rich plasma from a donated unit of whole blood and contains approximately 200 mL red blood cells, usually less than 50 mL plasma, and an additive that brings the component to 300 to 350 mL in total volume. Depending upon the additive, the storage life at 4°C will be from 35 to 42 days. Red blood cell storage has multiple theoretic and measurable effects. Any platelets still present in the component are rendered inactive by the cold storage. As red blood cells are stored, intracellular potassium leaks into the plasma space. 2,3-Diphosphoglyceric acid (2,3-DPG) may also be depleted from the red blood cells, which theoretically could cause increased oxygen affinity and decreased release of oxygen at the tissues [1]. This effect reverses after several hours in vivo but may be clinically significant in the patient undergoing massive transfusion. Stored pRBC also have elevated plasma ammonia levels, elevated PCO2, lowered pH, and increased amounts of microaggregates. These all have theoretic effects on oxygen delivery when given rapidly in large amounts. Massive transfusion can also theoretically result in hypocalcemia and hyperkalemia.
In 1993, Marik and Sibald [2] reported the incidental finding of increased gastric pH in 23 patients with septic shock transfused with 3 units of pRBC, but Walsh failed to find a similar effect in a small randomized control trial in 22 patients with septic shock transfused with pRBC stored for less than 5 or more than 20 days [3]. Hébert found a higher incidence of mortality and life-threatening complications who received blood stored less than 8 days when compared with standard therapy in a randomized study of 66 patients undergoing cardiac surgery [4]. Although van der Watering did find longer ICU stays and decreased survival in a retrospective study of 2,732 patients undergoing coronary artery bypass graft (CABG) who received blood that had undergone a median age of ≥ 18 days or more versus less than 18 days of storage, this difference was not apparent in a multivariate analysis [5]. A retrospective report of a large number of patients (5,902) by Koch et al. [6] showed a significant increase in mortality and complications at 1 year in patients undergoing CABG who received blood > 14 days of age versus < 15 days of age, but differences in characteristics of the two patient groups complicated the analysis. The effect of storage age remains controversial [7] and will require careful
prospective randomized clinical trials in adequate numbers of patients before the true clinical significance of storage age and the nature of the effect becomes clear [8].
prospective randomized clinical trials in adequate numbers of patients before the true clinical significance of storage age and the nature of the effect becomes clear [8].
Other than factors V and VIII, the activity of most coagulation factors are quite stable during storage, even after 2 weeks, and therefore whole blood (without the plasma removed), when available, may be used in selected patients with coagulopathy and bleeding, and can reduce donor exposure by limiting administration of multiple products (e.g., red cells and plasma) [9]. Factor V levels in stored whole blood are well above 50% and therefore adequate for hemostasis. Factor VIII is produced by endothelial cells as well as by the liver, and levels increase in the setting of inflammation, so a decrease with storage may be less clinically relevant. Whole blood may also be the preferred form of red cell transfusion in patients who require intravascular volume expansion as well as increased oxygen carrying capacity.
The primary function of hemoglobin in RBCs is to transport oxygen efficiently from the lungs to the various tissues of the body. Oxygen transport is a complex process regulated by several different mechanisms of control, involving the heart and vascular system. The most important functional feature of the hemoglobin molecule is its ability to combine loosely and reversibly with oxygen. Decreased hemoglobin oxygen affinity and increased tissue oxygen delivery occur with increased temperature and decreased pH, when there are increased tissue requirements. Oxygen is also less tightly bound with increased 2,3-DPG levels, which increases in the chronically ill patient [10]. In the seriously ill patient with severe acidosis and septic shock, however, 2,3-DPG levels may decrease resulting in decreased tissue oxygen delivery.
In a normovolemic, otherwise healthy individual, the effect of a decreased hematocrit is decreased blood viscosity and a compensatory augmentation of cardiac output and blood flow to most organs [11]. Human and animal studies reveal remarkable tolerance for hematocrit levels as low as 15% [12,13], but an optimum value has not been well defined and is very dependent on the patient’s physiologic state. A decrease in the hematocrit also involves a redistribution of blood flow away from the endocardium and may have adverse effects on ischemic cardiac tissue. A retrospective analysis of patients older than 65 years hospitalized with acute myocardial infarction found that in patients with a hematocrit less than 30.0% (and perhaps < 33.0%) on admission, transfusion was associated with a lower 30-day mortality rate [14]. However, in patients who had undergone elective CABG, postoperative transfusion for hemoglobin levels greater than 8 did not improve morbidity, mortality, or complication rates [15]. Postoperative patients with known vascular disease and hematocrits less than 28% have been shown to have a significant increase in myocardial ischemia and morbid cardiac events [16], and in one study that retrospectively evaluated patients refusing transfusion on religious grounds, low preoperative hemoglobin was associated with increased morbidity and mortality in patients with cardiovascular disease undergoing surgery [17]. In a large multicenter, randomized trial, there was no difference in adverse outcomes when patients with cardiac disease were transfused at a hemoglobin threshold of 7.0 g versus 10 g [18]. In this study of more than 800 patients, less acutely ill, younger patients (< 55 years of age) without cardiac disease who were randomized to the more liberal (higher) transfusion trigger had an overall higher mortality rate. A restrictive RBC transfusion strategy also did not adversely affect outcomes related to mechanical ventilation [19]. In postoperative patients without cardiovascular disease, few data support interference with wound healing or increased anesthesia risk at hemoglobin levels of less than 10 g per dL [20], and hemoglobin values as low as 7 g per dL appear to be safe in otherwise healthy individuals [21].
Advocates of restrictive transfusion strategies point out that transfusing to normal hemoglobin concentrations does not improve organ failure and mortality in the critically ill patient [22] and to data that transfusion may actually be associated with increased infection rates, morbidity and mortality [23]. Proponents of more liberal transfusion strategies point out the possible detrimental effects that may be associated with oxygen debt [24]. A thoughtful transfusion policy is dependent on the time the anemia developed over and can be expected to continue; additional medical problems that may make a patient more susceptible to anemia, such as tissue ischemia and pulmonary disease; and whether there is rapid, ongoing blood loss.
Blunted erythropoietin responses have been noted in critically ill pediatric [25] and adult patients [26]. Long-term intensive care patients may not only fail to increase their erythropoietin level in response to anemia but may have correctable nutritional deficiencies and iron profiles consistent with anemia of chronic disease. Although erythropoietin therapy increases red blood cell production and appears to decrease transfusion needs [27,28,29], the effect can take weeks and may reduce blood cell transfusion only minimally. It is an expensive alternative to more restrictive transfusion strategies to reduce transfusion exposure in appropriately chosen patients.
Studies in animal models [30] and in humans [31,32] reveal that platelet function and interaction with subendothelium decline at lower hematocrits. In the thrombocytopenic and thrombocytopathic (e.g., uremic) patient, transfusion to higher hematocrit values is appropriate in the patient at risk of bleeding.
Therapeutic Effect
The response to red cell transfusion will depend on intravascular volume, but it can be estimated that one unit of pRBC will increase the hematocrit by approximately 3%. It may take up to 24 hours while intravascular volume equilibrates for full effect. Rapid ongoing red cell destruction or splenic sequestration may also affect the hematocrit increment as well as the red cell survival.
Emergency Blood Usage
Uncrossmatched type O RBCs can be used for a bleeding patient in dire emergency. Type O, Rh-negative RBCs can be transfused to people of any blood type with only a slight risk of hemolysis. This risk increases in patients who have previously been transfused or pregnant and may have formed antibodies [33]. Type O, Rh-positive RBCs are sometimes used for women who are beyond childbearing age and in adult males. When Rh-positive RBCs are used in an Rh-negative patient, there is a chance of a D immunization, and if the patient requires emergency transfusion in the future, they may have preformed antibodies. Anti-D antibodies do not generally cause immediately intravascular hemolysis but rather a slow extravascular hemolysis, so the risk is small overall. Anti–Rh-D (Rhogam®) may be given within 48 hours of giving transfusion of Rh-positive blood to an Rh-negative woman of childbearing age, but the amounts required limit its use in prevention of immunization.
Platelets
Platelets are essential for the initial phase of hemostasis. Following exposure of subendothelial substances, platelets adhere to the subendothelial tissues by von Willebrand factor and other adhesive proteins. This initial adhesion activates platelets, causing release of platelet alpha and dense granules. Some of these granule contents, including factor V, fibrinogen, von Willebrand factor, and calcium, move to the extracellular space via the open canalicular system, increasing their concentrations in the immediate “neighborhood” of the platelet. With platelet activation, anionic phospholipids move to the platelet surface, forming binding sites collectively known as platelet factor 3, upon which coagulation factors can interact with
calcium to form IXa, Xa, and thrombin. Platelet glycoprotein IIb-IIIa is exposed and binds fibrinogen. Thrombin generation causes further platelet activation and converts fibrinogen to fibrin, resulting in a platelet-fibrin mass that can effectively cease bleeding from a break in the endothelium. Fifteen percent of the platelet’s protein is actin and myosin, which, upon coupling in the presence of increased concentrations of adenosine diphosphate (ADP) and calcium, leads to cytoskeletal movement and clot retraction.
calcium to form IXa, Xa, and thrombin. Platelet glycoprotein IIb-IIIa is exposed and binds fibrinogen. Thrombin generation causes further platelet activation and converts fibrinogen to fibrin, resulting in a platelet-fibrin mass that can effectively cease bleeding from a break in the endothelium. Fifteen percent of the platelet’s protein is actin and myosin, which, upon coupling in the presence of increased concentrations of adenosine diphosphate (ADP) and calcium, leads to cytoskeletal movement and clot retraction.
The threshold of thrombocytopenia at which bleeding may occur will vary depending on the patient’s clinical condition. In general, spontaneous bleeding does not occur until the platelet count falls below 5,000 to 10,000/μL [34,35,36,37]. The recommended “trigger” for prophylactic platelet transfusions in patients undergoing chemotherapy or hematopoietic stem cell transplantation (HSCT) without bleeding or other comorbid conditions is less than 10,000/μL. For the majority of invasive procedures, a platelet count of 30 to 50,000/μL will be adequate. For high-risk procedures, such as neurologic or ophthalmologic surgeries, a platelet count of 100,000/μL is recommended by the American Society of Anesthesiology [38] and the College of American Pathologists [39]. Technique and experience appear to be as least as important predictors of bleeding following placement of catheters as clotting abnormalities, even in patients with isolated platelet counts less than 20,000/μL [40]. The risk of bleeding with thrombocytopenia increases when complicated by other hemostatic abnormalities.
Platelet counts less than 50,000/μL are associated with increased risk of microvascular bleeding in the massively transfused patient [41]. For this reason, platelet transfusion has been advocated with replacement of every blood volume to avoid the effect of dilutional thrombocytopenia [42]; however, some investigators have found that patients receiving prophylactic platelet transfusion were no less likely to develop microvascular bleeding [43]. In patients with brisk ongoing blood loss, rapid turnaround of platelet counts can direct diagnosis and are important in managing transfusion therapy.
Higher transfusion triggers may be indicated with abnormal platelet function [44]. Platelet function abnormalities may be congenital or acquired. Medications, sepsis, malignancy, tissue trauma, obstetrical complications, and extra corporeal circulation may all adversely affect platelet function. Liver and kidney disease may be associated with severe thrombocytopathy. Hypothermia prolongs bleeding time in trauma patients [45] and arterial hemorrhage in animals [46]. Glycoprotein IIb-IIIa inhibitors may affect platelet number as well as function. If platelet dysfunction is present, the patient with a disrupted vascular system (e.g., trauma or surgery) will require a higher platelet count to achieve hemostasis. Higher counts may be necessary to prevent spontaneous bleeding as well. The transfused platelets may quickly become dysfunctional in the patient, and other therapy may be necessary, such as dialysis and dialysis and desmopressin acetate (DDAVP) for bleeding in renal failure, rewarming of the hypothermic patient, or correction of acidosis.
In several situations, platelet transfusions may not be indicated unless there is significant bleeding. In autoimmune thrombocytopenias (e.g., immune thrombocytopenia (ITP) and posttransfusion purpura), transfusion increments are usually poor and platelet survival is short. Administration of intravenous immune globulin in high doses may improve transfusion response and survival as well as treat the underlying disease [47]. There have been reports of rapid exacerbation of the thrombotic process in the cerebrovascular circulation in patients with thrombotic thrombocytopenic purpura (TTP) following platelet transfusion [48]. These reports are anecdotal and may represent disease progression, but in general, platelet transfusions are felt to be relatively contraindicated in TTP unless there is clinically significant bleeding.
Table 114.1 Expected Platelet Increment with Transfusiona | ||||||||||||||||||||||||||||
---|---|---|---|---|---|---|---|---|---|---|---|---|---|---|---|---|---|---|---|---|---|---|---|---|---|---|---|---|
|
Pooled random donor platelet concentrates are prepared from platelets that have been harvested by centrifuging units of donated whole blood. Up to 8 units of platelets, each from a separate donor, can be pooled into a single bag for transfusion. All units are from the same ABO type. If ABO compatible platelets are unavailable, in most cases, pooled ABO incompatible platelets can be substituted with very little risk. The usual adult dose is 1 unit per 15 kg of body weight. Four to six units of pooled random donor platelets are frequently used in patients receiving prophylactic transfusions; however, a study of more than 1,200 hospitalized patients with thrombocytopenia due to chemotherapy or HSCT for hematologic malignancy showed no difference in bleeding incidence and decreased platelet exposure overall when transfused with low (1.1 × 1011/m2), medium (2.2 × 1011/m2), or high (4.4 × 1011/m2) doses of platelets prophylactically for platelet counts of less than 10,000/μL [49], suggesting that a dose of only 3 or 4 units of pooled random donor platelets is adequate. Patients who received smaller doses did require more frequent transfusions, making this strategy less appropriate for outpatient transfusion.
In a 70-kg patient with a normal sized spleen, each unit is expected to increase the platelet count by approximately 7,000/μL (Table 114.1) when checked 10 minutes to 1 hour after transfusion [50]. The survival of transfused platelets averages 3 to 5 days but will decrease if a consumptive process is present. Platelet concentrates also contain about 60 mL of plasma per unit and small numbers of red blood cells and leukocytes. Platelet units must be maintained at room temperature, as platelets lose shape and release their granular contents when refrigerated. Apheresis platelets, collected from a single donor, are prepared in components equivalent to 4 to 6 pooled units. An apheresis platelet concentrate contains 200 to 400 mL of plasma and, if the plasma is of an incompatible type, may be reduced in volume by centrifugation, although this results in an approximate 10% to 15% loss of platelets and probably some loss of function. Apheresis platelets may be collected for a specific recipient from a family member or other human leukocyte antigen (HLA) compatible donor for patients that have become refractory to random donor platelet transfusions due to alloimmunization. Leukocyte reduction of transfused cellular blood components has been clearly shown to reduce the rate of alloimmunization in patients undergoing chemotherapy for acute myelocytic leukemia [51].
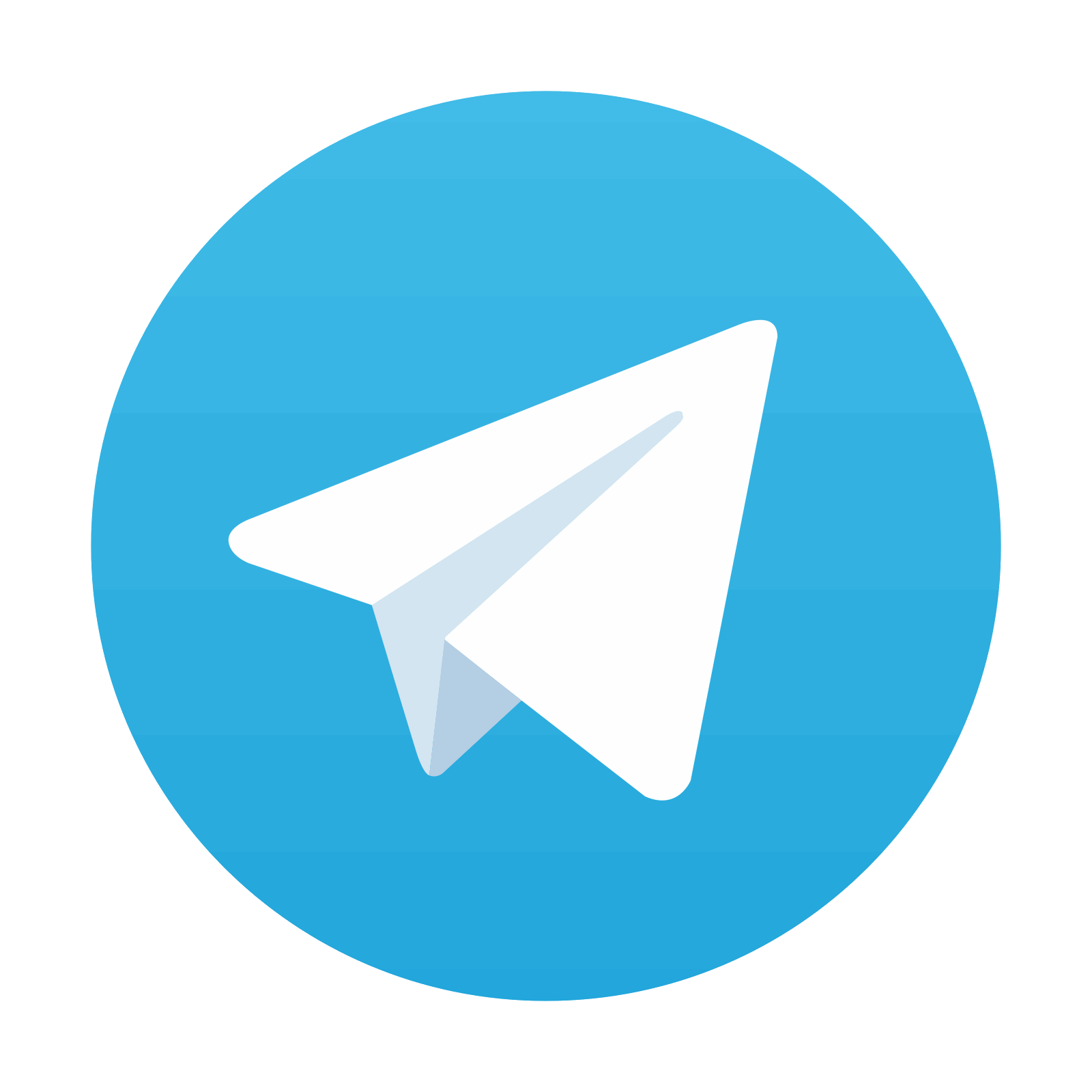
Stay updated, free articles. Join our Telegram channel

Full access? Get Clinical Tree
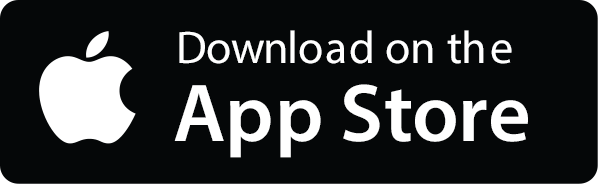
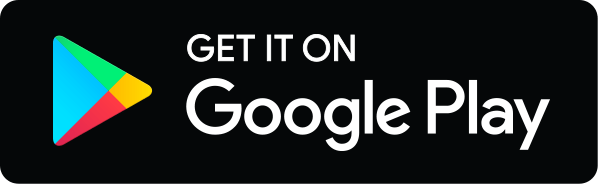
