The incidence of congenital heart disease (CHD) is 0.5% to 1%, and common malformations are less frequent (0.15%).1 An increasing percentage of these infants survive to adulthood largely due to advances in cardiology, cardiac surgery, and perioperative anesthetic and critical care management.2 At present, adults with congenital heart disease constitute a significant and growing cardiac population of 5%.
In patients with CHD, transesophageal echocardiography (TEE) allows for the real-time acquisition of both anatomic and hemodynamic information, thereby helping in clinical decision making. During interventional cardiac catheterization procedures, TEE is instrumental in the monitoring and guidance of valvuloplasties, angioplasties, closure of intracardiac shunts, trans-septal atrial puncture, and electrophysiological ablation. During palliative and corrective surgical procedures, TEE is fundamental in confirming diagnosis; detection of unanticipated findings; modification of surgical procedures; assessment of the adequacy of the procedure; guidance of revision; monitoring of intracardiac air, ventricular volume, and myocardial performance; and formulation of anesthetic and postoperative management. The primary objectives of TEE in patients with CHD are to define important anatomic and hemodynamic information when data provided by other modalities are inadequate, establish a complete evaluation of complex congenital heart disease, and confirm or exclude a diagnosis of clinical relevance.
Congenital heart disease has been classified based on the level of complexity, presence or absence of cyanosis, and primary physiologic alterations. TEE image interpretation is therefore best performed using a segmental approach,3 where the heart is considered in terms of three segments (atria, ventricles, and arterial trunks), and these are connected via two junctions (atrioventricular and ventriculoarterial).4 The use of a segmental approach provides a systematic guide for verification that all significant chambers and valves and their relationships have been recorded. Important determinants in this segmental analysis include:
- Visceral situs
- Venoatrial connections (systemic and pulmonary veins)
- Atrial situs (normal, inversus, right isomerism, or left isomerism)
- Ventricular morphology (right versus left)
- Atrioventricular septae
- Atrioventricular valves
- Semilunar valves
- Ventricular outflows
- Great arteries
- Atrioventricular connections (concordant, discordant, double inlet, straddling, absent)
- Ventriculoarterial connections (concordant, discordant, double outlet, single outlet)
The usual orientation of the organs with the liver on the right and the spleen on the left is referred to as situs solitus. Situs inversus refers to a situation where given organs, or even all bodily organs, are reversed (eg, liver on the left and spleen on the right). Asplenia is associated with bilateral right-sidedness (right isomerism—liver on both sides), while polysplenia is associated with bilateral left-sidedness (left isomerism—spleen on both sides).
A persistent left superior vena cava (PLSVC) is the most common thoracic venous anomaly and occurs in 0.4% of the general population and in 4% to 11% of patients with congenital heart disease.5 The etiology of this defect is thought to be the failure of regression of left anterior and common cardinal veins and left sinus horn. In 90% of cases, the PLSVC connects to the right atrium through the coronary sinus (Figure 18–1). In the remainder, the PLSVC connects to the left atrium. A PLSVC is associated with atrioventricular canal defects, tetralogy of Fallot, and anomalies of the inferior vena cava. The clinical presentation and physiologic consequence of a PSLVC depend on its association with other anomalies. If the PSLVC is isolated, patients may remain asymptomatic.
The diagnosis of this defect in patients undergoing cardiac surgery raises several issues. First, the passage of a pulmonary artery catheter into the right ventricle via puncture of the left internal jugular vein may be difficult because the catheter may traverse the coronary sinus. Similarly, a PSLVC can complicate placement of permanent pacemakers and automatic implantable cardioverter defibrillators. Second, placement of a separate cannula in the coronary sinus may be necessary for complete venous drainage into the cardiopulmonary bypass machine. Third, retrograde cardioplegia in these patients will be ineffectively delivered to the myocardium. Finally, if patients with PLSVC undergo a heart transplant, the coronary sinus would have to be carefully dissected so that the PLSVC can be re-anastomosed to the right atrium
The bicaval view, midesophageal (ME) four-chamber view, and ME two-chamber view are the most useful in assessing this lesion. On two-dimensional examination, an enlarged coronary sinus (normal coronary sinus size is 1 cm) is most often the first clue to the presence of a PLSVC (Figure 18–2). The diagnosis can be confirmed by injecting agitated saline solution into a vein in the left arm. In patients with a PLSVC, the “contrast” will be seen first in the coronary sinus before arriving into the right atrium (see Figure 18–2C).
Figure 18-2.



A: In the four-chamber view, a large coronary sinus is seen to the right of the image and often is the first clue to the presence of a persistent left superior vena cava. B: Advancing the probe from the four-chamber view shows a dramatically enlarged coronary sinus. C: Injection of agitated saline solution into the left arm demonstrating near opacification of the coronary sinus (arrow) before bubble entry into the right atrium confirms the presence of the persistent left superior vena cava. (CS, coronary sinus; LA, left atrium; LV, left ventricle; RA, right atrium; RV, right ventricle.)
Anomalous drainage of the pulmonary veins results from in utero failure of the pulmonary veins to fuse with the left atrium. Two types have been identified. In patients with total anomalous pulmonary venous drainage, all pulmonary venous return is directed into a systemic venous system, creating a large left-to-right shunt. The site of pulmonary venous drainage may be supracardiac (into the innominate vein or left- or right-sided superior vena cava), cardiac (into an enlarged coronary sinus), or infracardiac (into the portal vein, ductus venosus, hepatic vein, or inferior vena cava) (Figure 18–3). Some degree of interatrial mixing (usually atrial septal defect [ASD] or patent foramen ovale [PFO]) is mandatory and provides the only access for pulmonary venous blood to the left heart. Survival beyond infancy without surgical intervention is unlikely; hence, this entity is not encountered in the adult population.
Partial anomalous pulmonary venous drainage is characterized by failure of one or two of the pulmonary veins to connect with the left atrium. Most commonly, the right upper and/or right lower pulmonary veins drain into the superior vena cava or the junction of the right atrium and superior vena cava. A sinus venosus ASD often accompanies this lesion. In the scimitar syndrome, the right lower pulmonary vein anomalously joins the inferior vena cava.
The physiologic consequences of partial anomalous pulmonary venous drainage may be minor. If more of the pulmonary venous drainage is diverted to the right atrium, evidence of right-side volume overload will be present. Associated anomalies include sinus venosus ASD, patent ductus arteriosus, transposition of the great arteries, and pulmonic valve atresia.
A dilated coronary sinus, evidence of right ventricular (RV) and right atrial dilatation, and an ASD are sometimes the only initial abnormal echocardiographic findings and should prompt a careful search for all four pulmonary veins. The diagnosis of total anomalous pulmonary venous drainage relies on visualization of the termination of all four pulmonary veins and defining a venous confluence connecting to the right atrium, coronary sinus, or vena cava. Partial anomalous pulmonary venous drainage most often is an anomaly involving the right pulmonary vein, and the abnormal connection is usually near the right side of the atrial septum or the base of the superior vena cava and is best viewed in the bicaval view. Agitated saline injection proximal to the anomalous connection with the superior vena cava may be useful in delineating the anomalous pulmonary vein from the superior vena cava (Figure 18–4). Color Doppler aids in the detection of individual veins, the direction of flow, and the presence of turbulence. Spectral Doppler confirms or excludes pulmonary venous obstruction. Surgically corrected lesions may require reinvestigation with color and pulsed-wave Doppler to determine the patency of the venous connection. Normal pulmonary vein flow should have a biphasic systolic–diastolic pattern with a maximal velocity of less than 1 m/s. A continuous nonphasic pattern and a peak velocity of greater than 2 m/s signifies restrictive pulmonary venous flow.4
Figure 18-4.

Anomalous pulmonary venous drainage into the superior vena cava (left). Injection of agitated saline solution proximal to the anomalous connection with the superior vena cava shows no contrast (right) within the anomalous pulmonary vein, indicating that it is a vessel distinct from the superior vena cava. (AO, ascending aorta; APV, anomalous pulmonary vein; RPA, right pulmonary artery; SVC, superior vena cava.)
Four possible atrial arrangements can be encountered: (1) situs solitus—normal arrangement of right and left atrium; (2) situs inversus—right atrium is on the left and the left atrium is on the right; (3) right atrial isomerism—bilateral right atria; and (4) left atrial isomerism—bilateral left atria. The morphological right atrium and left atrium can be differentiated by the anatomy of the atrial appendages. The right atrial appendage is broad, whereas the left atrial appendage is narrow and pointed.
Complex CHD lesions may demonstrate one or two ventricular chambers, ventricular inversion with the RV on the left and LV on the right (atrioventricular discordance), or abnormal arterial connections to the ventricles (ventriculoarterial discordance). Hence, the ventricles need to be identified by their structure and not by their positions or connections.6 The morphologically right and left ventricles have distinctive features, which are summarized in Table 18–1. Atrioventricular valves are always associated with their respective ventricles; therefore a tricuspid valve will identify the right ventricle and a mitral valve the left ventricle.
Right Ventricle | Left Ventricle |
---|---|
Heavily trabeculated | Smooth walls |
Three papillary muscles | Two papillary muscles |
Moderator band | No moderator band |
Tricuspid valve has chordal attachments to ventricular septum | Mitral valve has no chordal attachment to ventricular septum |
Tricuspid valve has three leaflets | Mitral valve has two leaflets |
Tricuspid valve has a more apical septal insertion point | Mitral valve has a higher septal insertion point |
ASD is the second most common congenital heart defect, occurring in women two to three times as often as in men. ASDs include the following types: ostium secundum (70%), ostium primum (20%), sinus venosus (10%), and coronary sinus (rare).
Although not a “true” ASD, a patent foramen ovale (PFO) persists secondary to failure of fusion of the septum primum and secundum (Figure 18–5). The prevalence of probe patent PFO is as high as 26% in autopsy series. Clinical problems attributed to PFO include paradoxical embolism leading to cerebrovascular accidents, decompression illness in divers, and migraine headaches. In a meta-analysis, the presence of a PFO alone increased the risk of recurrent cerebrovascular events fivefold, with an even higher risk in the presence of an atrial septal aneurysm.7 Other identified risk factors are the size of the PFO, the number of microbubbles in the left atrium during the first seconds after release of a Valsalva maneuver, and the presence of a eustachian valve directed toward the PFO,8 hence the rationale of PFO closure to protect against recurrent strokes. Elective surgical closure of a PFO diagnosed as an incidental finding during routine intraoperative TEE is not currently thought to be of benefit unless the patient has experienced a prior stroke of uncertain etiology or as a result of known paradoxical embolism. Some surgeons choose to repair a PFO if the atrium is to be opened as part of the scheduled surgical procedure. However, it should be noted that, even when the PFO is surgically repaired, the risk of recurrent neurologic events is not completely eliminated. Catheter-based PFO closure can be performed as an outpatient procedure, is associated with minimal risk, and has superseded surgical closure.9
Ostium secundum defects are located in the mid-portion of the interatrial septum in the region of the fossa ovalis and are associated with mitral valve prolapse and mitral regurgitation. Ostium primum defects are located at the inferior portion of the interatrial septum and are associated with a cleft mitral valve with variable degrees of mitral regurgitation. Sinus venosus defects may be of the superior or inferior vena caval type. Most defects in this category are located near the entrance of the superior vena cava and right pulmonary veins high in the atrial septum (superior vena caval type) and are associated with anomalous return of the right upper and lower pulmonary veins (Figure 18–6). The relatively uncommon defects in the inferior vena caval–atrial junction are characterized by a deficiency of the inferior limbic septum. Coronary sinus defects occur from a partial or complete absence of the roof of the coronary sinus, creating a left-to-right shunt from the left atrium to the coronary sinus and then into the right atrium. These lesions are associated with a persistent left superior vena cava. The fundamental physiology of all these lesions is a transatrial shunt, and the direction and magnitude of shunting is determined by the size of the defect and the relative compliance of the ventricles.
TEE provides excellent interrogation of the interatrial septum, with a sensitivity exceeding that of transthoracic echocardiography for the detection of ASDs. Two-dimensional interrogation of the entire atrial septum should be performed in transverse and longitudinal planes to ensure that small defects at the margins of the septum are not missed. The dimension of and the relative size of the defect to the entire interatrial septum should be determined. The best views are midesophageal four chamber, two chamber, and bicaval.
Secundum defects are located in the midportion of the interatrial septum in the region of the fossa ovalis (Figure 18–7), and ostium primum defects are located at the inferior portion of the interatrial septum (Figure 18–8). The bicaval view is particularly useful in detecting inferior and superior sinus venosus ASDs (Figure 18–9). The least common type of atrial septal defect, the coronary sinus communication, is defined by an enlarged coronary sinus with a deficient roof (unroofed coronary sinus), and is found in association with a persistent left superior vena cava. In the transverse axis, this is seen as an echo-free space wedge between the left upper pulmonary vein and left atrial appendage, and in the longitudinal plane, it can be identified as it enters the coronary sinus.
Figure 18-9.

A sinus venosus defect in the posterior portion of the interatrial septum near the entrance of the superior vena cava is often best visualized with the bicaval view. A central venous catheter is seen passing through the superior vena cava into the right atrium. Arrows point to the margins of the defect. (LA, left atrium; RA, right atrium; SVC, superior vena cava.)
Color-flow Doppler allows for evaluation of flow across the defect and the detection of mitral or tricuspid regurgitation. Saline contrast injection may be useful in confirming right-to-left shunting and aid in detection of small lesions, but it must be used cautiously in light of the potential for cerebral embolism. Spectral Doppler is used to assess the hemodynamic consequences of the lesion. Measurement of the tricuspid regurgitant jet velocity can estimate systolic pulmonary artery pressures and diagnose pulmonary hypertension. In the absence of significant valvular disease, the shunt magnitude can be determined by measuring the velocity-time integrals (VTIs) across the RV and LV outflow tracts. A ratio of pulmonary to systemic flow (Qp/Qs) of greater than 1.5 is considered significant. Further intraoperative evaluation includes detection of associated lesions, assessment of adequacy of surgical repair and postoperative atrioventricular valve competency, and evaluation of ventricular function.
Even though VSDs are the most common congenital anomaly recognized at birth, they account for only 10% to 15% of defects observed in adults with CHD.10 VSDs can occur in isolation or as part of complex lesions, and are classified by location into four major groups: (1) supracristal (also known as subarterial, outlet, subpulmonic, doubly committed, and infundibular); (2) infracristal (perimembranous); (3) muscular; and (4) atrioventricular canal (inlet) types (Figure 18–10).
The perimembranous type (Figure 18–11) accounts for 70% of all VSDs and involves the membranous septum. The defect is adjacent to the aortic valve and the annulus of the tricuspid valve contributes to the rim of the defect. When the VSD is primarily adjacent to the tricuspid valve, it is called a perimembranous inlet defect but when the defect extends primarily toward the aortic valve, it is referred to as a perimembranous outlet defect. In an inlet defect, shunting of blood occurs from the LV outflow tract to the right ventricle just beneath the septal leaflet of the tricuspid valve. There may be an associated tricuspid valve aneurysm or redundant tricuspid septal leaflet tissue that may plug the defect. In 10% of cases, a perimembranous defect can undermine the right aortic cusp, causing herniation of the cusp and aortic insufficiency. Rarely, a perimembranous VSD may lead to the formation of a communication between the LV outflow tract and the right atrium known as Gerbode defect. Small membranous VSDs may close spontaneously during childhood by approximation of the tricuspid valve septal leaflet across the defect. This defect closure may be undetectable in adulthood, or a residual anatomic abnormality, a “ventricular septal aneurysm,” may be visualized at the closure site.
Muscular-type VSDs (20%) are located in the central (Figure 18–12) or apical trabecular portion of the septum. They are often quite large, isolated, or multiple, and can be associated with pulmonary vascular disease. In some cases, RV outflow tract hypertrophy occurs in association with the VSD and serves to limit the severity of pulmonary hypertension (known as Gasul phenomenon).
The crista supraventricularis can be considered synonymous with the infundibular septum—the muscle separating the outflow tracts of the left and the right ventricles. The supracristal defects (5%) are usually circular and are located within the infundibular portion of the right ventricular outflow tract. The superior edge of the VSD is the conjoined annulus of the aortic and pulmonary valves; both the outlet septum and septal component of subpulmonary infundibulum are absent. Thus, the superior rim may have a direct relationship with the right coronary cusp of the aortic valve such that the right aortic leaflet may prolapse into the VSD, resulting in functional restriction of the size of the VSD but worsening aortic regurgitation. Even in the presence of only mild aortic regurgitation and a small VSD, this defect should be surgically closed to prevent rapid progression of aortic valve regurgitation.11
The atrioventricular canal or inlet type defects (5%) are seen close to the atrioventricular valves in the posterior or inlet portion of the septum and usually are caused by a defect in the formation of the atrioventricular septum. As such, they can be a part of a complex defect (see below), and are associated with a cleft in the mitral or tricuspid valve, common atrioventricular valve, and fibrous aneurysms.
The physiologic consequences of these lesions are determined by the size of the defect and the relative vascular resistance in the pulmonary bed. Patients are also at a higher risk for infective endocarditis.
Two-dimensional echocardiographic assessment is focused on defining the anatomic abnormality, whereas color-flow Doppler enhances the sensitivity of detection of all forms of VSD and helps to determine the magnitude and direction of the shunt. The ventricular septum is best interrogated in the ME four-chamber view, ME long-axis (LAX) view, ME RV inflow-outflow view, and the transgastric short-axis (TG SAX) view.4 The ME RV inflow-outflow view is especially helpful in differentiating a perimembranous VSD from a supracristal VSD (Figure 18–13). Unlike the more common perimembranous type of VSD, a supracristal VSD does not lie near the tricuspid valve, and the tricuspid valve is not involved in partial closure of the defect. Color Doppler of a supracristal defect in this view shows left-to-right shunting with turbulent flow directed into the pulmonary outflow tract. It is important to remember that this defect may be missed in the ME four-chamber view. Distortion of the right aortic leaflet and aortic regurgitation may be the only clue to the presence of a significant supracristal defect.
“Ventricular septal aneurysms” are characterized on M-mode echocardiography by a pattern of multiple linear echoes moving into the right ventricle during systole. Two-dimensional imaging reveals a saccular protuberance with a rapid flicking motion extending into the right ventricle during systole and realigning with the ventricular septum during diastole.
For all types of VSDs, continuous-wave Doppler helps in measuring the peak jet velocity, and hence estimating RV systolic pressures and pulmonary artery systolic pressures. The pressure gradient between the right and left ventricle can be calculated by using the simplified Bernoulli equation as follows:
where PLV is the LV systolic pressure, which equals the aortic systolic blood pressure in the absence of LV outflow obstruction, and PRV is the RV systolic pressure, which equals the pulmonary artery systolic blood pressure in the absence of RV outflow obstruction.
The intracardiac shunt can be quantified by calculating the stroke volume across the pulmonic and aortic valves to develop the Qp/Qs ratio. The location for determining the stroke volume depends on the location of the shunt. Qp is typically measured at the level of the RV outflow tract, whereas Qs is measured at the level of the LV outflow tract. A Qp/Qs of 1.5 or greater is considered significant. The shunt volume (different from the shunt fraction) is the product of the cross-sectional area of the color-flow jet at the defect and the flow-velocity integral of the continuous-wave Doppler systolic flow signal.12
Postoperatively, residual shunting across the patch has to be excluded. A large dehiscence of the patch (>3 mm) is an indication for immediate surgical revision. The tricuspid valve also needs to be investigated carefully as surgical repair of a VSD through the right atrium may involve detachment of the septal tricuspid leaflet and reconstruction of the leaflet once the VSD is repair is completed. Also, both the right coronary cusp and the septal tricuspid leaflet can be tethered during repair of a perimembranous VSD.4
Also known as atrioventricular septal defects (AVSDs) or endocardial cushion defects, these lesions are produced by anomalies of the atrial and ventricular septa and the adjacent parts of the atrioventricular (AV) valve. Three types are described: (1) partial AVSDs, consisting of two separate atrioventricular valves, an ostium primum ASD, as well as a cleft mitral valve; (2) transitional AVSDs, made up of an ostium primum ASD, a small ventricular septal defect, and two distinct atrioventricular valves; and (3) complete AVSDs, the most common defect, made up of an ostium primum ASD, inlet-type VSD, and a common atrioventricular valve that bridges both the right and the left sides of the heart, creating superior (anterior) and inferior (posterior) bridging leaflets. The Rastelli classification describes three types of complete AV canal defects based on the morphology of the anterior (superior) bridging leaflet, its degree of bridging, and its chordal attachments (Table 18–2).13 These bridging leaflets may have chordal insertions into both ventricles. In such cases it must be surgically divided into left and right portions and resuspended from a central patch to create separate atrioventricular orifices.
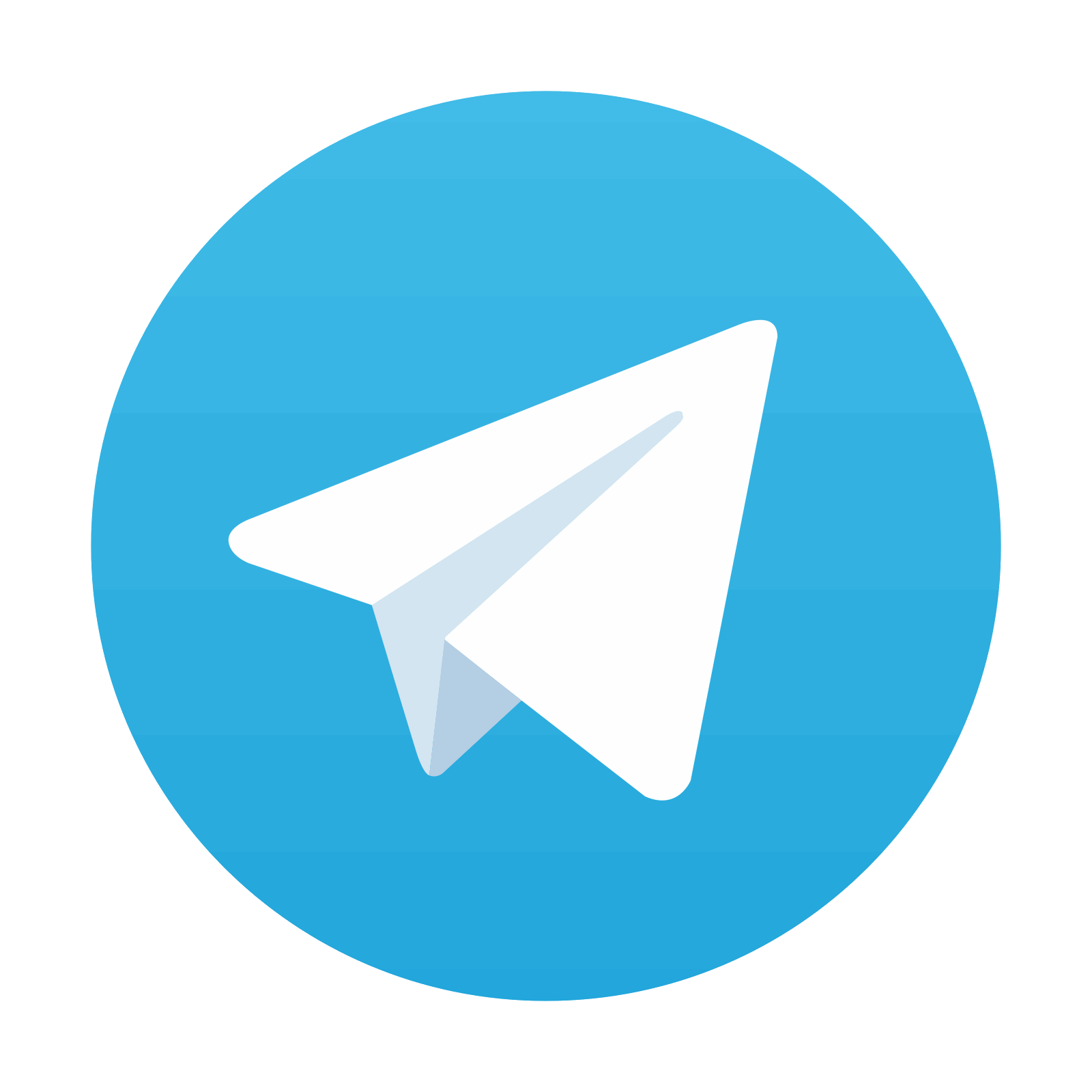
Stay updated, free articles. Join our Telegram channel

Full access? Get Clinical Tree
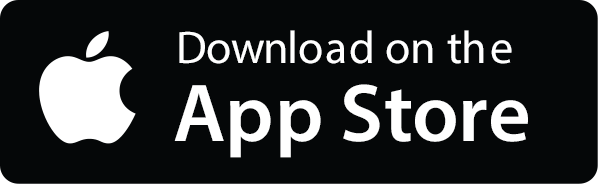
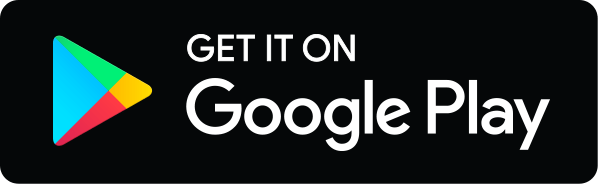