Acetaminophen Poisoning
Acetaminophen is estimated to be responsible for 51% of all cases of acute liver failure in the United States (6). Acetaminophen ingestions require ICU admission when hepatotoxicity is established. The analgesic effects of acetaminophen are mediated by central cyclo-oxygenase (COX)-2 and prostaglandin synthase inhibition (7). The metabolism of acetaminophen occurs principally in the liver and less than 5% of acetaminophen is eliminated unchanged in the urine. Ninety percent of absorbed acetaminophen undergoes hepatic conjugation with either glucuronide or sulfate to produce inactive metabolites. The remainder (5% to 15%) is oxidized by the cytochrome P450, forming N-acetyl-p-benzoquinoneimine (NAPQI), a toxic oxidant (8). Thiol-containing compounds, such as reduced glutathione, are used as electron donors to detoxify NAPQI.
TABLE 151.2 Selected Antidotes with Common Doses | |
![]() |
The single dose of acetaminophen generally thought to produce toxicity is at least 150 mg/kg (8). In overdose, absorption of acetaminophen may be delayed, although peak absorption generally occurs at 2 hours, and rarely after 4 hours (9,10). Absorption may be expected to be further delayed in the presence of peristalsis-decreasing opioid or anticholinergic co-ingestants, or if the acetaminophen is formulated for extended release. In overdose, metabolism by sulfation becomes saturated, and the formation of NAPQI exceeds that which can be detoxified by available glutathione (11). Because the toxic metabolite is formed in the liver, hepatic toxicity is the key clinical feature. N-acetylcysteine (NAC), the key to management of acetaminophen poisoning, acts as a precursor to glutathione synthesis, a substrate for sulfation; it directly binds to NAPQI itself; and enhances the reduction of NAPQI to acetaminophen (12).
Clinical Manifestations
Acute acetaminophen toxicity has been divided into four clinical stages (13). Not every untreated patient will advance through each of these stages. Spontaneous improvement is possible at any point, but the stages of toxicity serve as a useful guide to the progression of symptoms. During stage I, the patient is either asymptomatic or has nonspecific clinical findings (nausea, vomiting, malaise), and no laboratory abnormalities are recognized. Stage II begins with the onset of liver injury, generally within 24 hours but always within 36 hours of ingestion (14). Initial laboratory findings include elevated aminotransferases (aspartate aminotransferase [AST]/alanine aminotransferase [ALT]), but progress to signs of hepatic dysfunction, including prolonged prothrombin time (PT), metabolic acidosis, and hypoglycemia. Stage III represents the time of peak hepatotoxicity, usually 72 to 96 hours from ingestion. While AST and ALT may ultimately exceed 10,000 IU/L, creatinine, lactate, phosphate, and PT are better indicators of prognosis. Fatalities usually occur within 3 to 5 days of ingestion. When death does not occur, hepatic recovery is referred to as stage IV. Hepatic regeneration will be histologically and functionally complete in survivors.
Management
Decontamination with activated charcoal should be considered if significant co-ingestants are suspected. N-acetylcysteine is the key to managing acetaminophen poisoning and is available for both oral and intravenous (IV) administration. The oral protocol for acute ingestions is a 140 mg/kg loading dose, followed by 17 doses of 70 mg/kg every 4 hours for a total of 72 hours. The IV regimen is 150 mg/kg over 45 minutes, followed by 50 mg/kg over 4 hours, and then 100 mg/kg over 16 hours. Both regimens have equal efficacy for simple acute ingestion, but the IV regimen has the advantage of a shorter course, and is the only route that has been studied adequately in patients with hepatic failure. Unlike oral NAC, however, parenteral NAC carries the risk of anaphylactoid reactions. The duration and route of treatment should be determined by the patient presentation.
For simple, acute ingestions, the serum acetaminophen concentration should be plotted against the number of hours following ingestion on the Rumack–Matthew nomogram to determine whether treatment with NAC is necessary (15). The treatment line is a sensitive, but not specific, predictor of hepatotoxicity. The currently recommended line intersects 150 μg/mL at 4 hours, incorporating a 25% safety margin over the original nomogram line, which was itself nearly 100% sensitive for predicting hepatotoxicity. When the concentration at a specific time is plotted above the line, treatment is required. When treatment is initiated within 8 hours of ingestion, NAC has complete efficacy in preventing hepatotoxicity (16), and so should be started immediately when the laboratory result for the acetaminophen concentration is not expected to be available within 8 hours of the initial ingestion. Once the serum acetaminophen concentration is available, the decision whether to continue NAC can be reassessed based on the nomogram (Fig. 151.1). When there is uncertainty with regard to the exact time of ingestion, the physician should use the most conservative estimate (i.e., the earliest possible time) when using the nomogram. The risk of inadvertently failing to treat because of an incorrect history is mitigated by the safety margin associated with the nomogram.
The literature is less clear on the indications for the use of NAC for hepatotoxicity following suspected chronic acetaminophen use. The vast majority of people who take acetaminophen have no adverse clinical manifestations. Clinical trials involving daily dosing of 4 g of acetaminophen in both alcoholics and nonalcoholics showed that patients either have normal aminotransferase concentrations or very minor increases (17,18). Despite its safety, hepatotoxicity from chronic use occurs. Because chronic acetaminophen use often occurs in the setting of comorbid conditions, the diagnosis can be difficult to establish with certainty. NAC should be administered to all patients with suspected acetaminophen hepatotoxicity until the diagnosis has been excluded.
The nomogram cannot be used for patients who present more than 24 hours after ingestion. In such cases, NAC should be started immediately upon presentation. If the patient has both an undetectable acetaminophen concentration and normal aminotransferases, acetaminophen overdose is highly unlikely, and NAC need not be continued. If either acetaminophen or aminotransferase concentrations are elevated (even minimally so), the patient should be administered 20 hours of IV NAC. Following the treatment period, aminotransferase and acetaminophen concentrations should be obtained again; at this point, if the aminotransferase concentrations are only minimally elevated, the patient was either minimally poisoned or acetaminophen was not the cause of the liver damage.

FIGURE 151.1 Acetaminophen nomogram. (Adapted from Hendrickson RG, Bizovi KE. Acetaminophen. In: Flomenbaum NE, Goldfrank LR, Hoffman RS, et al., eds. Goldfrank’s Toxicologic Emergencies. 8th ed. New York: McGraw-Hill; 2006:523–543.)
When acetaminophen-induced hepatotoxicity is encountered, IV NAC should be administered as described above, but the maintenance dose should be continued until clinical improvement, liver transplantation, or death occurs. Even in the presence of fulminant hepatic failure, IV NAC has been shown to decrease mortality, cerebral edema, and the need for vasopressors (19).
Hepatic Transplantation
The long-term complications of a transplant are significant, and those who survive acetaminophen poisoning without transplant will make a complete recovery. Under ideal conditions, the clinician could immediately determine which patients would survive without transplant and which would not, so that the appropriate patients could be candidates for a liver transplant before irreversible clinical deterioration; in practice, it is not always clear. Several prognostic criteria are available. The King’s College Hospital Criteria suggest that pH less than 7.3 after resuscitation or the combination of PT more than 100, creatinine more than 3.3 mg/dL, and grade III or IV encephalopathy are predictive of death in the absence of a transplant (20). Alternatively, a 48-hour serum phosphate concentration more than 1.2 mmol/L has also been shown to be sensitive and specific for predicting the need for transplant and the probability of death from acetaminophen hepatotoxicity (21). Presumably, a low or normal phosphate concentration is an evidence that the phosphate is being utilized by hepatocytes for adenosine triphosphate (ATP) generation.
SALICYLATES AND OTHER NONSTEROIDAL ANTI-INFLAMMATORY DRUGS
The nonsteroidal anti-inflammatory drugs (NSAIDs) are widely available both with and without prescription for relief of inflammation, pain, and fever. Salicylates are a subgroup of NSAIDs that have unique features of toxicity and require distinct management. In this chapter, we will use the term NSAID to refer to nonsalicylate NSAIDs.
The therapeutic effects of salicylates and NSAIDs result from the inhibition of COX, a mediator of prostaglandin synthesis. In general, they are renally eliminated. Because they are designed to promote fast relief, therapeutic doses of the immediate-release drugs produce significant concentrations within an hour. However, when taken in overdose or as enteric-coated or sustained-release formulations, absorption may be delayed and maximal serum concentrations may not be observed for hours after the ingestion.
In addition to these effects, salicylates also uncouple oxidative phosphorylation, meaning that some of the proton gradient across the mitochondrial matrix is dissipated in the formation of heat, rather than ATP, forcing the production of lactate. As a result, ICU admission is required when patients present with metabolic acidosis and hemodynamic instability, or if they require bicarbonate infusion or frequent measurements of salicylate concentration.
Salicylate Poisoning
Clinical Manifestations
Acute salicylate poisoning may cause epigastric pain, nausea, and vomiting. Salicylates induce hyperventilation (both tachypnea and hyperpnea) by direct stimulation of the brainstem respiratory center (22). Neurologic signs and symptoms of salicylate poisoning range from mild to severe, and include tinnitus, delirium, coma, and seizure. The initial feature of toxicity is primary respiratory alkalosis. A primary metabolic acidosis is characterized by the presence of lactic acid, ketoacids, and salicylic acids (23); the net result is an increased anion gap metabolic acidosis. The simultaneous presence of a respiratory alkalosis and metabolic acidosis can be difficult to interpret. Because the respiratory alkalosis initially predominates in adults, the presence of an acidemia or even normal pH indicates advanced poisoning.
Chronic salicylate poisoning presents with the same signs and symptoms as acute poisoning, but typically occurs in elderly patients taking supratherapeutic amounts of salicylate to treat a chronic condition. Chronic poisoning can be a challenging diagnosis to establish because it is often not suspected. Elderly salicylate-poisoned patients presenting with metabolic acidosis and an altered sensorium may be initially misdiagnosed with sepsis, dehydration, or cerebrovascular accident (CVA) if a salicylate concentration is not obtained.
Serum salicylate concentrations only correlate loosely with toxicity because the principal site of poisoning is the central nervous system (CNS). The threshold for toxicity is usually considered to be 30 mg/dL when tinnitus develops. Chronically poisoned patients have a lower salicylate concentration for the same degree of illness because much of their total body burden has redistributed into the CNS. The degree of poisoning is determined by evaluating the serum concentration in the context of the patient’s clinical appearance, laboratory results, and acuity of the ingestion.
Management
The key principles for management of salicylate poisoning are to minimize absorption, speed elimination, and minimize redistribution to tissues. Gastric emptying should only be attempted if a significant amount of drug is expected to be present in the stomach. Activated charcoal, 1 g/kg, should be administered every 4 hours if it can be given safely. Salicylates cause pylorospasm and may form concretions in overdose, leading to delayed absorption. Multiple-dose activated charcoal (MDAC) not only prevents delayed absorption, but also may speed up the elimination of salicylates by disrupting the enteroenteric circulation of the drug (24). Serum chemistry, venous or arterial blood gas analysis, and salicylate concentration should be obtained every 2 hours until the salicylate concentration demonstrates an interval decrease.
Moderately poisoned patients (increased anion gap or a salicylate concentration >40 mg/dL) should also have blood and urine alkalinized with sodium bicarbonate. As a weak acid (pKa 3.5), salicylates will be ionized in an alkaline environment and “trapped” (i.e., unable to passively move through lipid membranes). Ionization prevents salicylate in the proximal tubule from diffusing into the plasma and salicylate in the plasma from diffusing into tissues, such as the brain (25). Alkalinization can be achieved with an infusion of sodium bicarbonate of 150 mEq in 1 L of D5W at twice the maintenance rate. The urine pH should be maintained from 7.5 to 8.0 and systemic arterial pH between 7.45 and 7.55. Close attention should be paid to potassium repletion, as low serum potassium will cause preferential reabsorption of potassium over hydrogen ions in the proximal tubule and compromise attempts to alkalinize the urine (26). Endotracheal intubation and sedation should be avoided whenever possible. The tachypnea and hyperpnea of salicylate poisoning do not necessarily represent “tiring,” and produces a helpful alkalosis. When intubation is unavoidable, patients should be administered 1 to 2 mEq/kg of bicarbonate prior to the procedure, intubated quickly, and hyperventilated afterward to avoid respiratory acidosis (27).
Early consultation with a nephrologist is recommended for seriously ill patients. Extracorporeal elimination is reserved for patients who are very ill, those who cannot tolerate alkalinization, or those with serum concentrations so elevated that their clinical status is expected to deteriorate. We recommend hemodialysis for severe acid–base disturbances, mental status changes, inability to tolerate alkalinization (renal failure or congestive heart failure), and serum salicylate concentrations of 100 mg/dL after acute poisoning and 60 mg/dL in chronic poisoning (Table 151.3).
NSAID Poisoning
Clinical Manifestations
NSAIDs are considered safer than salicylates in therapeutic dosing. While chronic NSAID use is associated with interstitial nephritis, nephritic syndrome, or analgesic nephropathy, acute overdose can cause gastric injury and is sometimes accompanied by a reversible azotemia caused by vasoconstriction from decreased prostaglandin production (28). In severe overdose, the most consequential effects are elevated anion gap metabolic acidosis, coma, and hypotension (29).
Management
Activated charcoal should be administered if the patient presents within several hours of overdose. Good supportive care is the mainstay of therapy after NSAID overdose. NSAID elimination is not increased with alkalinization and NSAIDs’ high degree of protein binding precludes removal with hemodialysis. Hemodialysis has, however, been used to correct acidemia and electrolyte abnormalities in patients with multiorgan system failure.
TABLE 151.3 Indications for Hemodialysis in Salicylate Poisoning |
![]() |
PSYCHIATRIC MEDICATIONS
Psychiatric medications represent a disproportionate number of poisonings in the United States. Antidepressants, antipsychotics, and sedative–hypnotics accounted for more than 20% of all deaths reported to poison control centers in 2013, while accounting for only 5.9% of total exposures (3).
Antipsychotic Poisoning
The antipsychotics are categorized as either typical or atypical. The typical antipsychotics, which include haloperidol, chlorpromazine, and thioridazine, antagonize dopamine primarily at the D2 receptor. The newer, atypical, medications are exemplified by clozapine, olanzapine, quetiapine, risperidone, and ziprasidone, which have less dopaminergic antagonism and more serotonergic effects than the typical antipsychotics. When antipsychotic medications produce coma, conduction abnormalities, or hyperthermia, these patients require ICU admission.
Clinical Manifestations
All antipsychotics produce sedation in overdose, though respiratory depression is usually not consequential. The drugs have varying degrees of muscarinic and α-adrenergic antagonism, often resulting in tachycardia and moderate hypotension (30). Many of the typical antipsychotics have type-IA antidysrhythmic properties; most of the typical and a few of the atypical drugs (notably ziprasidone) can cause QTc prolongation and torsades de pointes (31,32). Management of overdose of the antipsychotics generally only requires supportive care.
Neuroleptic Malignant Syndrome
The dopamine antagonism required for control of psychosis can cause a group of distinct movement disorders that range in severity from mild to life threatening. These conditions—dystonia, akathisia, parkinsonism, tardive dyskinesia, and neuroleptic malignant syndrome (NMS)—are more likely to occur in the presence of the typical antipsychotics, although the atypical antipsychotics can cause them as well. The first four conditions mentioned above are of less concern to the intensivist than NMS, and will not be discussed.
NMS is characterized by the presence of altered mental status, muscular rigidity, hyperthermia, and autonomic dysfunction (33). While symptoms usually begin within weeks of starting treatment, they do occur in individuals that are taking the drug on a chronic basis. Risk factors include young age, male gender, extracellular fluid volume contraction, use of high-potency antipsychotics, depot drug preparations, concomitant lithium use, rapid increase in dose, or simultaneous use of multiple drugs (32). Diagnosis is not always clear because there is no reference standard. Other diagnoses to consider include infection, environmental hyperthermia, hyperthyroidism, serotonin syndrome, ethanol and sedative–hypnotic withdrawal, sympathomimetic intoxication, and anticholinergic intoxication.
Management of NMS begins with immediate treatment of life-threatening hyperthermia. Ice–water immersion and paralysis by neuromuscular blockade should not be delayed if temperature is more than 106°F (41.1°C). In instances of environmental hyperthermia, a delay of cooling longer than 30 minutes has been associated with significant morbidity and mortality (34). Benzodiazepines should be titrated to sedation and muscle relaxation. When present, rhabdomyolysis, electrolyte disorders, and hypotension should be aggressively treated.
Bromocriptine, a centrally acting dopamine agonist given at 2.5 to 10 mg three to four times a day, may be of theoretical benefit even though it is not well studied and may take days to control symptoms. After evidence of clinical improvement, bromocriptine should be decreased by no more than 10% a day since decreasing the dose too rapidly may precipitate a relapse of NMS. We do not recommend dantrolene, the drug of choice for malignant hyperthermia, in the management of NMS.
Benzodiazepine Poisoning
Benzodiazepines are widely used for their sedative, anxiolytic, and anticonvulsant properties, which are moderated by the frequency of opening of γ-aminobutyric acid (GABA)-mediated chloride channels in the CNS (35). In overdose, these drugs produce somnolence, coma, and minimal decreases in blood pressure, heart rate, and respiratory rate.
Management
Management of benzodiazepine overdose is supportive. Care of the comatose patient should focus on supporting the airway and blood pressure while waiting for the drug to be eliminated. There is a limited role for flumazenil, a competitive benzodiazepine antagonist; flumazenil can precipitate withdrawal in individuals who are tolerant to benzodiazepines and induce seizures in those with seizure disorders (36,37). Flumazenil may be indicated in patients without tolerance to benzodiazepines, such as children, who suffer from benzodiazepine overdose. When indicated, flumazenil should be given intravenously, 0.1 mg/min, up to 1 mg and can be repeated if the clinical response is inadequate. Because the duration of the effect of flumazenil is shorter than the effect of the benzodiazepine, recurrence of symptoms should be expected.
If there is any doubt as to whether the patient has tolerance to benzodiazepines, flumazenil should not be administered. Benzodiazepine poisoning can be managed effectively and safely with supportive care only, but benzodiazepine withdrawal precipitated by flumazenil can be life threatening.
Cyclic Antidepressant Poisoning
Until the introduction of the selective serotonin reuptake inhibitors (SSRIs), the cyclic antidepressants (CAs) were the principal pharmacologic treatment available for depression. In 2013, 13,037 CA exposures were reported to the AAPCC, with tricyclic antidepressants accounting for 2.27% of total fatalities (3). While the CAs differ slightly from each other in their receptor affinities, they can be treated as a group.
The CAs are usually absorbed within hours of ingestion, although the antimuscarinic effects may delay absorption in overdose. The drugs also exhibit α-adrenergic antagonism, inhibition of reuptake of norepinephrine, and anticholinergic properties. Acting as type-IA antidysrhythmics, CAs block sodium entry into myocytes during phase 0 of depolarization. Acute ingestions of 10 to 20 mg/kg of most CAs can cause significant poisoning (38).

FIGURE 151.2 Terminal elevation of aVR and QRS prolongation. (From Clancy C. Electrocardiographic principles. In: Flomenbaum NE, Goldfrank LR, Hoffman RS, et al., eds. Goldfrank’s Toxicologic Emergencies. 8th ed. New York: McGraw-Hill; 2006:51–62.)
Clinical Manifestations
Important CNS effects include lethargy, delirium, coma, and seizures. Tachycardia and hypotension develop early in toxicity. The IA antidysrhythmic properties cause prolongation of the QRS interval. CAs also produce a characteristic rightward shift of the axis in the terminal portion of the QRS, best seen as an R wave in the terminal 40 milliseconds of lead aVR (Fig. 151.2) (39). Serum drug concentrations may be obtained but do not correlate well with toxicity (40).
Management
Gastrointestinal decontamination should be considered in every patient. If the history suggests a recent large ingestion, activated charcoal should be administered and gastric lavage may be attempted. The ECG is the most important diagnostic test when managing CA overdose. A terminal 40-millisecond QRS axis of 130 to 270 degrees discriminated patients with CA toxicity from those without toxicity in one study (41). While the terminal 40-millisecond QRS axis is a good indicator of exposure, QRS duration is a better indicator of severity of poisoning. In another series, no patients with QRS less than 160 milliseconds had ventricular dysrhythmias and no patients with a QRS duration less than 100 milliseconds had seizures (40).
Sodium bicarbonate should be administered if the QRS duration is 100 milliseconds or more. Both the high sodium concentration and alkaline pH of sodium bicarbonate solution are responsible for its salutary effects. The sodium load increases the sodium gradient across the poisoned myocardial sodium channel, resulting in a narrowing of the QRS complex. The bicarbonate raises the pH, reducing CA binding to the sodium channel. Sodium bicarbonate should be administered as a 1- to 2-mEq/kg bolus during continuous ECG monitoring. If the complex narrows, sodium bicarbonate can be administered as an infusion. If the complex remains unchanged, the diagnosis of CA poisoning should be reconsidered. The sodium bicarbonate infusion may be performed by adding 150 mEq of bicarbonate to 1 L of D5W and infusing at twice the maintenance rate, with an arterial pH target of 7.50 to 7.55. Occasional repeat boluses of 1 to 2 mEq/kg may be necessary. Hypertonic sodium chloride (3% NaCl) may be indicated when the QRS complex widens and the serum pH precludes further alkalinization. Hyperventilation may be used to induce alkalemia in intubated CA-poisoned patients and in those who cannot tolerate the fluid or sodium load from sodium bicarbonate. Although hyperventilation did not have an effect in one experimental model, it has been used clinically with success (42,43).
Seizures should be rapidly controlled as they will cause a metabolic acidosis, which will lead to even more avid binding of the CA to the cardiac sodium channels and potentially worsen cardiotoxicity. Benzodiazepines can be safely administered or, alternatively, propofol or barbiturates are also appropriate. Phenytoin, a type-IA antidysrhythmic, may worsen cardiac toxicity and is therefore not indicated (44).
If hypotension persists despite fluid resuscitation and vasopressors become necessary, norepinephrine may be superior to dopamine because intracellular catecholamines may be depleted. Therapy should continue until vital signs and ECG improve. In most instances, CA poisoning results in a rapid deterioration within hours of overdose. Unless there has been a significant secondary injury (such as from shock), those who survive to 24 hours are expected to make a complete recovery. Because of avid CA protein binding, hemodialysis is deemed ineffective.
Lithium Poisoning
Lithium is used in the treatment of bipolar affective disorders. Of 6,610 exposures reported to the AAPCC, 2.3% were classified as major or fatal (3). Patients will require ICU admission when they have signs of CNS toxicity, do not tolerate fluid therapy, or have serum concentrations more than 2 mmol/L, which may result in rapid deterioration.
Lithium is thought to increase serotonin release and increase receptor sensitivity to serotonin, as well as modulate the effects of norepinephrine on its second-messenger system (45). Lithium is freely filtered by the glomerulus, 80% of which is reabsorbed, with 60% occurring in the proximal tubule (46). Immediate-release preparations produce peak serum concentrations within hours, but sustained-release lithium may not peak for 6 to 12 hours. Acute toxicity occurs when an individual without a body burden of lithium takes a supratherapeutic dose of the drug. Chronic toxicity is usually the result of decreased elimination of the drug in a patient who is receiving a fixed dose (e.g., after developing renal insufficiency). Acute-on-chronic toxicity occurs when a patient with a pre-existent total body drug takes a supratherapeutic dose. The generally accepted therapeutic range of lithium is 0.6 to 1.2 mmol/L, although in both overdose and therapeutic dosing, clinical signs and symptoms may serve as a better guide than the serum concentration.
Diagnosis
In acute toxicity, a large ingestion of lithium—a gastrointestinal irritant—will initially cause gastrointestinal symptoms, such as vomiting and diarrhea. Neurotoxicity—which is clinically more significant—will be delayed until the drug has been absorbed and is redistributed into the CNS. In chronic toxicity, gastrointestinal symptoms may be completely absent. Neurotoxicity manifests itself as disorders of movement and alterations in mental status. In very mild toxicity, only a fine tremor will be present, but in more advanced poisoning, fasciculations, hyperreflexia, dysarthria, and nystagmus may be seen as well (46). Mental status changes range from confusion to coma and seizures (47).
Nephrogenic diabetes insipidus and hypothyroidism occur following chronic therapeutic lithium use, but are not features of overdose. The syndrome of irreversible lithium-effectuated neurotoxicity (SILENT) is a chronic neurologic disorder with many of the same features of lithium neurotoxicity. The distinction is that SILENT persists even when the body burden of lithium is eliminated. The mechanism is not completely elucidated but may involve demyelination. SILENT has been reported both as a result of chronic therapeutic use and as a sequela of lithium intoxication (48).
Management
Since lithium does not bind to activated charcoal, it should only be considered when a mixed overdose is suspected (49). When sustained-release preparations are ingested, whole bowel irrigation has been shown to decrease serum lithium concentration (50); whole bowel irrigation can be performed by administering 2 L of polyethylene glycol orally every hour (25 mL/kg/hr in children) until the rectal effluent is clear. After both acute and chronic toxicities, IV fluids should be given to optimize intravascular volume, as volume-depleted patients will have a decreased glomerular filtration rate and increased reabsorption of lithium. When fluid deficits are restored, 0.9% saline can be administered at twice the maintenance rate or approximately 200 mL/hr in adults to aid in elimination of lithium.
Lithium can be removed by hemodialysis due to its low volume of distribution and limited protein binding. Although hemodialysis can only remove the lithium residing in the vascular compartment, the elimination of serum lithium will allow the remaining intracellular lithium to redistribute into the plasma. Thus, although lithium concentrations may rebound following dialysis, the tissue burden has actually decreased. The indications for dialysis are not universally agreed upon. Hemodialysis should be performed when there are signs of significant end-organ damage, when lithium cannot be eliminated without dialysis, or when the serum concentration is elevated such that severe toxicity is highly likely. We recommend dialysis when there is significant CNS toxicity such as clonus, obtundation, coma, or seizures; when a patient with milder toxicity cannot eliminate lithium efficiently (renal insufficiency) or tolerate saline resuscitation (congestive heart failure); or in the presence of a serum lithium concentration more than 4.0 mmol/L following acute poisoning, or more than 2.5 mmol/L following chronic poisoning. Since repeat dialysis may be necessary, the clinician should reapply the above criteria 4 hours after dialysis is completed to determine if dialysis should be repeated.
A common clinical pitfall is to deny dialysis to patients with an elevated lithium concentration and signs of toxicity because consecutive lithium concentrations have shown a small decrease. The clinician concludes that the lithium will eventually be eliminated without dialysis, so dialysis should not be helpful. Unfortunately, duration of exposure to the toxic lithium levels may predispose the patient to SILENT. In other words, it is better to be exposed to a neurotoxin for a few hours than a few days. While this area is not adequately studied, it seems prudent to hemodialyze these patients.
TOXICOLOGIC BRADYCARDIA
In 2013, the AAPCC NPED reported 101,544 exposures to cardioactive medications including digoxin, β-adrenergic antagonists, and calcium channel blockers (CCBs), and accounted for 10.67% of total exposure fatalities that year (3). These xenobiotics have a narrow therapeutic index, drawing a fine line between therapeutic dosing and poisoning. The individuals who take these medications usually have underlying cardiovascular disease, making management of overdose even more challenging.
Digoxin Poisoning
Digoxin is a cardioactive steroid derived from the foxglove plant. Though digoxin and digitoxin are the only pharmaceuticals in the class, plants such as oleander, yellow oleander, dogbane, and red squill contain cardioactive steroids with similar toxicity. While some of these plants cause a great deal of morbidity worldwide, this chapter will deal primarily with digoxin, which causes more morbidity than any other cardioactive steroid in North America.
Digoxin has multiple therapeutic and toxic cardiovascular effects, all of which result from inhibition of the Na+–K+–ATPase. The Na+–K+–ATPase extrudes sodium from the myocardial cell, creating a sodium gradient that drives Na+–Ca2+–antiporters to move calcium extracellularly. The inhibition of the Na+–K+–ATPase by digoxin increases intracellular Ca2+, which therapeutically triggers Ca2+-mediated Ca2+ release from the sarcoplasmic reticulum (SR); this is commonly called Ca-mediated Ca release (Fig. 151.3).
Digoxin also slows conduction through the sinoatrial (SA) and atrioventricular (AV) nodes, probably through direct and vagally mediated mechanisms (51). In therapeutic use, digoxin decreases heart rate and increases inotropy. In overdose, the increased intracellular Ca2+ brings the cell closer to threshold, resulting in increased automaticity.

FIGURE 151.3 Normal depolarization. (Adapted from Hack JB, Lewin NA. Cardioactive steroids. In: Flomenbaum NE, Goldfrank LR, Hoffman RS, et al., eds. Goldfrank’s Toxicologic Emergencies. 8th ed. New York: McGraw-Hill; 2006:971–982.)
Digoxin does not exert its therapeutic and toxic effects until it redistributes from the serum into the myocardium. Digoxin has a large volume of distribution, precluding elimination by hemodialysis, and is mostly eliminated renally, although there is some hepatic metabolism. The maximal effect from a therapeutic dose of digoxin is seen at 4 to 6 hours when administered orally and 1.5 to 3 hours when given intravenously.
Clinical Manifestations
Digoxin poisoning can be either acute or chronic. Acute toxicity occurs when an individual without a tissue burden of digoxin ingests a supratherapeutic dosage of the drug. Chronic toxicity usually occurs when an individual on a fixed dose of the drug loses the ability to excrete it effectively. Both syndromes have similar cardiovascular manifestations, but acute toxicity may feature more prominent gastrointestinal symptoms. Acute poisoning may result in nausea, vomiting, and abdominal pain, whereas chronic poisoning develops more insidiously. In addition to gastrointestinal symptoms, chronic poisoning may present with weakness, confusion, or delirium (52,53).
Bradycardia with a preserved blood pressure typically occurs in digoxin toxicity. The ECG is the most important test in establishing the diagnosis. Because digoxin has multiple cardiac effects, there is no single ECG manifestation that is consistently seen in patients with digoxin toxicity. The most common rhythm disturbance reported is the presence of ventricular ectopy (54). The ECG could potentially exhibit increased automaticity from elevated resting potential, conduction disturbance from AV and SA nodal block, both, or neither. The ectopy may degrade into ventricular tachycardia or ventricular fibrillation. If conduction disturbance predominates, the ECG may demonstrate sinus bradycardia or varying degrees of AV block.
The laboratory provides clues to toxicity. The therapeutic range for digoxin is usually reported as 0.5 to 2.0 ng/mL. Serum digoxin concentration should be interpreted in the context of the history and ECG. Digoxin is a cardiotoxin, and serum concentrations do not necessarily reflect the degree of poisoning. Digoxin requires several hours to redistribute from the serum to the tissues. Shortly after an acute ingestion, the serum concentration may overestimate toxicity, while a mild increase in serum concentration of a patient chronically on digoxin may underestimate the high extent of the increased tissue burden.
Serum potassium concentration is a better predictor of illness following acute ingestions. A study of 91 digitoxin-poisoned patients performed in the pre–digoxin-specific antibody fragment era found no mortality when the potassium concentration was less than 5.0 mEq/L and 50% mortality when the potassium concentration was 5.0 to 5.5 mEq/L (55).
Management
Because acute toxicity can cause vomiting, activated charcoal and gastric lavage may be of limited value. Atropine can be given intravenously in 0.5-mg doses for bradycardia, although it is probably not important to “correct” the heart rate if the blood pressure is preserved. If necessary, potassium should be supplemented. Hypokalemia inhibits the function of the Na+–K+–ATPase, and thereby exacerbates digoxin poisoning. A pitfall in managing digoxin-poisoned patients is the administration of calcium in response to the recognition of hyperkalemia. When hyperkalemia is the result of an increase in total body burden of potassium, such as in renal failure, calcium is the treatment of choice. However, calcium administration is not recommended in the setting of digoxin poisoning where extracellular distribution of potassium is the result of a poisoned Na+–K+–ATPase, not an increase in total body potassium. Under these circumstances, increasing extracellular calcium may accentuate toxicity.
Digoxin-Specific Immune Fragments
Administration of digoxin-specific antibody fragments (Fab) is the most important intervention in digoxin-poisoned patients. Fab are prepared by cleaving the Fc fragment from IgG. The resulting Fab fragments are much less immunoreactive than the whole IgG antibodies. In a large series, digoxin-specific antibody fragments caused allergic reaction in 0.8% of patients (56).
Digoxin-specific Fab should be administered to anyone with digoxin-induced cardiotoxicity, a serum potassium concentration of 5.0 mEq/L or more after an acute overdose, or a serum digoxin concentration 15 ng/mL or more at any time or 10 ng/mL or more at 6 hours postingestion (57).
Digoxin-specific Fab are given by IV infusion and dosed according to serum concentration (Table 151.4). In the presence of suspected severe toxicity, treatment should not be dependent on nor await serum digoxin concentration results. The first clinical effect of Fab should be seen within 20 minutes and a maximal response within several hours (58). Following immune-specific antibody fragment administration, the serum digoxin concentration determined by most laboratories will be a total digoxin concentration, which will include the antibody-bound digoxin. The result will be a very elevated value without clinical utility. The determination of free digoxin concentration, which is available at some institutions, would not be affected.
β-Adrenergic Antagonist and Calcium Channel Blockers
β-Adrenergic Antagonist Overdose
β-Adrenergic receptors are coupled to G-proteins, which activate adenyl cyclase, resulting in increased production of ATP from cyclic adenosine monophosphate (cAMP). The cAMP activates protein kinase A (PKA), which initiates a series of phosphorylations. Phosphorylation of L-type calcium channels on cell membranes increases intracellular calcium, which allows more activation of the SR and further calcium release, causing muscle contraction. The calcium influx also brings pacemaker cells closer to threshold (59).
TABLE 151.4 Digoxin-Specific Fab Dose Calculation |
![]() |
Most of the β-adrenergic antagonists are exclusively metabolized or biotransformed in the liver and then renally eliminated. The exception to the rule is atenolol, which is exclusively renally eliminated.
Clinical Manifestations
All β-adrenergic antagonists have the potential to produce bradycardia and hypotension in a dose-dependent fashion. However, there are subtle differences among the agents in terms of receptor selectivity (α-, β1-, and β2-adrenergic receptors), lipid solubility, membrane-stabilizing activity, and potassium channel blockade that result in varied clinical manifestations.
Drugs with α- and β-adrenergic antagonist effects, such as labetalol and carvedilol, produce more hypotension and afterload reduction. The more β1-selective drugs, including metoprolol and atenolol, have less potential for β2-related adverse effects such as bronchospasm. The more lipid-soluble β-adrenergic antagonists, such as propranolol, penetrate the CNS more readily, causing obtundation or seizures prior to hemodynamic collapse (60). Membrane-stabilizing activity, similar to type-I antidysrhythmic activity, produces lengthening of the QRS interval, tachydysrhythmias, and hypotension. The membrane-stabilizing effect is usually associated with propranolol and other lipid-soluble drugs, although it has been observed after overdose with others (61,62). Sotalol and acebutolol can produce QTc prolongation due to potassium channel blockade, which may result in torsades de pointes (63).
Calcium Channel Blocker Overdose
The CCBs are formulated as both immediate and sustained release, but in overdose, the effects of either type may be prolonged. The CCBs undergo hepatic metabolism. There are three major classes of CCBs: dihydropyridines (including amlodipine, nifedipine, and others ending with the suffix “-pine”), phenylalkylamines (verapamil), and benzothiazepine (diltiazem). In practice, it is more clinically useful to divide them into two classes: the dihydropyridines and the nondihydropyridines. All of the drugs inhibit the function of L-type calcium channels. The dihydropyridines have greater affinity for calcium channels in vascular smooth muscle than the myocardium (64).
Clinical Manifestations
The most consequential clinical features of CCB overdose are cardiovascular. All of the CCBs produce hypotension, but effects on heart rate and contractility vary based on the class of the particular drug. As a result, they cause hypotension with a reflex tachycardia. Diltiazem, in contrast, produces little peripheral blockade, but does suppress contractility and conduction through the SA and AV nodes, resulting in bradycardia and decreased inotropy. These cardiac effects can be much more difficult to treat than the peripheral vasodilation of the dihydropyridines. In severe poisoning, heart block or complete cardiovascular collapse results. Verapamil, which is active in the peripheral vasculature and in the myocardium, produces a combination of the effects of the dihydropyridines and diltiazem. For this reason, verapamil is considered to be the most dangerous of the CCBs, although any of them can cause death in overdose.
CCBs have effects outside the cardiovascular system. The blockade of L-type calcium channels in pancreatic β-islet cells, where they trigger the blockade of insulin release, may result in hyperglycemia (65).
Management
Patients who initially present without symptoms of β-adrenergic antagonists or CCB overdose may rapidly become very ill. Patients with these overdoses should be taken very seriously and treated aggressively. Many antidotes have been investigated, with varying degrees of clinical success. Patients with β-adrenergic antagonist and CCB overdose do not benefit from removal with hemodialysis. There is no single antidote for either β-adrenergic antagonists or CCBs. The optimal treatment consists of a combination of the treatments described below.
Gastrointestinal decontamination should be considered in all patients. Gastric lavage may be indicated if there are pills still expected to be in the stomach—usually in the first hour or two after ingestion; activated charcoal should be administered. Whole bowel irrigation with polyethylene glycol is indicated for patients with a history of ingesting sustained-release drugs.
The initial management for hypotension will be IV crystalloid fluid. Although IV atropine, 0.5 to 1 mg, may be given for bradycardia, studies of atropine efficacy in CCB toxicity are not definitive (66). Calcium has a role not only in CCB toxicity, but also for β-adrenergic antagonist poisoning (67,68). Increasing extracellular calcium helps to overcome calcium channel blockade and increase intracellular calcium, typically with greater improvement in blood pressure than heart rate. The ideal dosing of calcium is not yet established. An IV bolus of 13 to 25 mEq of Ca2+ (10 to 20 mL of 10% calcium chloride or 30 to 60 mL of 10% calcium gluconate) can be followed by repeat boluses or an infusion of 0.5 mEq/kg/hr of Ca2+ (69); calcium concentration should be closely monitored.
Glucagon, an endogenous polypeptide hormone released by pancreatic α-cells, has significant inotropic effects mediated by its ability to activate myocyte adenylate cyclase, effectively bypassing the β-adrenergic receptor (70). Because calcium channel opening occurs “downstream” from adenylate cyclase, glucagon may not be as effective for overcoming calcium channel blockade. Glucagon should be given intravenously, at an initial dose of 3 to 5 mg (50 μg/kg in children), up to 10 mg. The total initial dose that produces a response should be given hourly as an infusion (59). Glucagon may cause hyperglycemia or vomiting, but neither complication should limit the therapy if it is effective.
Hyperinsulemia/euglycemia therapy should be instituted early in patients with moderate to severe poisoning. Insulin is a positive inotrope and may independently increase Ca2+ entry into cells (71) and allow the myocardium, which usually relies on fatty acids, to use more carbohydrate for metabolism (72). Although the ideal dose is not known, we recommend an IV bolus of 1 unit/kg, followed by an infusion of 0.5 to 1 unit/kg/hr. The initial bolus should be preceded by a 1-g/kg bolus of dextrose, followed by a 0.5-g/kg/hr infusion of dextrose to maintain euglycemia titrated to subsequent glucose concentrations. Although some clinicians are understandably apprehensive about using a dose of insulin that is 10-fold greater than the typical diabetic ketoacidosis regimen, the regimen has been demonstrated to have utility in both clinical and animal testing (73,74).
In severe poisoning, all of the above measures should be performed, as well as institution of inotropes and vasoactive drugs. Intra-aortic balloon counterpulsation should also be considered if cardiac output is severely compromised. These patients may be ideal for this procedure because, unlike with most other causes of cardiogenic shock, their cardiac output can recover in a relatively short period of time.
Controversies
Intravenous lipid emulsion (ILE) therapy, a component of total parenteral nutrition, has been proposed as an adjunctive therapy for β-adrenergic antagonist and CCB toxicity in critically ill patients; the exact mechanism is poorly understood. Theories include the induction of an intravascular “lipid sink,” or alternative lipid binding surface for the xenobiotic, effectively inactivating a percentage of the drug; evidence is limited to animal models and case reports. Clinical trials are lacking (75). ILE infusion may be considered after all alternative supportive methods—calcium, glucagon, fluids, insulin, vasopressors—have been attempted.
TOXIC ALCOHOL POISONING
The term toxic alcohols refers in particular to methanol and ethylene glycol, which are the most important chemicals in the class because they are both of high potential toxicity and wide availability. In 2013, the NPED received 6,600 reports of exposure to ethylene glycol, including 2.9% classified as major or fatal, and 1,784 exposures to methanol, with 1.3% major or fatal (3). When suspected, toxic alcohol poisoning requires ICU admission.
Methanol is commonly found in windshield wiper fluid, ethylene glycol in automobile antifreeze, and isopropanol is a ubiquitous topical disinfectant. The toxic alcohols are readily absorbed and have a volume of distribution similar to total body water. Both the parent compounds and the toxic metabolites are dialyzable. It is not the toxic alcohols themselves that produce significant toxicity, but their metabolites. Methanol and ethylene glycol are metabolized in a stepwise fashion by alcohol dehydrogenase (ADH) and aldehyde dehydrogenase (ALDH) to the clinically important metabolites: formic acid (methanol) and glycolic, glycoxylic, and oxalic acid (ethylene glycol) (Figs. 151.4 and 151.5). Isopropanol is less clinically consequential, as it is converted by ADH to acetone, which is an end product rather than a substrate of ALDH.
Because ADH preferentially metabolizes ethanol over all other alcohols, no significant metabolism of toxic alcohols will occur while high concentrations of ethanol are present. When ADH is inhibited, the parent compounds are eliminated very slowly without metabolism. In the absence of ADH, ethylene glycol is renally eliminated with a half-life of 8.5 hours while methanol, which is eliminated as a vapor, has a half-life of 30 to 54 hours (76–78).
Clinical Manifestations
The initial physical examination may be unremarkable. All of the toxic alcohols can produce significant CNS depression, and a compensatory tachypnea may be present if there is a metabolic acidosis. Acetone, generated from ADH metabolism of isopropanol, produces nausea, hypotension, hemorrhagic gastritis, and tachycardia, but these are not usually life threatening (79). Formate, a methanol metabolite, can produce blindness from toxicity to the retina and optic nerve (80). Ethylene glycol may cause nephrotoxicity in cases where oxalic acid, the primary metabolite, precipitates as calcium oxalate crystals in the renal tubules (81).

FIGURE 151.4 Metabolism of methanol. (Adapted from Wiener SW. Toxic alcohols. In: Flomenbaum NE, Goldfrank LR, Hoffman RS, et al., eds. Goldfrank’s Toxicologic Emergencies. 8th ed. New York: McGraw-Hill; 2006:1447–1459.)
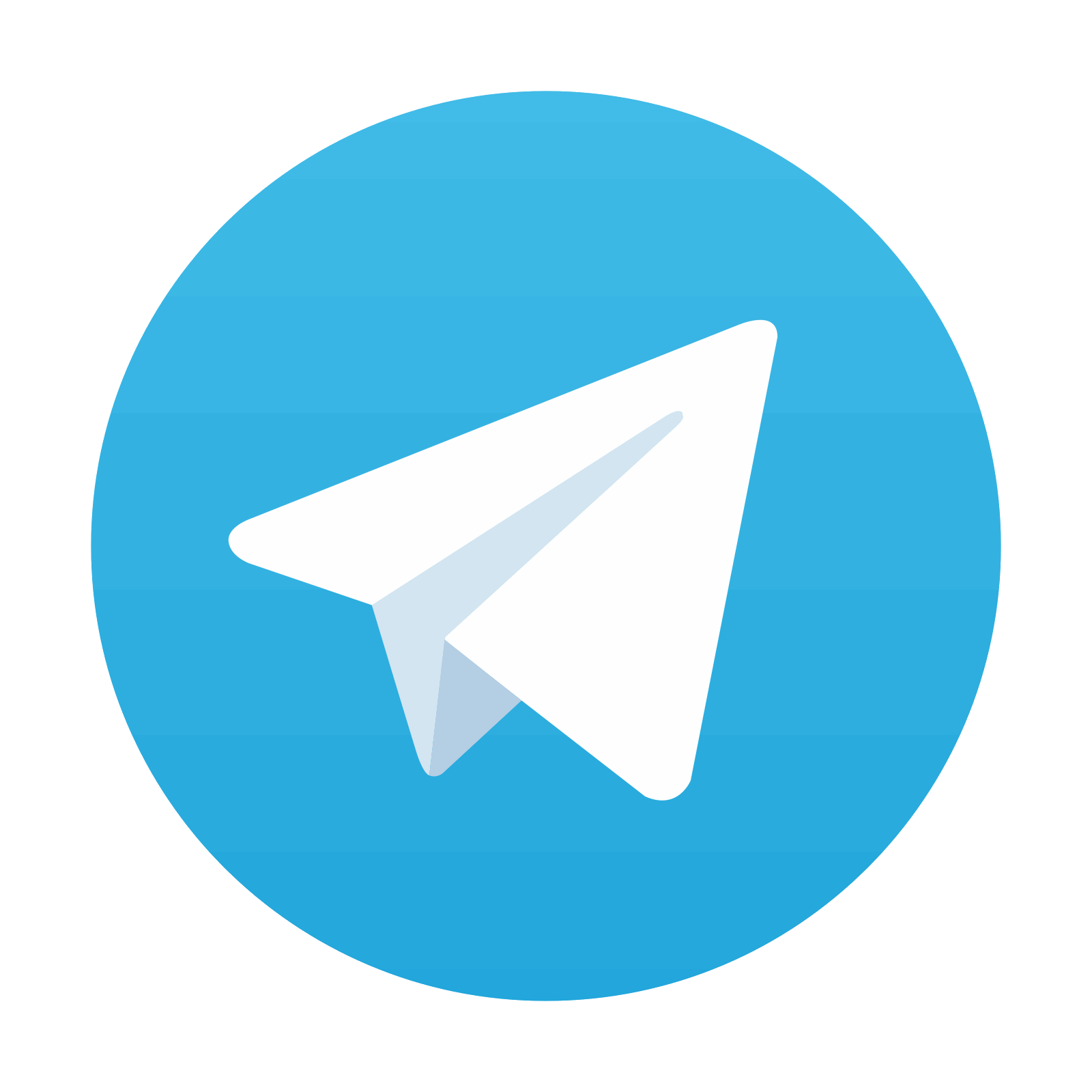
Stay updated, free articles. Join our Telegram channel

Full access? Get Clinical Tree
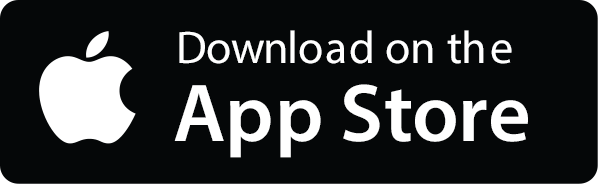
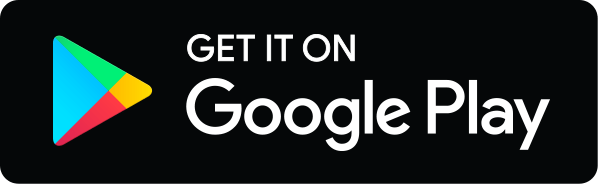