7 Total Intravenous Anesthesia and Target-Controlled Infusion
Total Intravenous Anesthesia
Indications
The most common indications for total intravenous anesthesia (TIVA) techniques in children are the following: those at risk for malignant hyperthermia (in whom volatile agents are to be avoided); children with a high risk of postoperative nausea and vomiting; brief radiologic or painful procedures, when rapid recovery is needed (e.g., magnetic resonance imaging, bone marrow aspiration, gastrointestinal endoscopy); frequent repeated anesthesia (e.g., radiation therapy); major surgery to control the stress response; neurosurgical procedures to assist with control of intracranial pressure and for cerebral metabolic protection, spinal instrumentation, and when evoked motor and auditory brain potentials are needed; and children in need of airway procedures (e.g., bronchoscopy).1–4 Total intravenous sedation and anesthesia are used in pediatric intensive care, but propofol is specifically contraindicated for prolonged use with high infusion rates in very sick or young children, because of the propofol infusion syndrome risk.5–14
Drugs and Techniques
The most commonly used medications include propofol, remifentanil, alfentanil, and sufentanil; ketamine is occasionally used.15 Delivery can be by a manual infusion scheme (Table 7-1) or by using pharmacokinetic model–driven infusion devices with software developed specifically for use in children. Unfortunately, the commercially available software packages usually limit the applicable age from 1 to 3 years or older, or weight from 10 to 15 kg or greater, and the pharmacokinetic (PK) parameters are derived from studies of a relatively few healthy children. Propofol programs that allow for age-, weight-, and gender-related changes in central compartment volume, clearance, and distribution have been developed and perform well in healthy children.16,17 However, there are considerable gaps in knowledge for some drugs, for ill children, and for young children, infants, and neonates, so caution is needed when applying such programs to these populations. Therefore, the anesthesiologist can use these preprogrammed devices as a basis for initiating a TIVA technique but must also use skill, knowledge, and experience to titrate the intravenous (IV) agents to avoid awareness, pain, and adverse effects.
Principles
Healthy children often need a relatively high dose of IV agent per unit of body weight, and maintenance infusion rates need to be higher than the weight-corrected dose for adults.18 Conversely, the immature neonate, the critically ill child, or those with major organ failure need considerably smaller doses of IV anesthetic agents; care is particularly needed in children receiving vasoactive medication and those with congenital heart disease.19 Titration is advisable to allow for wide interindividual variation in PK and pharmacodynamics (PD). Electronic monitoring of depth of anesthesia (processed electroencephalography) has not been sufficiently well-validated in infants and children, and is yet to be used as the sole guide to dosing. After IV injection, it is assumed that the drug rapidly and evenly distributes throughout the body. However, the drug is delivered into the venous side of the systemic circulation, passes through the pulmonary circulation, and then distributes to the major organs and other tissues, all of which have variable blood flow, metabolic enzyme activity, and composition (in terms of proportions of fat and water). Because regional blood flow, body composition, and body proportions vary during development, the PK and PD of drugs are even more complex in children than in adults.
For a fixed infusion rate in a single compartment model, it takes five half-lives to reach a steady-state concentration (greater than 96% of the target) in the blood (Fig. 7-1). To more rapidly achieve steady-state conditions, a bolus dose or loading infusion may be administered. This rapidly fills the Vd, after which a new rate of infusion is calculated to maintain the blood concentration (Fig. 7-2). This is the dilemma of manual infusions; this loading dose has to pass the central volume and is usually so large that it will cause adverse effects, hemodynamic instability, and/or toxicity. Even a remifentanil bolus should be administered over several minutes to reduce the risk of bradycardia, hypotension, and/or difficult mask ventilation.
To more clearly understand the disposition of drugs after an IV dose, it is useful to consider a three-compartment model. The drug is delivered and eliminated from a central compartment, V1 (which includes the blood), but also distributes to and redistributes from two peripheral compartments, one representing well-perfused organs and tissues (fast compartment, V2), and the other representing more poorly perfused tissues, such as fat (slow compartment, V3) (Table 7-2). The transfer of the drug between the central compartment (V1) and the two peripheral compartments (V2, V3), and also the elimination of the drug from the central compartment, is described by a series of clearances indicating the distribution back and forth between paired compartments, such as V1 to V2 and then V2 back to V1. The primary target organ that IV anesthetic agents affect is the brain. Therefore, an additional rate constant (keo) is added to describe the equilibration between the central compartment and the effect site in the brain. This compartment is not represented by a volume but rather by the time required to equilibrate. Consequently, there is a time lag before changes in the blood concentration are reflected in the effect site (see Figs. 7-1 and 7-2).
TABLE 7-2 Nomenclature for TCI Systems
Term | Meaning | Units |
---|---|---|
TCI | Target-controlled infusion | |
Vc or V1 | Central compartment volume | L |
V2 | Fast compartment volume (vessel rich group) = V1 × k12/k21 | L |
V3 | Slow compartment volume (vessel poor group) = V3 × k13/k31 | L |
CL1 | Elimination clearance = V1 × k10 | L/hr |
CL2 or Q2 | Clearance between V1 and V2 = V2 × k21 | L/hr |
CL3 or Q3 | Clearance between V1 and V3 = V3 × k31 | L/hr |
Cp | Blood concentration | |
Ce | Effect-site concentration | |
T | Target concentration | |
CALC | Concentration calculated by TCI software | |
MEAS | Concentration measured | |
k10 | Elimination rate constant | /min |
keo | Rate constant for equilibration between blood and effect-site | /min |
T1/2keo | Half-time for equilibration between blood and effect-site![]() | min |
k12, k21 | Rate constants for movement between V1 and V2 | /min |
k13, k31 | Rate constants for movement between V1 and V3 | /min |
A hydraulic model is useful for understanding these concepts. The central compartment is connected to the peripheral compartments and effect site by a series of pipes of different diameters, and also to a drainage pipe to represent elimination (Fig. 7-3, A through F). The heights of the columns of fluid, which represent concentrations of drug, illustrate the gradient down which drug travels between the central and peripheral compartments, and this can be animated over time to show filling and emptying of compartments relative to each other. The diameter of the interconnecting pipes between the central and peripheral compartments represents the intercompartment clearances, and size of the drainage channel represents elimination. This hydraulic analogy is used in the Tivatrainer PK simulation program.20
Fixed Infusion Rate and a Three-Compartment Model
When a fixed infusion rate is started (see Fig. 7-1), the blood concentration will increase but, almost simultaneously, distribution of drug to the fast compartment and elimination both begin. Distribution of drugs throughout the body contributes more to the removal of drug from blood than elimination for most medications. For remifentanil, extremely rapid esterase clearance dominates. As the concentrations within each compartment equilibrate, the concentration gradient between compartments lessens (slowing drug transfer between compartments) but distribution to the slow compartment continues along with elimination. The net effect is that the blood concentration continues to increase, albeit at a slower rate. As the blood concentration increases toward equilibrium, elimination becomes relatively more important; Figure 7-1 illustrates how far behind the effect-site concentration lags. Eventually, after several hours (or in some cases, days), a steady state is reached where infusion rate is directly proportional to clearance.
Bolus and Variable Rate Infusion in a Three-Compartment Model
A loading dose can start to fill the central compartment and ideally should be aiming to create an effect-site concentration at a specific target concentration without overshoot. Then the rate of infusion should decrease in a stepwise manner to maintain a constant effect-site concentration until a steady state is reached. As drug is delivered into the central compartment, it continuously distributes to the peripheral compartments while it is continuously eliminated. The infusion rate must vary because it has to match the changes in the contribution of distribution and elimination with time (Figs. 7-4 and 7-5). When the infusion is stopped, then elimination will continue to drain the central compartment, and drug will continue to distribute to V2 and V3 along concentration gradients from V1 for some time. Equilibrium may be reached, but the drug now begins to move back from the peripheral compartments into the central compartment, maintaining the central compartment drug concentration for a period of time. This can continue for a protracted interval, particularly for highly lipid-soluble drugs that have a very large slow compartment V3 and a reservoir or depot effect (see Fig. 7-3, F). Eventually the central compartment concentration will decrease. For most anesthetics, the longer the duration of an infusion, the more the drug has distributed into the peripheral compartments, and the larger the reservoir of drug to be redistributed back into the central compartment and eliminated, once the infusion ceases. The half-time of the decrease in drug concentration in blood, therefore, is related to the duration of the infusion for most drugs (except remifentanil [see later discussion]). This is termed the context-sensitive half-time (CSHT), where the context is the duration of the infusion and relates to a pseudo–steady-state maintained by a target-controlled infusion (see later discussion). For an individual drug in an individual patient, CSHT can be plotted against the duration of the infusion (Fig. 7-6, A and B). The CSHT graph will eventually become parallel to the time axis and this is when the pseudo–steady-state becomes a true steady-state. At that time, the infusion has become context insensitive
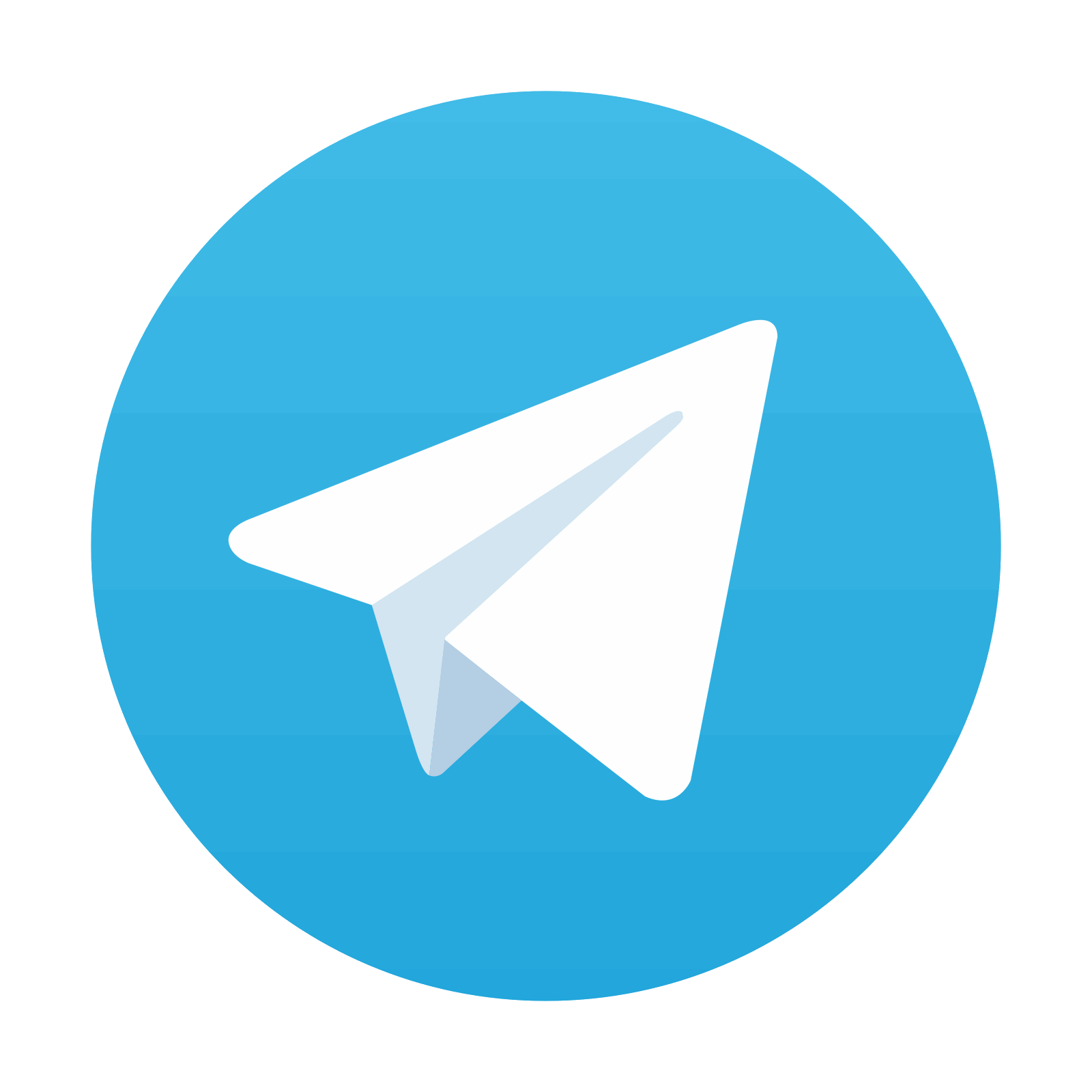
Stay updated, free articles. Join our Telegram channel

Full access? Get Clinical Tree
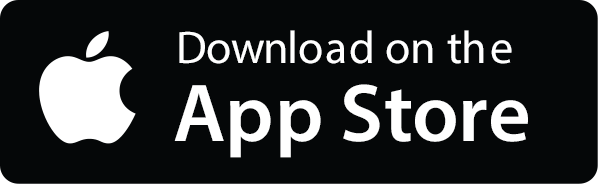
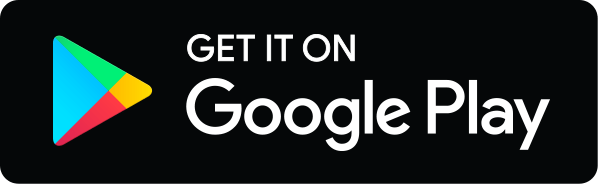