Thyroid Disease
Ori Eyal
Susan R. Rose
KEY POINTS




NORMAL PHYSIOLOGY

![]() FIGURE 109.1. Basic elements in the regulation of thyroid function. TRH stimulates TSH synthesis and release. TRH synthesis is regulated directly by thyroid hormones. TSH stimulates the thyroid gland to synthesize and secret thyroid hormones. T4 is the predominant secretory product of the thyroid gland, with peripheral deiodination of tetraiodothyronine (T4) to triiodothyronine (T3) in the liver and the kidney, supplying 80% of the circulating T3. Both circulating T3 and T4 inhibit TSH synthesis and release independently; T4 via its rapid conversion to T3. SRIH, somatostatin. (Adapted from www.thyroidmanager.org, permission from Endocrine Education Inc.) |
The substrate for the synthesis of thyroid hormone is circulating iodide. The thyroid gland is able to trap iodide from the circulation by an energy-requiring mechanism. The trapped iodide is oxidized by the enzyme peroxidase. The next step in the synthesis of triiodothyronine (T3) and tetraiodothyronine (T4) is iodination of tyrosine to monoiodotyrosine (MIT) and to diiodotyrosine (DIT). Coupling of two DIT molecules results in the formation of T4. Some T3 is also formed within the thyroid gland by condensation of one molecule of MIT and DIT. The formed T3 and T4 are stored in the thyroid gland in combination with thyroglobulin. Release of T3 and T4 from thyroglobulin in the colloid (fluid that contains thyroglobulin in the lumens of the follicles) occurs by proteolysis and is regulated by TSH (2).
Under normal conditions, 10%-20% of T3 and 100% of T4 in the serum are directly secreted by the thyroid gland. The remaining 80%-90% of T3 is derived from peripheral monodeiodination of T4 by the enzyme 5′-monodeiodinase. Thyroxine (T4) may also be metabolized peripherally by the enzyme 5′-monodeiodinase to reverse T3 (rT3), which is largely metabolically inactive. Three types of monodeiodinase have been described: Type I 5′-monodeiodinase predominantly is expressed in the liver and the kidney and accounts for most circulating T3. Type II 5′-monodeiodinase is located predominantly in the brain, pituitary, and brown adipose tissue, and acts primarily to increase the intracellular levels of T3 in these tissues. Circulating T3 is not active in the brain, and T4 is not active in the brain without this enzyme. Type III 5′-monodeiodinase catalyzes the conversion of T4 to rT3 and of T3 to T2 (diiodothyronine).
In the blood, thyroid hormones are mainly associated with carrier proteins: thyroxine-binding globulin (TBG), prealbumin or transthyretin, and albumin. The prolonged half-life of thyroid hormone is related to its protein binding. The bound form is in equilibrium with free hormones. The most important carrier protein for T4 is TBG. TBG and albumin seem equally important as carrier proteins for T3. The concentrations of free T4 and free T3 approximate 0.03% and 0.30%, respectively, of the total serum thyroid hormone concentration (3). The bound hormone acts merely as a serum reservoir. It is the free hormone that is available to the tissues for intracellular transport and feedback regulation. The free hormones penetrate the cell membrane by carrier-mediated, energy-dependent transport. The carrier-transport system for T3 and T4 is saturable and stereospecific and requires ATP. The two iodothyronines typically do not compete for uptake (4). T4 is monodeiodinated to T3 in the cell cytoplasm.
The cellular cytoplasmic T3 diffuses into the nucleus and initiates its actions by binding to one of two groups of nuclear DNA-bound thyroid hormone receptors (TR), TRα or TRβ. After binding, the T3/TR complex either homodimerizes with a second TR or heterodimerizes to a retinoid x receptor. These complexes directly bind to consensus DNA sequences, resulting in either activation or inhibition of gene transcription (Fig. 109.2). The thyroid hormone nuclear receptors are members of the steroid hormone/retinoic acid receptor superfamily and function as DNA transcription factors. The gene for TRα is located on chromosome 17, and the gene for TRβ is on chromosome 3. Each gene is translated to several mRNA species, including TRα1, TRα2, TRβ1, and TRβ2. TRα1 and TRβ1 are the major nuclear receptors present in most tissues. TRα2 is present in developing brain tissue. TRβ2 is present in pituitary and selected brain tissues (3). T3 is the active hormone and has a 15-fold higher binding affinity for the DNA receptor than does T4.
A number of assays have been developed for TSH and free T4 (FT4). One should be aware of the specific assays being used at one’s own institution, the normal ranges in these assays, and the possible factors that may interfere with each assay and cause falsely elevated or falsely decreased results. The ultrasensitive TSH immunoassay uses monoclonal antibodies. With the availability of third- and fourth-generation ultrasensitive assays, TSH can now be measured quickly and with more accuracy than with previous assays. Estimates of the FT4 concentration in serum can be generated by direct and indirect assay. Analog indirect assays for FT4 employ radioimmunoassay. The gold standard for measurement of FT4 uses equilibrium dialysis, which is the most accurate and direct measurement of the concentrations of FT4. FT4 can be measured indirectly by using radiolabeled T4; the proportion that is unbound by protein is determined by dialysis, and the concentration of FT4 can then be calculated as the product of the total hormone concentration and the fraction that is free (4).
![]() FIGURE 109.2. Thyroid hormones exhibit their action through binding to nuclear receptors. T3 and T4 (the major circulating thyroid hormones) are transported across plasma membranes into peripheral cells, where T4 is monodeiodinated to T3. The cytoplasmic T3 diffuses into the nucleus, where it binds to the thyroid hormone receptors, which in turn, bind to DNA at retinoid x receptor (RXR). The hormone activated receptor binds to thyroid hormone response elements (TRE) and regulates gene transcription and, thus, levels of mRNA. (Adapted from www.thyroidmanager.org, permission from Endocrine Education Inc.) |

A decrease in serum T3 and an increase in rT3 levels are characteristic of the fasting state. These are also the most common changes in nonthyroidal illness (NTI) in response to a variety of acute and chronic illnesses, a condition that is referred to as the euthyroid sick syndrome, NTI, or the low-T3 syndrome. The most rapid and consistent findings in NTI are decline in circulating total T3 and free T3 and an increase in the inactivated rT3 concentrations. A major contributing factor to the decrease in the peripheral production of T3 is the decreased conversion of T4 into T3 by type I enzyme 5′-monodeiodinase. The concomitant rise of rT3 appears to be due to impaired rT3 clearance secondary to decreased 5′-monodeiodinase activity during illness, and not so much due to an increase in rT3 production by type III monodeiodinase. The greater the severity of the disease, the greater the decline in serum T3 levels (5,6), greater the rise in rT3, and the greater the reduction in the T3/rT3 ratio (7).
The majority of patients with NTI have a normal or slightly decreased serum FT4 level. Most commonly, serum total and free T3 concentrations are low, whereas serum T4 concentration remains within the normal range. However, with increasing severity of illness, the serum T4 level and that of T3 decrease. In severely ill patients, an additional suppression of the hypothalamic-pituitary hormone release is often observed (6). The concentration of TSH typically remains within the low-to-normal range, but the circadian variation of TSH may be lost, and response of TSH to TRH is blunted. Low TSH level during critical illness is associated with poorer overall prognosis. Various agents, such as dopamine and corticosteroids, may further decrease TSH levels (Table 109.1). Patients with prolonged critical illness show diminished TSH pulsatility, as if progressive dysfunction of the hypothalamus is involved, characterized by an absent nocturnal TSH surge and decreased TSH pulse amplitude. The changes in nocturnal TSH secretion in NTI resemble those found in central hypothyroidism, suggesting that hypothalamic changes are involved in this condition. In severe prolonged illness, the changes in thyroid function may be accompanied by a decline in secretion of growth hormone, gonadotropins, and adrenocorticotropic hormone (ACTH) (6,7,8). During the recovery phase, the TSH level may rise slightly above the normal range. Whether these alterations serve as physiologic adaptation or contribute to further deterioration remains an intriguing question (Fig. 109.3). The treatment of NTI is controversial. Multiple studies have addressed this issue in patients with cardiac disease, sepsis, pulmonary disease, burn, and trauma. Brent and Hershman (9) found no difference in mortality between critically ill patients with NTI who were treated with T4 and the control group consisting of critically ill patients with NTI who were not treated with T4. Treatment with T4 delayed the normalization of thyroid function during recovery. Treatment of critically ill patients with T3 has the potential hazardous effect of increasing metabolic rate, catabolism, and energy expenditure. In view of these considerations, critically ill patients with a low T3 and T4 should not be treated with thyroid hormone replacement unless there is also clinical evidence of hypothyroidism (8). A full thyroid function panel is needed to distinguish NTI from hypothyroidism. However even then, the diagnosis may be difficult because free T4 levels are often unreliable in the face of critical illness.
TABLE 109.1 MEDICATIONS ASSOCIATED WITH HYPOTHYROIDISM | ||||||||||||||||
---|---|---|---|---|---|---|---|---|---|---|---|---|---|---|---|---|
|
![]() FIGURE 109.3. Changes in thyroid function during critical illness. In the acute phase of illness, the following are observed: reduction in circulating T3; increase in serum rT3; and no change in serum FT4, total T4, or TSH. In phase 2: modest increase in FT4 and further decrease in T3 and increase in rT3. In phase 3: a loss of pulsatile secretion of TSH; decrease in T4, T3, and FT4; and an increase—followed by a decrease—in serum rT3. In the recovery phase: a gradual normalization of parameters; TSH can be elevated in this stage. (From Brent GA, Hershman JM. Effects of nonthyroidal illness on thyroid function tests. In: Van Middlesworth L, ed. The Thyroid Gland: A Practical Clinical Treatise. Chicago, IL: Year Book Medical, 1986:83-110, with permission.) |
TABLE 109.2 CHANGES IN THYROID FUNCTION TESTS IN HYPOTHYROIDISM AND DURING CRITICAL ILLNESS | ||||||||||||||||||||||||||||||||||||||||||
---|---|---|---|---|---|---|---|---|---|---|---|---|---|---|---|---|---|---|---|---|---|---|---|---|---|---|---|---|---|---|---|---|---|---|---|---|---|---|---|---|---|---|
|
If the downregulation in NTI is an energy-saving neuroendocrine adaptation to disease, attempts to increase and thereby restore thyroid hormone concentrations may be disadvantageous. At the current time, thyroid hormone therapy should not be initiated in the critical care setting in the absence of clear clinical and laboratory evidence for hypothyroidism (10
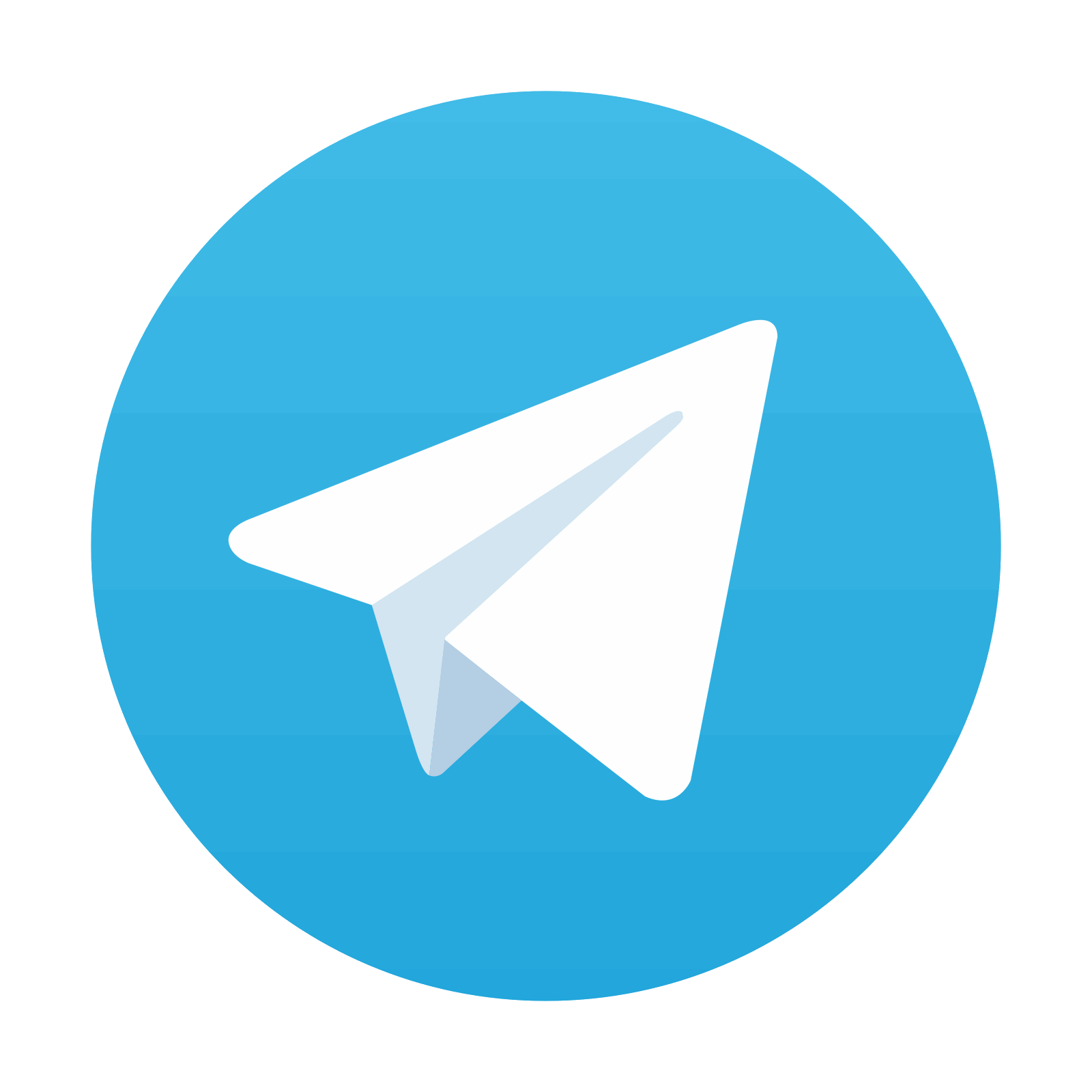
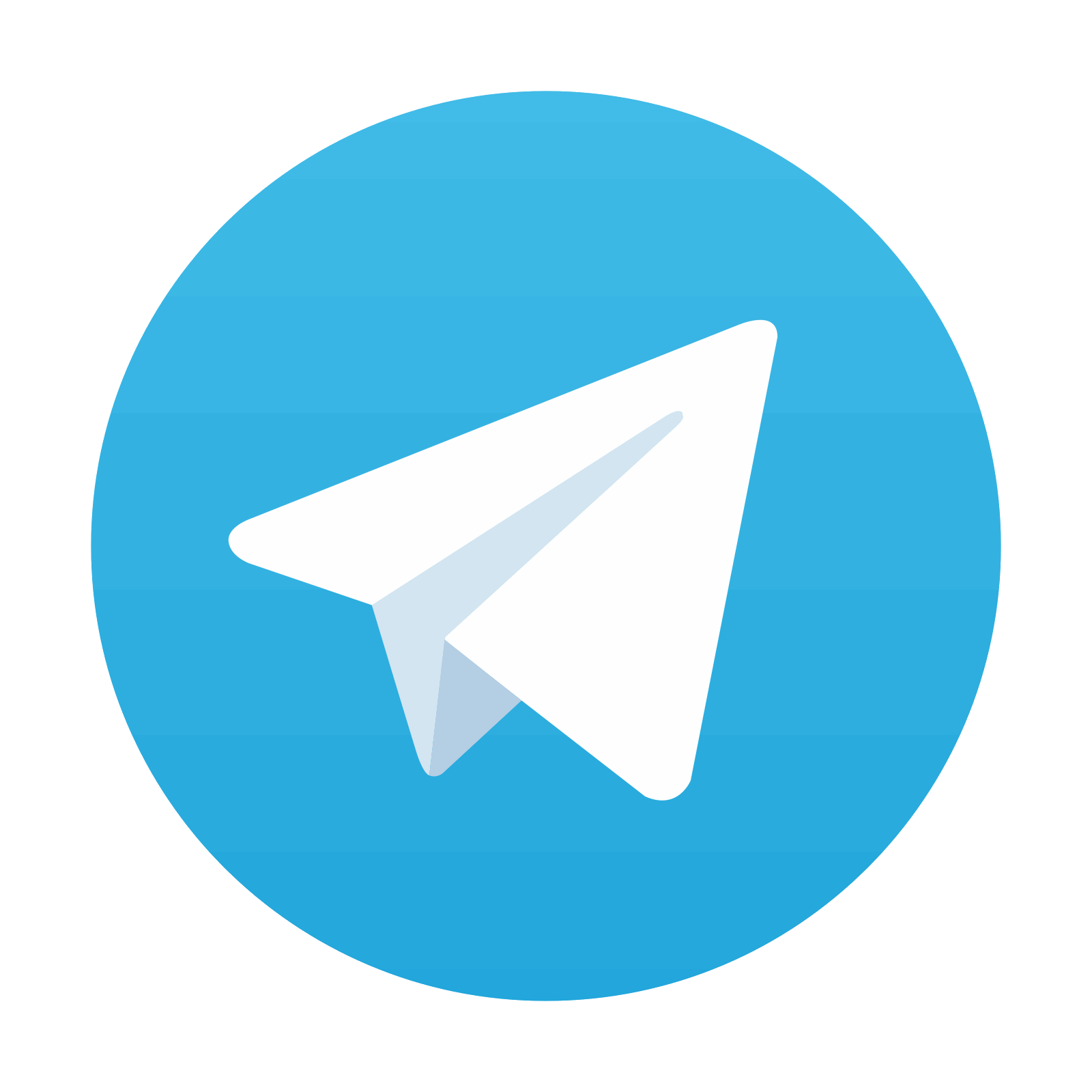
Stay updated, free articles. Join our Telegram channel

Full access? Get Clinical Tree
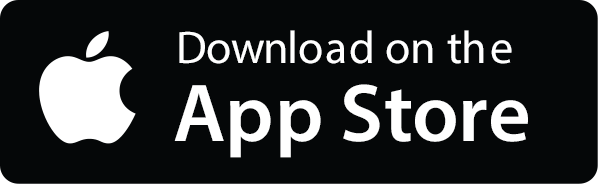
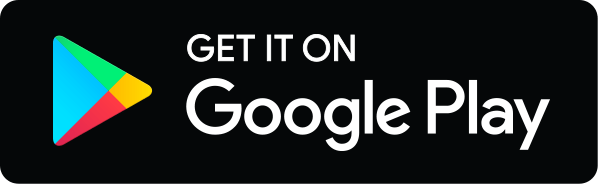